相干测风激光雷达研究进展和应用
下载: 2795次封面文章
1 引言
对流层风场、大气退偏比等参数是风能源开发供应、航空安全、大型建筑物和重大工程安全设计、城市规划和防灾管理等的重要参数,也是大气污染物稀释、扩散、输送的重要参数。目前,使用基于米氏散射原理的相干多普勒测风激光雷达,可以实现从地面到对流层无盲区的大气参数观测,并且具有高精度、高分辨率、大探测范围等优点,成为国际研究的重点。
目前,美国航空航天局(NASA)、美国国家海洋和大气管理局(NOAA)、美国洛克希德马丁公司(LMCT)、美国雷神公司(Raytheon)、日本三菱公司(Mitsubishi Electric)、法国Leosphere公司等相继开展了理论和实验研究,并进行了商业化样机的研制。国内相干激光雷达主要研究单位包括中国科学技术大学(USTC)、中国电子科技集团公司第二十七研究所(CETC27)、中国科学院上海光学精密机械研究所(SIOM)、哈尔滨工业大学(HIT)、中国海洋大学(OUC)、北京理工大学(BIT)等。本文简要回顾了相干激光雷达的发展历史,介绍了不同波段相干激光雷达研究现状及其应用。
2 相干激光雷达研究现状
全光纤相干多普勒测风激光雷达系统可以分为激光光源模块、发射接收模块及后期的信号处理模块。如
相干测风激光雷达具有如下优点:
1) 相干探测采取拍频的方式将后向散射信号放大,理论上信噪比可达到量子噪声极限;
2) 相干激光雷达要求本振光及信号光的波前匹配,因此相干激光雷达对背景噪声、探测器噪声具有抑制作用,可实现在无滤波器条件下的连续观测;
3) 相干测风激光雷达无需光学鉴频器,接收光路简单,对温度梯度、应力梯度不敏感;
4) 随着激光波长的增加,单光子能量
相对于CO2激光器和闪光灯抽运固体激光器,二极管抽运的固体激光器在发光效率、激光器尺寸大小和使用寿命等方面都有巨大提升。相对于CO2激光器,固态激光器的波长更短。为了实现更好的相干混频效率,短波长对光学器件表面精度的要求更高,对光路精度要求也更高,短波长对大气折射率变化和湍流更敏感,但是短波长具有很多优势。根据径向风速
表 1. 多普勒测风激光雷达研究状况
Table 1. Research status of Doppler wind lidar
|
2.1 相干激光雷达波长选取考虑因素
1) 大气透过率。
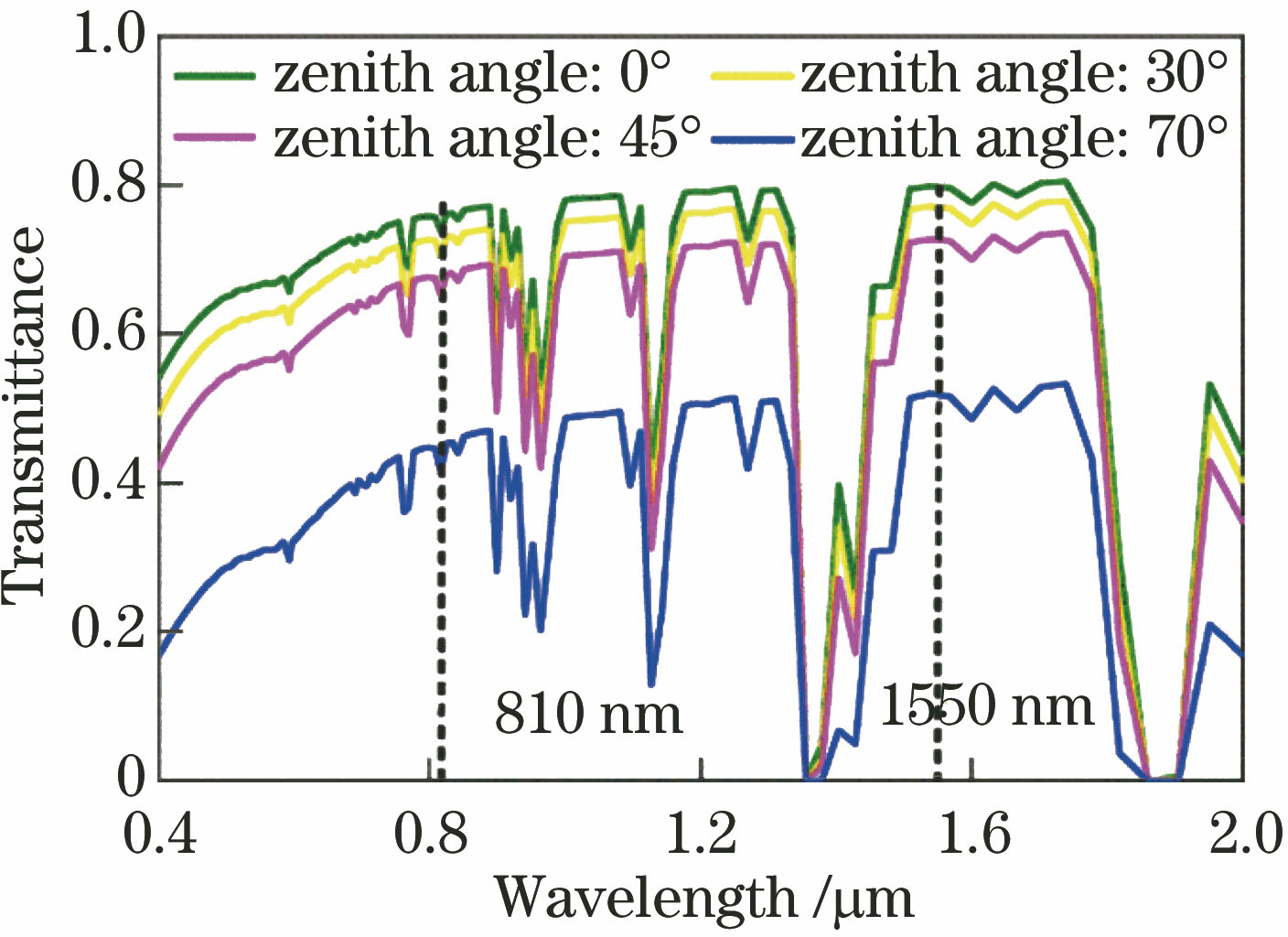
图 2. 不同天顶角下可见光到近红外波段的大气透过率
Fig. 2. Transmittance of the atmosphere from visible light to near infrared bands at different zenith angles
2) 人眼安全,激光增益介质。
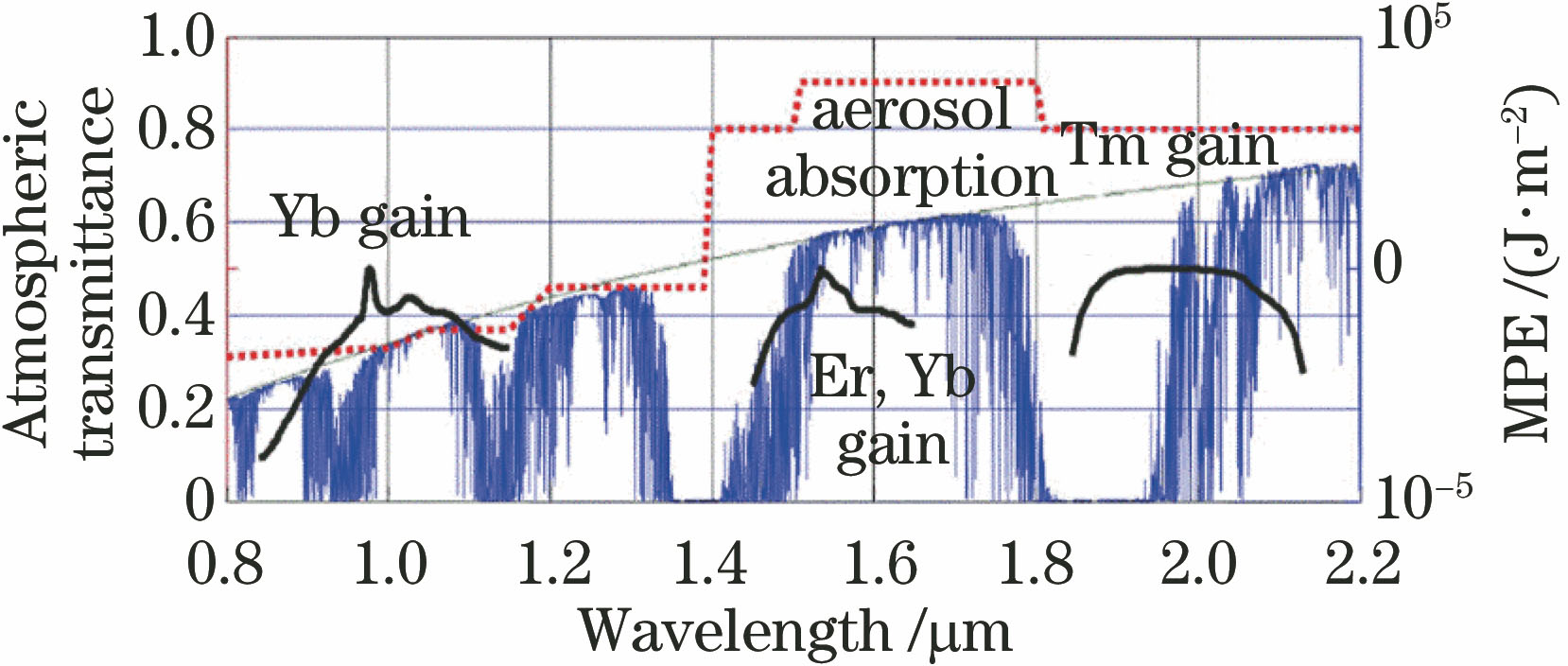
图 3. 近红外波段激光增益介质和人眼最大曝光率示意图
Fig. 3. Laser gain medium and human eye maximum exposure rate in the near infrared band
3) 光纤损耗。激光在光纤中的衰减主要由光纤本身材料不均匀、瑞利散射及光纤中的杂质散射和吸收等因素造成。
4) 天空背景辐射。由于天空背景的影响,雷达白天工作的性能变差,假设太阳为5900 K的理想黑体,其光谱辐射如
综上,相干激光雷达波长主要集中在1.5 μm和2.0 μm,同时1.5 μm波段为通信波段,各种光器件比较成熟,使得1.5 μm成为目前的主流波段。接下来分别介绍不同波段的相干激光雷达的发展。
2.2 气体相干测风激光雷达
CO2激光器具有高能量转化效率、稳定的单频率激光输出、高能量的脉冲和连续波输出、大气透射窗口好、人眼安全的激光波长等特点,被相干雷达系统大规模使用[9]。
第一台基于连续波(CW)CO2激光器的相干多普勒测风激光雷达由NASA的Huffaker研制成功[1],该雷达使用焦距可调的变焦光学系统实现了不同的空间分辨率;大气后向散射信号被接收望远镜耦合进CO2激光器的谐振腔进行放大,并选择与本振光匹配的激光模式,与本振光进行拍频。系统的本振光和出射激光都没有经过移频,所以该套系统是“自差”或“零差”系统。由于激光器谐振腔增益带宽受限,该系统在风速较大时多普勒频移较大,导致后向散射信号超出谐振腔增益带宽,无法工作。1968年,Raytheon和 NASA合作对该系统进行升级,解决了大风速情况下系统失能的问题,并用于实现几百米高空情况下由飞机产生的涡流[10-11]和大气边界层内风场的探测[12]。英格兰的Vaughan课题组使用基于CW CO2相干多普勒测风激光雷达系统实现了对气溶胶后向散射的探测[13-14]。
1970年代,美国雷神公司成功研制出脉冲式CO2相干多普勒测风激光雷达,并将其用于商业航班路线上晴空湍流的探测,该系统激光脉宽为1 μs,脉冲重复频率为200 Hz[15],随后,在NASA Convair990上进行了实验[16]。1984年,NOAA基于10.6 μm的CO2激光雷达,对大气风场进行了测量,并和风速计、气球及微波雷达的测量结果进行了比较,其结果和风速计比较,标准差为0.34 m/s[17]。
1980年代,为了进一步缩小激光器体积并增加激光器的功率,横向激励气体(TEA)激光器开始被使用,基于TEA技术,激光单脉冲能量可达到100 mJ。1980年代中期,美国的Hardensty课题组及其他机载测风项目的课题组,开始使用单脉冲能量1 J,脉冲重复频率20 Hz的脉冲式CO2激光器[18-22]作为相干多普勒测风激光雷达的光源。
美国大气研究中心(NCAR)的Mayor等[23]和加州理工大学的Kavaya等[24]使用波长为9.25 μm和10.6 μm的TEA CO2相干多普勒测风激光雷达开展了大气折射率结构常数、湍流和大气后向散射参数测量的相关工作。法国国家科学院(CNRS)的Flamant和德国航空太空中心(DLR)的Werner课题组合作进行了基于TEA CO2激光器的机载脉冲式相干多普勒测风激光雷达的研究[25]。
CO2激光技术的问世,在一定程度上提高了相干激光雷达探测能力,但CO2激光器能耗高、体积大、工作环境要求低温等缺点限制了其发展。
2.3 1.06 μm相干激光雷达
1985年,斯坦福大学的Kane课题组研发了基于Nd∶YAG激光器的1.06 μm波长相干多普勒测风激光雷达[26],该系统使用闪光灯抽运激光器将激光脉冲功率放大至2.3 kW,实现了600 m风场和2.7 km的云层探测。1988年,Kavaya等[27]研发出了1.06 μm波长的相干多普勒测风激光雷达系统,该雷达在激光脉冲宽度为1 μs时能量为8 mJ;脉冲宽度为0.5 μs时能量为5 mJ,重复频率为10 Hz,该雷达实现了3.75 km的水平风场探测距离。随后,在美国相干技术公司(CTI)、NASA马歇尔太空飞行中心(MSFC)和NASA兰利研究中心(LaRC)的共同合作下,该系统的脉冲能量升级为1 J,脉冲重复频率为10 Hz,在肯尼迪宇航中心(KSC),为发现者号航天飞机发射和着陆过程提供气象保障,实现了地表到26 km高度的风场探测[28]。考虑到人眼安全, 1.06 μm逐渐被1.5 μm和2.0 μm波长取代。
2.4 1.5 μm相干激光雷达研究现状
2000年以后,得益于光纤通信技术的发展,结构更为紧凑、发光效率更高、成本更低的1.5 μm波长的相干多普勒测风激光雷达系统成为研究的新热点。
从第一台基于CO2激光器的相干多普勒测风激光雷达问世以来,LMCT和CTI就一直在致力于相干多普勒测风激光雷达的研究。2002年,LMCT发布了基于2.0 μm的WindTracer商用相干多普勒测风激光雷达系统。目前WindTracer已经升级为基于1.617 μm的Er∶YAG激光器。NASA使用商用WindTracer系统进行了飞机风切变、晴空湍流等探测,并于2009年在丹佛国际机场对飞机涡流进行了建模和预测[29]。
由于3 mJ的单脉冲能量导致WindTracer对激光器的性能、光学器件的品质等要求都很高,造成器件的寿命都很短且极易发生损坏,因此系统的适用性差[30-31]。为此,NASA研发了基于Er∶Glass光纤激光器的全光纤Windimager相干多普勒测风激光雷达系统[31]。其系统装置及风速扫描结果如
与常用的相干多普勒测风激光雷达系统相比,该系统的创新点在于:1) 在AOM之前加入了一个光开关,用于抑制AOM的直流噪声泄露;2) 激光模块参数可调,可以实现近距离低脉冲能量、高脉冲重复频率、高距离分辨率的探测,或者实现高脉冲能量、低脉冲重复频率、低距离分辨率的远距离风场探测;3) 将2个相距1 m的4 pixel高灵敏度InGaAs探测器,放置在雷达望远镜前40 m处,用于调节望远镜的重叠因子。外场实验证明,在脉冲能量为240 μJ,脉冲宽度为400 ns,脉冲重复频率为4 kHz,累计0.25 s情况下,当PM2.5含量(质量浓度,下同)为10 μg·m-3时,水平最远探测距离为9.5 km;当PM2.5含量为17 μg·m-3时,垂直最远探测距离为3 km。

图 6. NASA相干激光雷达装置图及风速测量结果图。(a)相干激光雷达装置图;(b)风速测量结果
Fig. 6. NASA coherent lidar installation diagram and wind velocity measurement results. (a) Lidar prototype; (b) wind speed measurement results
2010年8月,NCAR的Spuler等[32]基于连续波相干多普勒测风激光雷达,在12 km高空进行了飞机前方湍流探测的机载实验。该系统使用的是波长为1560 nm的分布反馈式(DFB)激光器,5 W的单模光纤放大器,带放大器模块的InGaAs二极管探测器,采集卡采样频率为200 MHz。飞机机翼吊舱处安装直径为50 mm的望远镜,望远镜聚焦在飞机前方30 m。对比使用飞机上皮托管与连续波相干多普勒测风激光雷达测得的飞行速度,得到激光雷达测量风速的精度优于0.052 m/s。
2011年,FiberTek公司的Akbulut等[33]进行了湍流和波音747飞机尾流的数值模拟,并使用该公司自己研发的相干多普勒测风激光雷达进行了实际探测。该系统工作波长为1.55 μm,线宽为2.5 kHz,相对强度噪声(RIN)在20 kHz重复频率时小于-140 dBc/Hz,AOM频移55 MHz,使用二级激光放大系统将出射激光脉冲能量放大至120 μJ,脉冲重复频率为25 kHz,脉冲宽度为800 ns。
2016年,FiberTek公司进一步提高了激光雷达的脉冲能量[34]。使用中心波长为1572.3 nm,脉冲能量440 μJ的激光雷达实现了二氧化碳气体探测。
日本三菱电机有限公司(MEC)从20世纪90年代后期开始致力于相干多普勒测风激光雷达的研究工作[35]。
1998年,三菱电机公司的Asaka等[36]使用1.53 μm波长的半导体种子激光器,经Er,Yb∶Glass激光器放大器放大后,实现单脉冲能量为2.5 mJ,脉冲宽度为200 ns,脉冲重复频率为20 Hz的脉冲激光输出,使用直径600 mm的望远镜实现超过800 m的水平探测距离。这是世界上首台基于1.5 μm人眼安全波长的相干多普勒测风激光雷达。
2001年,Yanagisawa等[37]和Asaka等[38]将Er,Yb∶Glass激光器改进为波长为1.54 μm的Er,Yb∶Glass激光器,单脉冲能量为10.9 mJ,脉冲宽度为228 ns,脉冲重复频率为15 Hz,并将该激光器用于望远镜直径为100 mm的相干多普勒测风激光雷达系统,在距离分辨率为30 m的情况下可实现5 km距离的风场探测。
2003年,经过Hiranno的升级,三菱电机公司将1.54 μm的Er,Yb∶Glass相干多普勒测风激光雷达系统的探测距离提升到10 km,并使用该系统进行了一些外场实验[39]。
全光纤系统具有结构紧凑、方便组装和维护、成本低、系统更稳定等诸多优点,因此三菱公司从2002年起开始报道其全光纤相干多普勒测风激光雷达系统的研发成果,完成了机载验证实验,并于2003年推出首台原理样机[40]。2006年,三菱电机公司推出了商用的全光纤相干多普勒测风激光雷达系统LR-05FC[41]。2010年,升级之后的LR-08FS系统被用于香港机场[42]。
2007年,三菱电机通过使用芯径25 μm的大芯径光纤,在LR-08FS机型的光纤激光放大器后方再增加一个光纤激光放大器,使用二级放大系统将单脉冲能量提高至179 μJ,实现大于10 km的水平风场探测。至此,三菱电机公司已将全光纤相干多普勒测风激光雷达系统按照探测距离分为3个系列(1,3,5 mile,其中1 mile=1.609344 km)[43-45]。
2012年,Sakimura等[46]使用Er,Yb∶Glass平面波导技术和二级激光放大技术,对出射激光输出功率进一步放大[47],实现了超过30 km的水平风场探测距离。2014年,三菱电机公司又报道了该系统在机载实验中的结果,如
2008年,法国航空航天中心(ONERA)首次报道了基于1.5 μm光纤激光器的相干多普勒测风激光雷达[49],并使用该系统进行了飞机尾流的探测。在考虑到光纤的受激布里渊现象之后,ONERA自主研发了掺Er,Yb的光纤激光放大器[50]。
2009年,ONERA对第一代相干多普勒测风激光雷达系统进行了升级,使用三级抽运对种子激光进行放大,并使用大模场面积光纤抑制受激布里渊现象,进一步将激光器的脉冲能量提高至120 μJ,实现了飞机尾流的探测[51-52]。

图 7. 三菱电机公司机载雷达风速测量结果。(a)风速结果;(b)垂直加速度的周期性变化;(c)室外温度的周期性变化
Fig. 7. Mitsubishi electric corporation airborne lidar wind velocity measurement results. (a) Results of wind velocity; (b) periodic change in vertical acceleration; (c) periodic change in outside air temperature
2014年,通过在大模场面积的光纤中加入应力等方式,ONERA进一步提高了光纤的受激布里渊阈值[53],在单脉冲能量370 μJ的情况下,实现了超过10 km的风场探测距离。
2015年,ONERA通过使用多个光纤放大器并联,提高了光纤激光器的激光脉冲能量[54],在500 μJ脉冲能量情况下,实现了16 km的风场探测距离[55]。通过与Leosphere公司合作,该激光器已经应用于WindCube产品中[56-57],并进行了灾难天气预测、机场风切变监测等外场实验[58-61]。
法国Leosphere成立于2004年,与ONERA和丹麦科技大学(DTU)都有合作关系[56-57]。其产品分为陆基WindCube系列和风电机舱雷达Windiris系列,广泛应用于风力发电[62]、航空安全保障[63]、天气预报[64]、空气质量监测[65]等。
英国ZephIR公司为英国QinetiQ公司2007年成立的子公司。在20世纪90年代中期,QinetiQ公司就致力于使用光纤激光器替代当时相干多普勒测风激光雷达系统中使用的CO2激光器,并于20世纪90年代后期成功研究了全光纤的连续波相干多普勒测风激光雷达系统[66-67],且在2002年成功研究了全光纤的脉冲式相干多普勒测风激光雷达系统[68-71],同时与DTU合作,将其系统用在风力发电领域[69-70]。

图 8. 不同雷达的风速测量结果。(a)微波雷达;(b) Leosphere公司相干雷达
Fig. 8. Wind velocity measurement results of different radars. (a) Microwave radar; (b) Leosphere coherent lidar
2003年,QinetiQ公司与DTU合作研发了该公司的第一台商用的基于连续波的相干多普勒测风激光雷达,该系统使用变焦式望远镜,实现了不同距离处的风场探测,并使用独特的算法系统,去除了云层对风速探测精度的干扰。该套系统在2003年12月经过外场实验验证后,已经在世界50多个国家和地区销售超过3000套。该公司目前的产品为ZephIR300,可安装在陆基、移动平台或风电机机舱上。
英国SgurrEnergy公司成立于2002年,与DTU合作生产了Galion系列脉冲式相干多普勒测风激光雷达,主要用于海上和陆地风力发电机厂的风场探测、风能储量预测等[72-73]。2013年,DTU根据多年实际使用情况,给出了Galion系列的测试报告[74],认为该系统稳定可靠,其目前的型号为G250和G4000。
英国Halo-Photonics公司从20世纪80年代末期就开始研究基于CO2激光器的相干多普勒测风激光雷达系统,2001年研发了基于光纤器件的1.548 μm脉冲式相干多普勒测风激光雷达系统,并于2002年使用该系统进行大气风场观测[75]。
2004年,Halo-Photonics公司基于掺Er,Yb激光放大器,研发出了波长为1.562 μm,脉冲能量为1.15 mJ的高功率激光器[76]。并于2005年使用该高功率激光器将相干多普勒测风激光雷达的探测距离提升至8 km[77]。并使用该雷达对雷暴[78]、晴空湍流[79]、热带雨林的边界层变化[80]、冰晶云下落速度[81]、湍流耗散率[82]等进行了研究。
DTU风能技术中心主要研究风力发电领域的相关技术,在相干多普勒测风激光雷达领域与ZephIR和Leosphere等公司有大量合作。由于风力发电领域对激光雷达的制造成本要求苛刻,因此DTU更关注于造价相对便宜的连续波相干多普勒测风激光雷达。Abari课题组从理论上设计了具有区分径向风速方向的连续波相干多普勒测风激光雷达[83-85],并从理论上提出了一种可以同时测大气退偏振比和大气风场的相干多普勒测风激光雷达[86]。DTU也致力于使用更便宜的半导体激光器替代光纤激光器,进一步降低相干多普勒测风激光雷达的成本[87-88]。DTU还推出了商用的WindScanner产品,有脉冲式和连续波两种模式,可以实现0~300 m高度的风场探测。
得益于国内光纤器件制造工艺的提高,以及机场飞行安全、环保产业、风力发电等对风场观测需求的加大,国内相干多普勒测风激光雷达的技术水平发展迅猛。
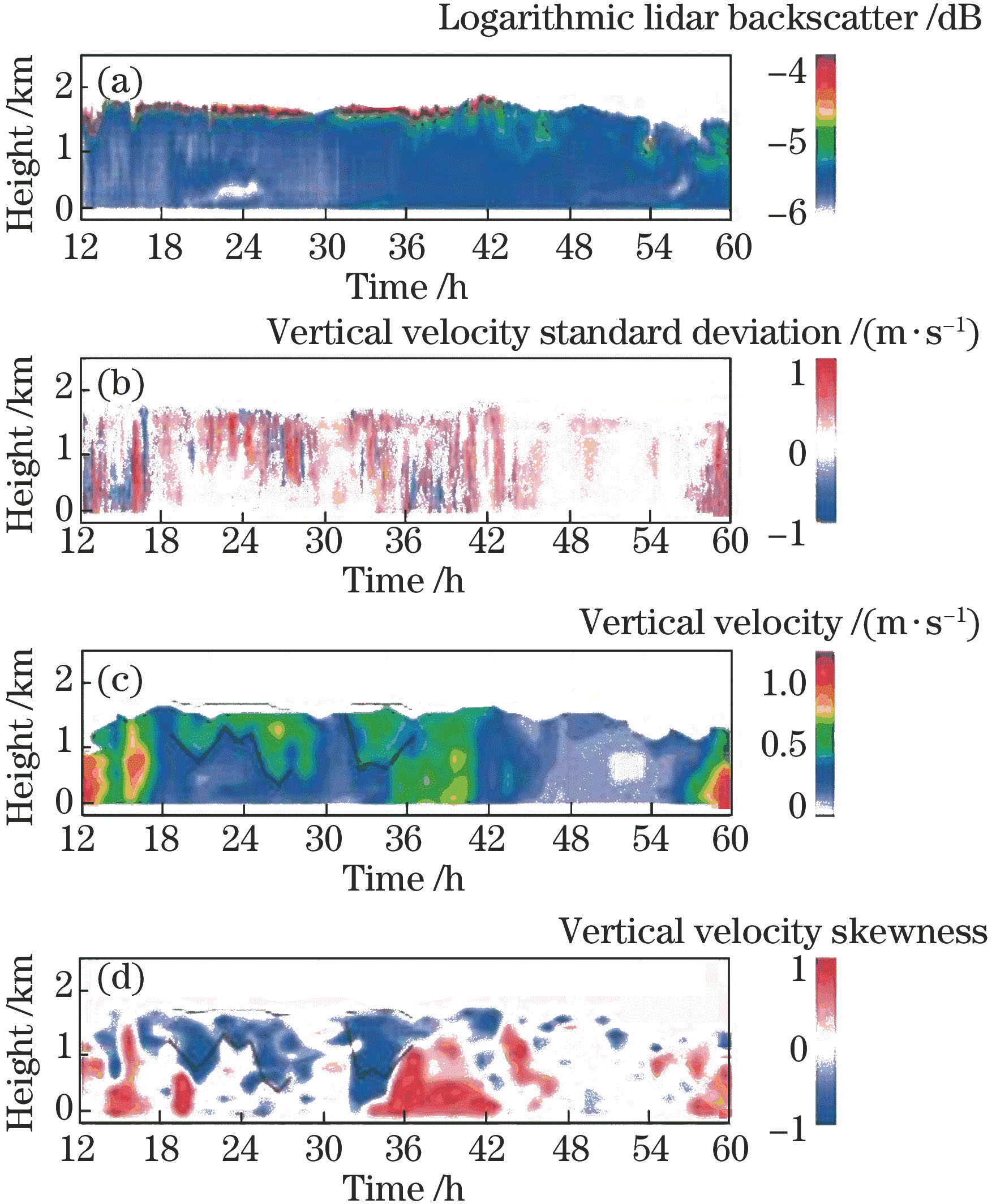
图 9. Halo-Photonics公司机载雷达风速测量结果边界层反演结果。(a)相干激光雷达后向散射系数;(b)垂直风速标准差;(c)垂直风速;(d)垂直速度偏度
Fig. 9. Halo-Photonics airborne lidar wind measurement results and boundary layer inversion results. (a) Coherent lidar backscatter coefficient; (b) vertical velocity standard deviation; (c) vertical velocity; (d) vertical velocity skewness
2010年,中国电子科技集团公司第二十七研究所李冬梅等[89]报道了一套基于1.5 μm的连续波相干多普勒测风激光雷达系统,实现了200 m距离内的风速测量。2013年和2015年,潘静岩等[90]和封治华等[91]又分别报道了全光纤相干多普勒测风激光雷达系统与测风塔和探空气球的对比实验,在800 m的探测距离内,均取得了良好结果。
表 2. 1.5 μm国外相干激光雷达研究单位及指标
Table 2. Research institutes and parameters of 1.5 μm coherent lidar abroad
|
Note: QinetiQ data are from ZephIR 300 (https://www.zxlidars.com/wind-lidars/zx-300/); Leosphere data are from WindCube (https://www.leosphere.com/); Halo-Photonics data are from Stream Line XR (http://halo-photonics.com/StreamLine-XR-Doppler_Li) 2011年,西南技术物理所(SITP)(209所)冯力天等[92]报道了1.55 μm全光纤相干多普勒测风激光雷达系统,实现了5~200 m高度范围内的风场测量,并与实验基地内测风塔数据进行了对比。
中国科学院上海精密光学机械研究所Liu等[93]和Diao等[94]2012年报道了基于全光纤1.539 μm的相干多普勒测风激光雷达,并于2014年对系统进行了升级,实现了水平3 km、垂直方向1.9 km的探测距离。2015年,刁伟峰等[95]采用非线性最小二乘法对激光雷达测量的风速剖面矢量进行反演,对比了激光雷达与风廓线雷达测量的风速,两者测量的水平风速、风向和竖直风速的相关系数分别为 0.988,0.941,0.966。
北京理工大学张寅超课题组一直从事于相干多普勒测风激光雷达的理论研究。在2014年,与中国科学院上海精密光学机械研究所合作研制了一台全光纤的相干多普勒测风激光雷达系统,基于该系统进行了一些模拟仿真的研制和数据处理算法的研究[96]。
2017年,209所范琪等[97]报道了全光纤相干多普勒测风激光雷达系统在不同天气类型下的探测性能,证明了晴天、阴天、雾霾天和雨天4种天气类型下该系统都具有良好的性能。
中国海洋大学Wu等[98]于2014年报道了1.55 μm全光纤相干多普勒测风激光雷达系统。并将其用于风电厂的风场观测。经过不断改造和升级后,推出了商用的WindPrint系统,并报道了该系统在不同地形下的探测性能,以及用于湍流探测的一些算法 [99-101]。2018年,冯长中等[101]提出基于共轭梯度算法的速度方位显示风场反演方法,应用最优化理论将共轭梯度算法代替传统傅里叶变换算法。
2017年,中国科学技术大学Wang等[102]成功研制了世界上第一台能同时观测大气退偏振比和大气风场的相干多普勒测风激光雷达。测风模式下该系统在100 μJ脉冲能量,15 kHz重复频率时,可以实现6 km的水平探测距离。相比于传统的相干测风激光雷达,该系统采用时分复用的模式,将回波信号通过偏振分束器(PBS)后,将P偏振态经过3 km的延时后到达探测器与本振光拍频,从而实现偏振态的测量。其装置图和系统测量结果如
同年,Wang等[103]提出利用联合时频分析的方法对相干激光雷达数据进行处理,可以清楚地检测出风切变的动态结构。相比于传统的快速傅里叶变换算法,自适应最优核时频分布具有运算量小、交叉项抑制效果好、时频聚集度高等优点。
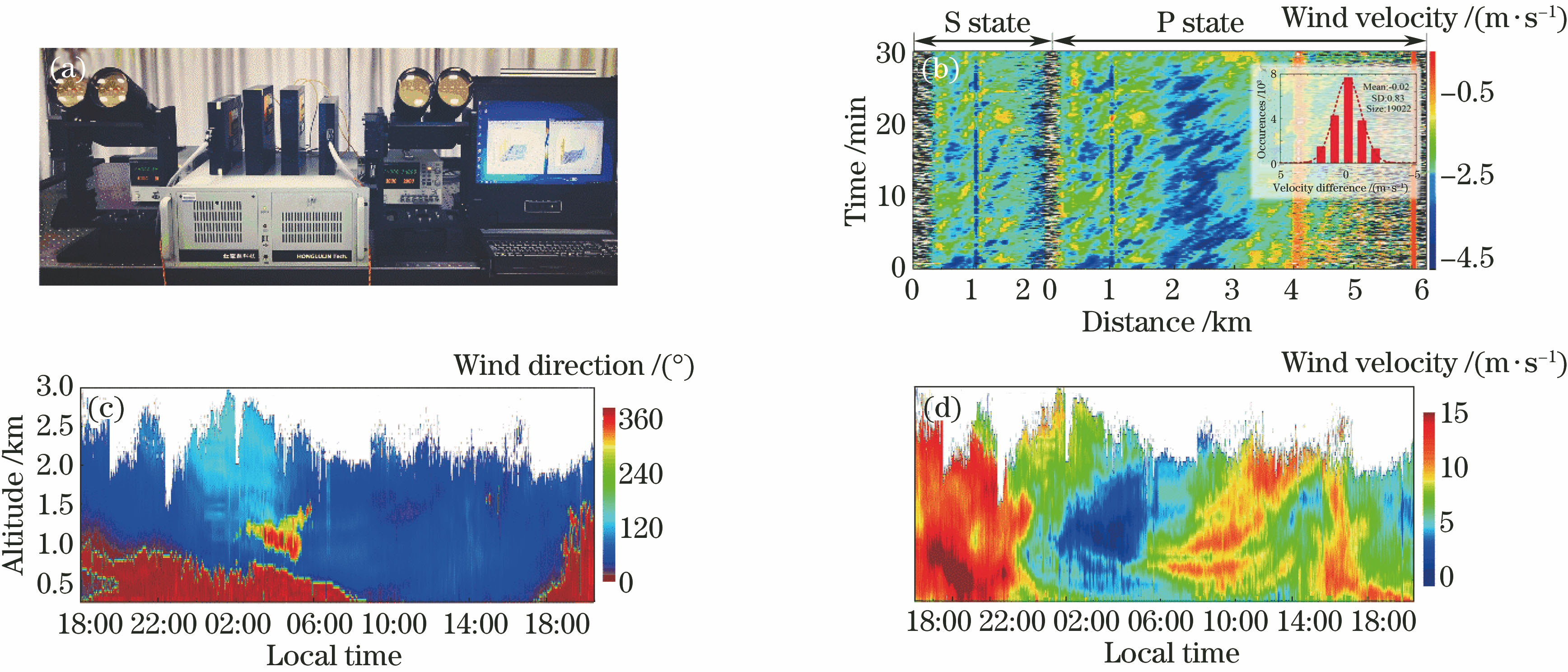
图 10. 中国科学技术大学全光纤测风激光雷达及风速风向测量结果。(a)相干激光雷达样机;(b)利用单平衡探测器从S态和P态反散射反演得到的风速,插图表示S态和P态之间的速度差;(c)水平风速;(d)水平风向
Fig. 10. All-fiber coherent wind lidar of USTC and results of wind velocity and direction. (a) Coherent lidar prototype; (b) wind velocity retrieved from both S and P states backscattering by single balanced detector, in which the inset shows the difference in velocity between S and P states; (c) horizontal wind speed; (d) horizontal wind direction
表 3. 国内相干激光雷达研究单位及指标
Table 3. Research institutes and parameters of coherent Doppler lidar in China
|
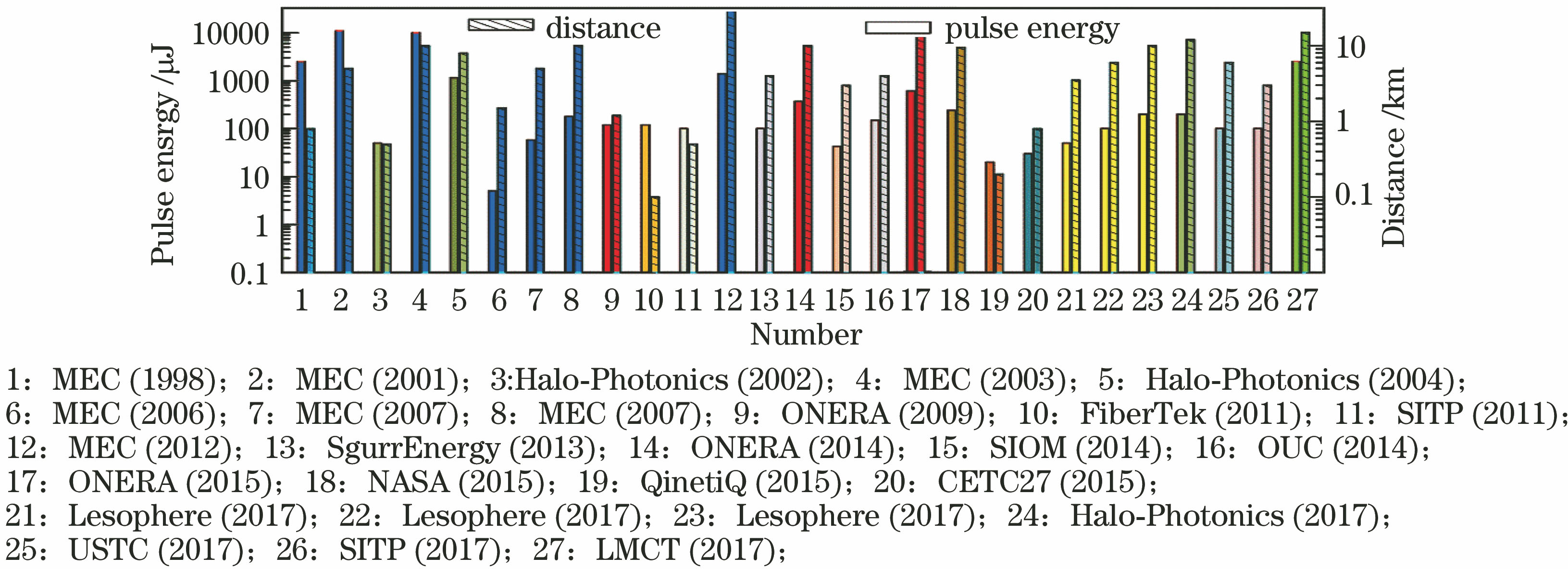
图 11. 1.5 μm激光雷达研究机构探测距离及脉冲能量指标
Fig. 11. Detection distance and pulse energy parameters of 1.5 μm lidar research institutions
2.5 2.0 μm相干激光雷达研究现状
2.0 μm波长在人眼安全方面优势巨大,比1.06 μm波长的人眼允许最大曝光功率高4个数量级。出于对人眼安全的考虑,2.0 μm波长的全固态相干多普勒测风激光雷达系统比1.06 μm系统应用领域更广泛。
第一台2.09 μm波长的相干多普勒测风激光雷达使用闪光灯抽运的Tm,Ho∶YAG激光器,由Henderson课题组在1990年首次报道 [104-105]。1991年,Henderson等[106]进一步优化了雷达系统,使用2.0 μm波长的激光器,在20 mJ的激光脉冲能量,20 cm直径的望远镜情况下,实现了20 km距离的水平风场探测和145 km的硬目标探测。基于Henderson的技术,美国相干技术公司使用纯半导体抽运的Tm∶YAG激光器,设计出了第一台2.01 μm的机载相干多普勒测风激光雷达,并于1994年使用NASA的波音737飞机进行机载实验,该系统使用InGaAs探测器,脉冲能量为1.8 mJ,脉冲宽度为0.5 μs,距离分辨率为75 m,探测到了风切变和微下击暴流[107]。1996年,该公司经过改进设计,进行了精度为10 cm/s的机载矢量风场探测。NOAA使用类似设计的相干多普勒测风激光雷达,基于船载[108]或地基系统[109-111],在空间分辨率最高为30 m情况下,对大气边界层进行了多年的高分辨率风场观测[112]。
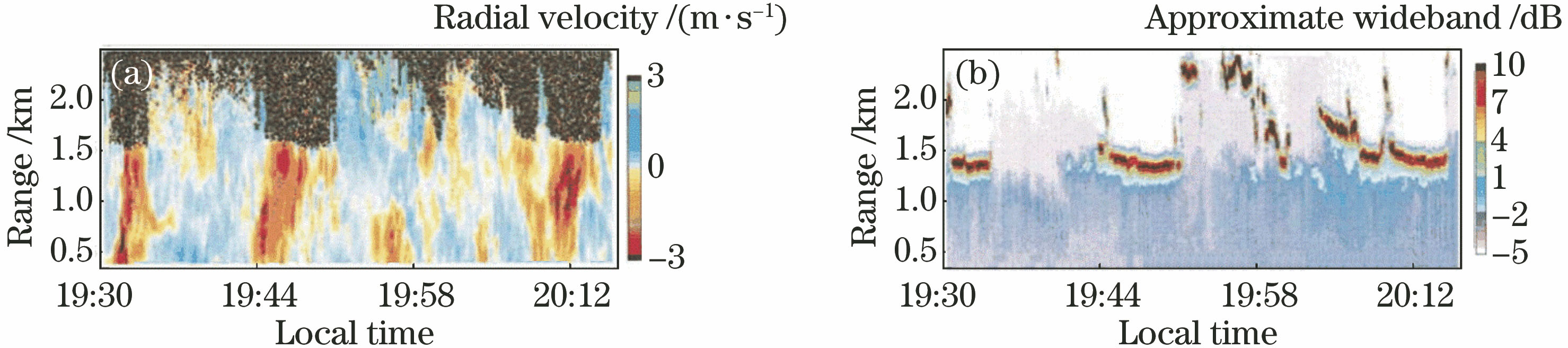
图 12. NOAA相干激光雷达风速测量结果。(a)径向风速数据;(b)对应的相对后向散射强度
Fig. 12. NOAA lidar wind velocity measurement results. (a) Radial velocity data; (b) corresponding relative backscatter intensity profile
NASA和美国空军将高脉冲能量(10 mJ),100 Hz脉冲重复频率的2 μm相干多普勒测风激光雷达系统用在机载测风应用上,用于晴空湍流探测以及飞机周边的风场探测。2012年,NASA的Kavaya等[117]报道了基于2 μm相干多普勒测风激光雷达风速测量结果。该系统采用的脉冲能量为250 mJ,重复频率为10 Hz,脉宽为180 ns。

图 13. NASA相干激光雷达风速风向测量结果。(a)风速;(b)风向
Fig. 13. NASA lidar wind velocity and wind direction results measurement results. (a) Wind velocity; (b) wind direction
单脉冲能量达到125 mJ的2.0 μm激光器系统还计划用于星载测风激光雷达系统[118],目前也有小部分2.0 μm的系统被集成在其他类型的雷达系统中[119]。
2010年,哈尔滨工业大学李彦超[120]建立了2.0 μm相干测风激光雷达系统,对理论模型、2.0 μm处的光学设计及信号流程算法进行了验证。其中激光器单脉冲能量为2 mJ,重复频率为100 Hz,脉冲宽度为300 ns,望远镜口径为150 mm,得到了16 m和96 m处探测目标外差信号。
2015年,北京理工大学步志超等[121]基于全微分和统计理论对2.0 μm星载相干测风激光雷达的风速及风向误差进行建模。建立了通用型的星载相干测风激光雷达合成水平风速和风向误差计算模型,朱振宇等[122]对天基相干激光雷达的关键技术进行了分析。
3 相干激光雷达应用
从上述相干激光雷达发展历程来看,目前其应用方向主要有以下几个方面:
1) 风切变测量。风切变是指风速矢量在空中水平或垂直距离上的变化。风切变会使飞机偏离路线,造成飞机失事。中国香港九龙天文台基于2.0 μm的相干激光雷达实现了风切变的追踪,其测量结果如
2) 飞机尾流测量。飞机的飞行过程会对大气产生扰动,其翼尖会产生涡旋流动场,严重威胁飞行器的飞行安全。ONERA基于1.5 μm机载相干激光雷达,对飞机产生的飞机轴向的涡流进行提取,其测量结果如
3) 湍流测量。大气湍流常在大气边界层由大气不规则运动产生,湍流扩散会伴随能量和物质交换,其产生的剪切力远大于层流运动,从而造成飞机飞行事故。NOAA基于相干激光雷达风速测量结果,反演出大气的湍流信息,并对混合层的高度进行了估计。
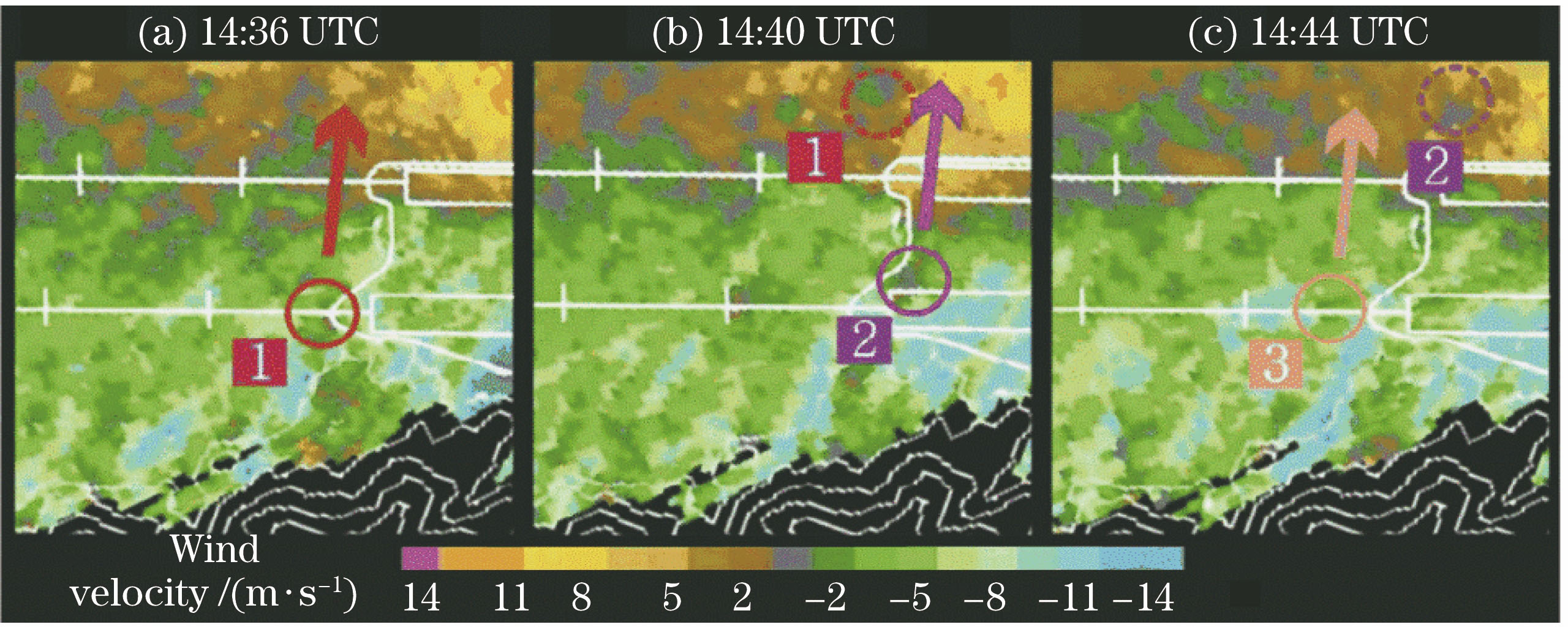
图 14. 中国香港九龙天文台测得的风切变
Fig. 14. Wind shear detected by Kowloon Observatory, Hong Kong, China

图 15. ONERA测得的飞机涡流。(a)尾流涡模型径向速度;(b)平均速度三维视图
Fig. 15. Aircraft wake vortex detected by ONERA. (a) Radial velocity of wake vortex model; (b) 3D view of mean velocity images
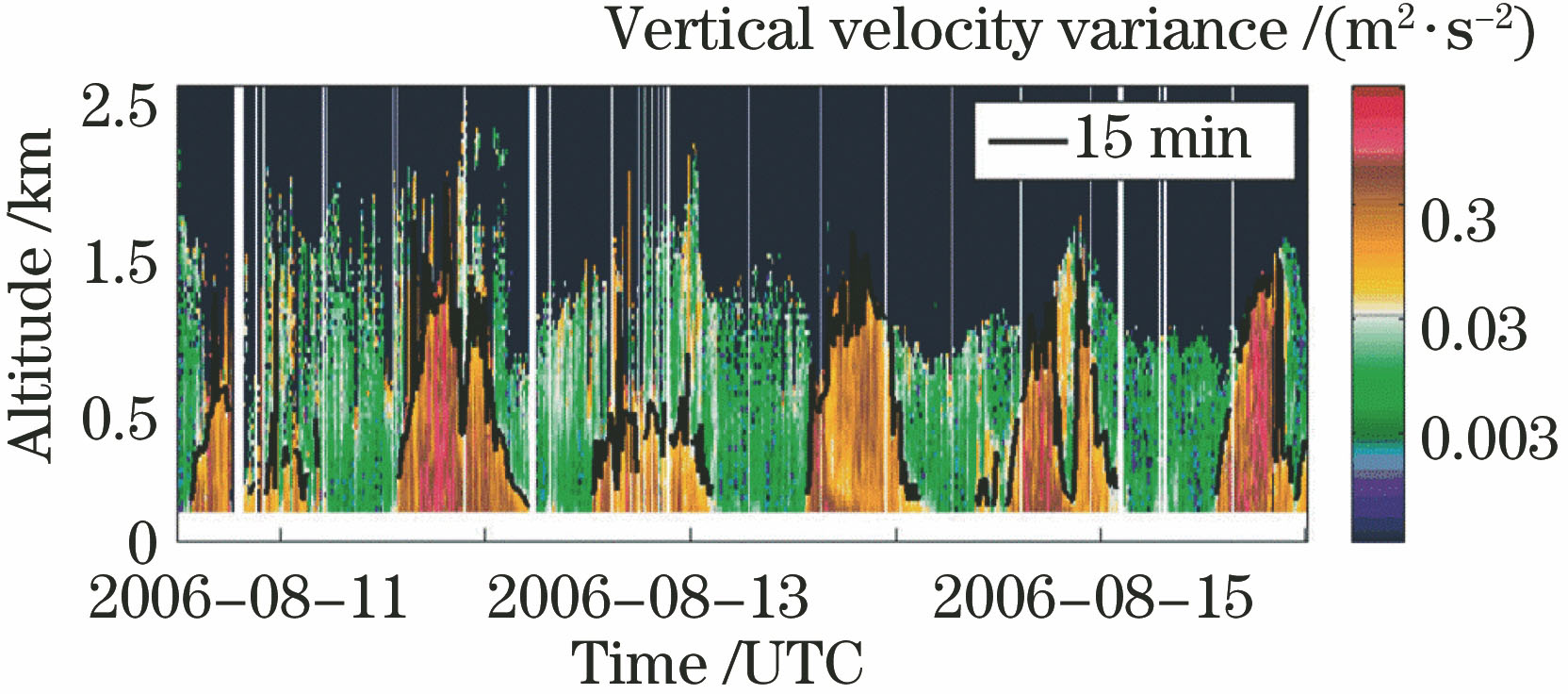
图 16. 垂直风速误差和混合层高度图
Fig. 16. Results of vertical wind velocity error and mixed layer height
4) 重力波分析。大气的温度、密度、风场等参数信息会随着重力波活动发生变化,重力波的产生与强对流活动、背景风场、灾害性天气联系也十分密切。DLR基于相干激光雷达的水平和垂直风速信息对纳维亚山脉的内部重力波进行表征。
5) 风能发电。风能作为新兴能源,其重要作用是风能发电,随着相干激光雷达稳健性的提高,其对大气研究和电力寻址等方面起着非常重要的作用。
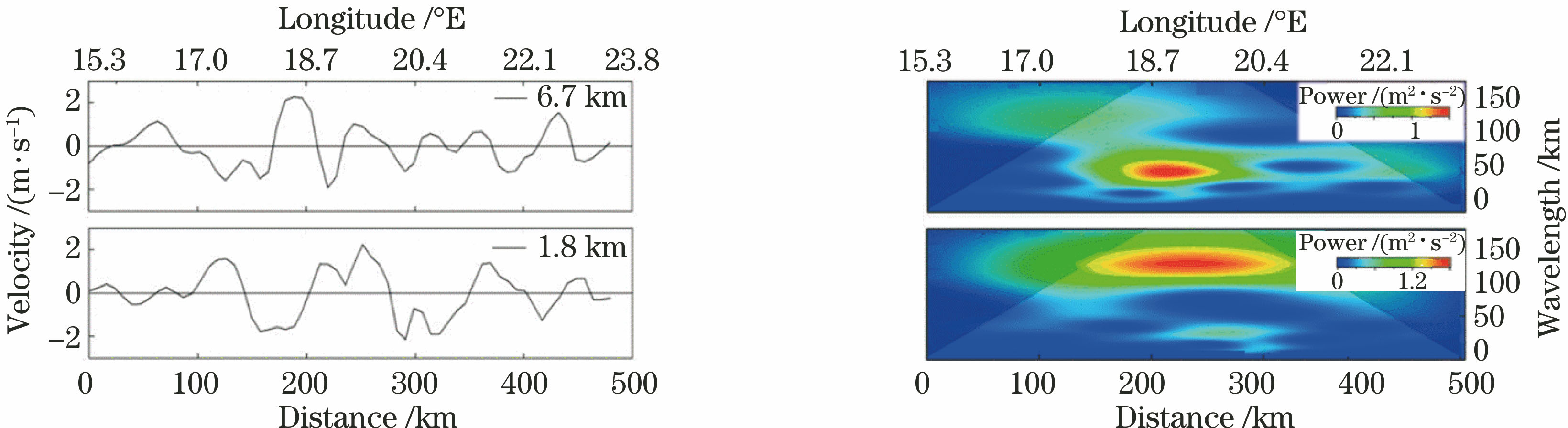
图 17. 1.8 km和6.7 km处的(a)水平风及(b)小波变换功率谱
Fig. 17. (a) Horizontal wind perturbations and (b)wavelet power spectra measured at 1.8 km and 6.7 km altitude
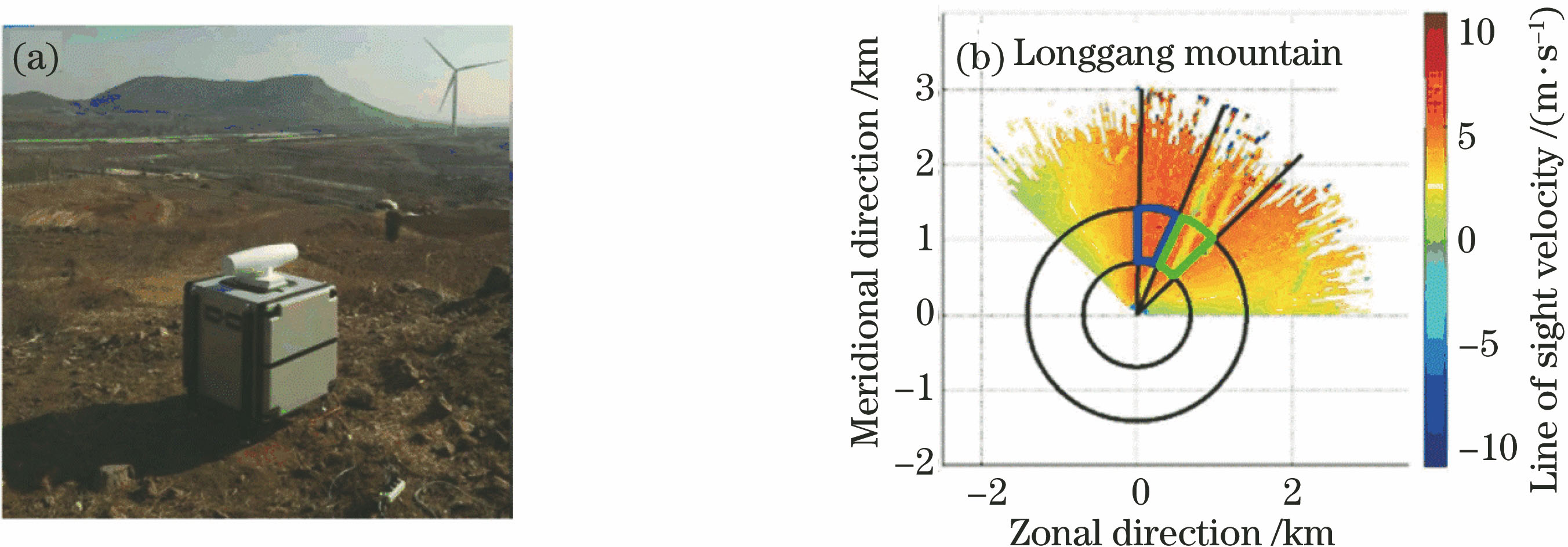
图 18. 中国海洋大学相干激光雷达风速测量结果。(a)雷达样机;(b)风速测量结果
Fig. 18. OUC lidar wind velocity measurement results. (a) Lidar prototype; (b) wind velocity measurement results
4 相干激光雷达发展趋势
通过对国内外相干激光雷达发展历史的总结,相干激光雷达呈现以下的发展趋势:
1) 相干激光雷达历经气态到全固态,再到全光纤相干测风激光雷达三个过程,激光器的波长也由10.6 μm到1.06 μm,再到2.0 μm和1.5 μm,其中主流波段主要在1.5 μm。
2) 由于风场的双向测量比单方向测量优势更明显,相干激光雷达也从以单一角度锥扫描发展到多角度扫描模式,实现了三维风场的探测。
3) 随着相干探测技术的提高,相干激光雷达探测风速灵敏度以及探测距离增加。但是由于气溶胶主要分布在5 km以下,随着探测距离增加气溶胶含量急剧下降,从而增加了回波信号的探测难度,因此,相干激光雷达测风垂直探测距离往往限制在5 km的高度。目前通过光纤放大器并联、抽运放大等方式可提高激光器能量,从而提高测量距离。
4) 人眼安全方面得到满足,1.55 μm附近MPE达到最大值,比2.1 μm高约1个数量级,比1.06 μm高约6个数量级。同时1.5 μm波段的光纤和光器件生产工艺成熟,大气窗口透过率高等优点也使得目前相干激光雷达研究的主流波段为1.55 μm。
5) 从商业化角度来看,国外实现商业集成化的公司较多,如Leosphere、Halo-Photonics公司、日本三菱电机公司等,而国内研究机构目前主要用于实验研究,相对成熟的相干激光雷达公司较少。
6) 从信号算法处理角度来看,从传统的快速傅里叶变换、周期图算法,到联合时频分析等信号处理方法应用,从时间-频率域刻画了信号全貌,提高了系统的距离分辨率。
5 结论
相干激光雷达因具有高精度、高时空分辨等优点被广泛应于用大气风场测量、航空航天安全等各个方面。随着激光技术的发展,相干激光雷达技术也不断成熟,相干激光雷达目前已经实现全光纤结构,并朝着小型化、集成化的趋势迈进,本文介绍的各研究机构成果对相干激光雷达的工作展开具有指导意义。
[2] QuantF, Farmer KR, Tan PV, et al. Handheld laser induced breakdown spectroscopy device: US9506869[P/OL]. 2016-11-29[2018-05-21]. http: ∥www. freepatentsonline. com/9506869. html.
[5] 上官明佳. 1.5 μm单光子探测器在激光遥感中的应用[D]. 合肥: 中国科学技术大学, 2017.
Shangguan MJ. Laser remote sensing with 1.5 μm single photon detectors[D]. Hefei: University of Science and Technology of China, 2017.
[7] 朱京平. 光电子技术基础[M]. 北京: 科学出版社, 2009.
Zhu JP. Optoelectronic technology foundation[M]. Beijing: Science Press, 2009.
[8] 夏海云. 基于气溶胶后向散射的双边缘直接探测多普勒测风激光雷达研究[D]. 苏州: 苏州大学, 2006.
Xia HY. Direct detection Doppler wind lidar based on aerosol backscattered signal with twin-channel Fabry-Perot etalon[D]. Suzhou: Soochow University, 2006.
[13] Woodfield AA, Vaughan JM. Using anairborne CO2 CW laser for free stream airspeed and wind shear measurements[Z]. [S. l. ]:AGARD Flight Test Techniques Series, 1984: 18.
[16] Bilbro J W. DiMarzio C, Fitzjarrald D, et al. Airborne Doppler lidar measurements[J]. Applied Optics, 1986, 25(21): 3952-3960.
[26] Kane T J, Byvik C E, Kozlovsky W J, et al. Coherent laser radar at 1.06 μm using Nd∶YAG lasers[J]. Optics Letters, 1987, 12(4): 239-241.
[29] Proctor FH, Hamilton DW. Evaluation of fast-time wake vortex prediction models[C]∥47th AIAA Aerospace Sciences Meeting Including The New Horizons Forum and Aerospace Exposition, January 5-8, 2009, Orlando, Florida. Virginia: AIAA, 2009: 344.
[31] Prasad N S, Sibell R, Vetorino S, et al. An all-fiber, modular, compact wind lidar for wind sensing and wake vortex applications[J]. Proceedings of SPIE, 2015, 9465: 94650C.
[32] Spuler S M, Richter D, Spowart M P, et al. Optical fiber-based laser remote sensor for airborne measurement of wind velocity and turbulence[J]. Applied Optics, 2011, 50(6): 842-851.
[33] Akbulut M, Hwang J, Kimpel F, et al. Pulsed coherent fiber lidar transceiver for aircraft in-flight turbulence and wake-vortex hazard detection[J]. Proceedings of SPIE, 2011, 8037: 80370R.
[34] Engin D, Mathason B, Stephen M, et al. High energy, narrow linewidth 1572 nm Er, Yb-fiber based MOPA for a multi-aperture CO2 trace-gas laser space transmitter[J]. Proceedings of SPIE, 2016, 9728: 97282S.
[35] KameyamaS, YanagisawaT, AndoT, et al. Development of wind sensing coherent Doppler LIDAR at Mitsubishi Electric Corporation-from late 1990s to 2013[C]∥Proceedings of 17th Coherent Laser Radar Conference, June 17-20, 2013, Barcelona, Spain. Huntsville, Alabama: Universities Space Research Association, 2013: 12- 13.
[36] Asaka K, Hirano Y, Morimoto Y, et al. Er, Yb∶glass coherent lidar using a microchip laser as a reference optical source[J]. The Review of Laser Engineering, 1998, 26(12): 876-880.
[37] Yanagisawa T, Asaka K, Hamazu K, et al. 11-mJ, 15-Hz single-frequency diode-pumped Q-switched Er, Yb∶ phosphate glass laser[J]. Optics Letters, 2001, 26(16): 1262-1264.
[38] Asaka K, Yanagisawa T, Hirano Y. 1.5 μm eye-safe coherent lidar system for wind velocity measurement[J]. Proceedings of SPIE, 2001, 4153: 321-329.
[39] Fujiyoshi Y, Yamashita K, Fujiwara C. Visualization of streaks, thermals and waves in the atmospheric boundary layer[J]. Journal of visualization, 2006, 9(4): 359-359.
[40] Kameyama S, Ando T, Asaka K, et al. Compact all-fiber pulsed coherent Doppler lidar system for wind sensing[J]. Applied Optics, 2007, 46(11): 1953-1962.
[41] Ando T, Kameyama S, Hirano Y. All-fiber coherent Doppler lidar technologies at Mitsubishi Electric Corporation[J]. IOP Conference Series: Earth and Environmental Science, 2008, 1(1): 012011.
[42] Chan P W, Lee Y F. Application of short-range lidar in wind shear alerting[J]. Journal of Atmospheric and Oceanic Technology, 2012, 29(2): 207-220.
[43] InokuchiH, TanakaH, Ando T. Development of a long range airborne Doppler lidar[C/OL]∥Proceedings of 27th Congress of International Council of the Aeronautical Sciences, ICAS, September 19-24, 2010, Nice, France. [S.l.: s.n.], 2010[2018-05-21]. http:∥icas. org/ICAS_ARCHIVE/ICAS2010/PAPERS/179. PDF.
[44] Inokuchi H, Endo E, Ando T, et al. Development of an airborne wind measurement system[J]. Proceedings of SPIE, 2009, 7328: 738205.
[45] Inokuchi H, Tanaka H, Ando T. Development of an onboard Doppler lidar for flight safety[J]. Journal of Aircraft, 2009, 46(4): 1411-1415.
[46] SakimuraT, WatanabeY, AndoT, et al. 3.2 mJ, 1.5 μm laser power amplifier using an Er, Yb∶glass planar waveguide for a coherent Doppler LIDAR[C]∥Proceedings of 17th Coherent Laser Radar Conference, June 17-20, 2013, Barcelona, Spain. Huntsville, Alabama: Universities Space Research Association, 2013: 35- 39.
[47] Kameyama S, Sakimura T, Watanabe Y, et al. Wind sensing demonstration of more than 30 km measurable range with a 1.5 μm coherent Doppler LIDAR which has the laser amplifier using Er, Yb∶glass planar waveguide[J]. Proceedings of SPIE, 2012, 8526: 85260E.
[48] InokuchiH, FurutaM, Inagaki T. High altitude turbulence detection using an airborne Doppler lidar[C/OL]∥Proceedings of 29th Congress of the International Council of the Aeronautical Sciences, September 7-12, 2014, Petersburg, Russia. [S.l.: s.n.], 2014[2018-05-21]. http:∥www. icas. org/ICAS_ARCHIVE/ICAS2014/data/papers/2014_0208_paper. pdf.
[49] Dolfi-BouteyreA, AugéreB, BessonC, et al. 1.5 μm all fiber pulsed lidar for wake vortex monitoring[C]∥Conference on Lasers and Electro-Optics, May 4-9, 2008, San Jose, California United States. Washington: Optical Society of America, 2008: CMQ3.
[50] CanatG, LombardL, DurécuA, et al. Er-Yb-Doped LMA fiber structures for high energy amplification of narrow linewidth pulses at 1.5 μm[C]∥Conference on Lasers and Electro-Optics, May 6-11, 2007, Baltimore, Maryland United States. Washington: Optical Society of America, 2007: CTuBB1.
[51] Dolfi-Bouteyre A, Canat G, Valla M, et al. Pulsed 1.5 μm LIDAR for axial aircraft wake vortex detection based on high-brightness large-core fiber amplifier[J]. IEEE Journal of Selected Topics in Quantum Electronics, 2009, 15(2): 441-450.
[52] Dolfi-BouteyreA, AugereB, VallaM, et al. Aircraft wake vortex study and characterization with 1.5 μm fiber Doppler lidar[J]. Aerospace Lab, 2009( 1): 1- 13.
[53] RenardW, GoularD, VallaM, et al. Beyond 10 km range wind-speed measurement with a 1.5 μm all-fiber laser source[C]∥CLEO: Applications and Technology, June 8-13, 2014, San Jose, California United States. Washington: Optical Society of America, 2014: AW1P. 5.
[54] Lombard L, Valla M, Planchat C, et al. Eyesafe coherent detection wind lidar based on a beam-combined pulsed laser source[J]. Optics Letters, 2015, 40(6): 1030-1033.
[55] Lombard L, Dolfi-Bouteyre A, Besson C, et al. Long range wind lidars based on novel high spectral brilliance all-fibered sources[J]. Proceedings of SPIE, 2015, 9645: 96450B.
[56] Thobois LP, KrishnamurthyR, LoaecS. Wind and EDR measurements with scanning Doppler LIDARs for preparing future weather dependent separation concepts[C]∥7th AIAA Atmospheric and Space Environments Conference, June 22-26, 2015, Dallas, Texas, USA. Virginia: AIAA, 2015: 3317.
[57] ThoboisL, LoaecS, BoquetM, et al. Recent developments of WINDCUBE Doppler Lidars for airport wind hazards monitoring[R/OL]∥WakeNet-Eu2014Workshop, 2014 [2018-05-21]. http:∥www. wakenet. eu/fileadmin/user_upload/Workshop2014/Presentations/WakeNetEurope_Workshop2014_504_Thobois. pdf.
[58] VranckenP, WirthM, RempelD, et al. Clear air turbulence detection and characterization in the DELICAT airborne lidar project[C/OL]∥Proceedings of the 25th International Laser Radar Conference, July 5-9, Petersburg, Russia, [S.l.:s.n.].2010 [ 2018-05-21]. https: ∥elib.dlr.de/67271/1/Seiten_aus_ILRC_25_PROCEEDINGS_VOL_1. pdf.
[59] Besson C, Augere B, Canat G, et al. New fiber laser for lidar developments in disaster management[J]. Proceedings of SPIE, 2014, 9250: 92500H.
[60] BarbarescoF, ThoboisL, Dolfi-BouteyreA, et al. Monitoring wind, turbulence and aircraft wake vortices by high resolution RADAR and LIDAR remote sensors in all weather conditions[C/OL]∥Proceedings of URSI France JS 15, Paris. [S.l.:s.n.]. 2015: 81-110[2018-05-21]. http:∥webistem.com/ursi-f2015/output_directory/cd1/data/articles/000033.pdf.
[61] HallermeyerA, Dolfi-BouteyreA, VallaM, et al. Development and assessment of a Wake Vortex characterization algorithm based on a hybrid LIDAR signal processing[C]∥8th AIAA Atmospheric and Space Environments Conference, June 13-17, 2016, Washington, DC. Virginia: AIAA, 2016: 3272.
[62] KigleS. Wake identification and characterization of a full scale wind energy converter in complex terrain with scanning Doppler wind Lidar systems[D]. München:Ludwig-Maximilians-Universität München, 2017.
[63] AugrosC, TabaryP, DavrincheD, et al. Test of an X-band Doppler polar metric radar combined with a Doppler LIDAR for wind shear detection at Nice Airport[C/OL]∥The Seventh European Conference on Radar in Meteorology and Hydrology, June 25-29, 2012, Toulouse, France. [S.l.:s.n.], 2012[2018-05-21]. http:∥www.meteo.fr/cic/meetings/2012/ERAD/extended_abs/ATM_014_ext_abs.pdf.
[64] GibertF, DumasA, ThoboisL, et al. Afternoon transition turbulence decay revisited by Doppler Lidar[C/OL]∥Symposium on Boundary Layer and Turbulence, July 8-13, 2012, Boston, USA. [2018-05-21]. http:∥bllast.sedoo.fr/workshops/february2016/presentations/FabienGibert_TKE-budget.pdf.
[65] Chen Y, An J, Wang X, et al. Observation of wind shear during evening transition and an estimation of submicron aerosol concentrations in Beijing using a Doppler wind lidar[J]. Journal of Meteorological Research, 2017, 31(2): 350-362.
[66] Karlsson C J, Olsson F A, Letalick D, et al. All-fiber multifunction continuous-wave coherent laser radar at 1.55 μm for range, speed, vibration, and wind measurements[J]. Applied Optics, 2000, 39(21): 3716-3726.
[67] Harris M, Constant G, Ward C. Continuous-wave bistatic laser Doppler wind sensor[J]. Applied Optics, 2001, 40(9): 1501-1506.
[68] Jørgensen HE, MikkelsenT, MannJ, et al. Site wind field determination using a CW Doppler LIDAR-comparison with cup anemometers at Risø[C]∥Special Topic Conference: The Science of Making Torque from Wind, April 19-21, 2004. Delft, Netherlands. [S. l. ]: Delft University of Technology, 2004: 261- 266.
[69] Smith D A, Harris M, Coffey A S, et al. Wind lidar evaluation at the Danish wind test site in Høvsøre[J]. Wind Energy, 2006, 9(1/2): 87-93.
[70] BingölF. Adapting a Doppler laser anemometer to wind energy[D]. Lyngby: Technical University of Denmark, 2005.
[71] Pearson G N, Roberts P J, Eacock J R, et al. Analysis of the performance of a coherent pulsed fiber lidar for aerosol backscatter applications[J]. Applied Optics, 2002, 41(30): 6442-6450.
[72] Wang H, Barthelmie R J, Crippa P, et al. Profiles of wind and turbulence in the coastal atmospheric boundary layer of Lake Erie[J]. Journal of Physics: Conference Series, 2014, 524(1): 012117.
[73] Gottschall J, Wolken-Möhlmann G, Lange B. About offshore resource assessment with floating lidars with special respect to turbulence and extreme events[J]. Journal of Physics: Conference Series, 2014, 555(1): 012043.
[74] Gottschall J. Galion lidar performance verification[R/OL]. Fraunhofer IWES, 2013[ 2018-05-21]. https:∥www. woodgroup.com/__data/assets/pdf_file/0023/15692/report_Sgurr_20130529_FINAL1.pdf.
[75] Pearson G N, Eacock J R. Fiber-based coherent pulsed Doppler lidar for atmospheric monitoring[J]. Proceedings of SPIE, 2002, 4484: 51-58.
[76] Philippov V N, Sahu J K, Codemard C A, et al. All-fiber 1.15-mJ pulsed eye-safe optical source[J]. Proceedings of SPIE, 2004, 5335: 1-8.
[77] Pearson G N, Ridley K D, Willetts D V. Long range 3D active imagery with a scanned single element 1.5 μm coherent lidar system[J]. Proceedings of SPIE, 2005, 5988: 59880M.
[78] Collier CG, DaviesF, DaviesJ, et al. Doppler radar and lidar observations of a thunderstorm outflow[C]∥Proceedings of Fifth European Conference on Radar in Meteorology and Hydrology, June 30-July 4, 2008, Helsinki. [S.l.:s.n.], 2008-12-04.
[79] Hogan R J. Grant A L M, Illingworth A J, et al. Vertical velocity variance and skewness in clear and cloud-topped boundary layers as revealed by Doppler lidar[J]. Quarterly Journal of the Royal Meteorological Society, 2009, 135(640): 635-643.
[80] Pearson G, Davies F, Collier C. Remote sensing of the tropical rain forest boundary layer using pulsed Doppler lidar[J]. Atmospheric Chemistry and Physics, 2010, 10(13): 5891-5901.
[81] Westbrook C D, Illingworth A J. O'Connor E J, et al. Doppler lidar measurements of oriented planar ice crystals falling from supercooled and glaciated layer clouds[J]. Quarterly Journal of the Royal Meteorological Society, 2010, 136(646): 260-276.
[82] O’Connor E J, Illingworth A J, Brooks I M, et al. . A method for estimating the turbulent kinetic energy dissipation rate from a vertically pointing Doppler lidar, and independent evaluation from balloon-borne in situ measurements[J]. Journal of Atmospheric and Oceanic Technology, 2010, 27(10): 1652-1664.
[83] Abari C F, Pedersen A T, Mann J. An all-fiber image-reject homodyne coherent Doppler wind lidar[J]. Optics Express, 2014, 22(21): 25880-25894.
[84] Pedersen A T, Abari C F, Mann J, et al. Theoretical and experimental signal-to-noise ratio assessment in new direction sensing continuous-wave Doppler lidar[J]. Journal of Physics: Conference Series, 2014, 524(1): 012004.
[85] Abari C F, Dellwik E, Mann J. Performance evaluation of an all-fiber image-reject homodyne coherent Doppler wind lidar[J]. Atmospheric Measurement Techniques, 2015, 8(10): 4145-4153.
[86] Abari C F, Chu X, Hardesty R M, et al. A reconfigurable all-fiber polarization-diversity coherent Doppler lidar: principles and numerical simulations[J]. Applied Optics, 2015, 54(30): 8999-9009.
[87] Hu Q, Rodrigo P J, Pedersen C. Remote wind sensing with a CW diode laser lidar beyond the coherence regime[J]. Optics Letters, 2014, 39(16): 4875-4878.
[88] Rodrigo P J, Pedersen C. Comparative study of the performance of semiconductor laser based coherent Doppler lidars[J]. Proceedings of SPIE, 2012, 8241: 824112.
[89] 李冬梅, 郑永超, 潘静岩, 等. 相干多普勒激光测风雷达系统研究[J]. 光学技术, 2010, 6: 880-884.
Li D M, Zheng Y C, Pan J Y, et al. Index system of coherence Doppler wind lidar[J]. Optics Technology, 2010, 6: 880-884.
[90] 潘静岩, 邬双阳, 刘果, 等. 相干激光测风雷达风场测量技术[J]. 红外与激光工程, 2013, 42(7): 1720-1724.
Pan J Y, Qin S Y, Liu G, et al. Coherent laser wind measurement radar wind field measurement technology[J]. Infrared and Laser Engineering, 2013, 42(7): 1720-1724.
[91] 封治华, 杜改丽. 相干多普勒激光测风雷达系统研究及验证[J]. 激光与红外, 2015, 45(2): 128-132.
Feng Z H, Du G L. Research and verification of coherent Doppler wind lidar[J]. Laser and Infrared, 2015, 45(2): 128-132.
[92] 冯力天, 郭弘其, 陈涌, 等. 1.55 μm全光纤多普勒测风雷达系统与试验[J]. 红外与激光工程, 2011, 40(5): 844-847.
Feng L T, Guo H Q, Chen Y, et al. Experiment of all fiber Doppler liar at 1.55 μm[J]. Infrared and Laser Engineering, 2011, 40(5): 844-847.
[93] LiuJ, ChenW, ZhuX. Development of all-fiber coherent Doppler LIDAR to measure atmosphere wind speed[C]∥Optical Instrumentation for Energy and Environmental Applications, November 11-14, 2012. Eindhoven Netherlands. Washington: Optical Society of America, 2012: ET4D. 1.
[95] 刁伟峰, 刘继桥, 竹孝鹏, 等. 全光纤相干多普勒激光雷达非线性最小二乘风速反演方法及实验研究[J]. 中国激光, 2015, 42(9): 0914003.
[96] Bu Z, Zhang Y, Chen S, et al. Noise modeling by the trend of each range gate for coherent Doppler LIDAR[J]. Optical Engineering, 2014, 53(6): 063109.
[97] 范琪, 朱克云, 郑佳锋, 等. 不同天气类型下全光纤相干激光测风雷达探测性能分析[J]. 中国激光, 2017, 44(2): 0210003.
[98] Wu S, Yin J, Liu B, et al. Characterization of turbulent wake of wind turbine by coherent Doppler lidar[J]. Proceedings of SPIE, 2014, 9262: 92620H.
[99] Zhai X, Wu S, Liu B. Doppler lidar investigation of wind turbine wake characteristics and atmospheric turbulence under different surface roughness[J]. Optics Express, 2017, 25(12): A515-A529.
[100] Wu S, Liu B, Liu J, et al. Wind turbine wake visualization and characteristics analysis by Doppler lidar[J]. Optics Express, 2016, 24(10): A762-A780.
[101] 冯长中, 吴松华, 刘秉义. 相干多普勒激光雷达风场反演方法研究与实验印证[J]. 中国激光, 2018, 45(4): 0410001.
[102] Wang C, Xia H, Shangguan M, et al. 1.5 μm polarization coherent lidar incorporating time-division multiplexing[J]. Optics Express, 2017, 25(17): 20663-20674.
[103] Wang C, Xia H, Liu Y, et al. Spatial resolution enhancement of coherent Doppler wind lidar using joint time-frequency analysis[J]. Optics Communications, 2018, 424: 48-53.
[104] Henderson S W, Hale C P, Magee J R, et al. Eye-safe coherent laser radar system at 2.1 μm using Tm, Ho∶YAG lasers[J]. Optics Letters, 1991, 16(10): 773-775.
[105] Suni P J M, Henderson S W. 1-mJ/pulse Tm∶YAG laser pumped by a 3-W diode laser[J]. Optics Letters, 1991, 16(11): 817-819.
[106] Henderson S W. Suni P J M, Hale C P, et al. Coherent laser radar at 2 μm using solid-state lasers[J]. IEEE Transactions on Geoscience and Remote Sensing, 1993, 31(1): 4-15.
[107] Targ R, Steakley B C, Hawley J G, et al. Coherent lidar airborne wind sensor II: flight-test results at 2 and 10 μm[J]. Applied Optics, 1996, 35(36): 7117-7127.
[108] Wulfmeyer V, Randall M, Brewer A, et al. 2-μm Doppler lidar transmitter with high frequency stability and low chirp[J]. Optics Letters, 2000, 25(17): 1228-1230.
[109] Lenschow D H, Wulfmeyer V, Senff C. Measuring second-through fourth-order moments in noisy data[J]. Journal of Atmospheric and Oceanic Technology, 2000, 17(10): 1330-1347.
[110] Newsom R K, Banta R M. Shear-flow instability in the stable nocturnal boundary layer as observed by Doppler lidar during CASES-99[J]. Journal of the Atmospheric Sciences, 2003, 60(1): 16-33.
[111] Poulos G S, Blumen W, Fritts D C, et al. CASES-99: A comprehensive investigation of the stable nocturnal boundary layer[J]. Bulletin of the American Meteorological Society, 2002, 83(4): 555-581.
[112] Grund C J, Banta R M, George J L, et al. High-resolution Doppler lidar for boundary layer and cloud research[J]. Journal of Atmospheric and Oceanic Technology, 2001, 18(3): 376-393.
[113] Frehlich R, Hannon S M, Henderson S W. Performance of a 2-μm coherent Doppler lidar for wind measurements[J]. Journal of Atmospheric and Oceanic Technology, 1994, 11(6): 1517-1528.
[114] Frehlich R, Hannon S M, Henderson S W. Coherent Doppler lidar measurements of winds in the weak signal regime[J]. Applied Optics, 1997, 36(15): 3491-3499.
[115] Henderson S W, Yuen E H, Hannon S M. Autonomous lidar wind field sensor: design and performance[J]. Proceedings of SPIE, 1999, 3757: 18-28.
[116] Hannon S M. Autonomous infrared Doppler radar: Airport surveillance applications[J]. Physics and Chemistry of the Earth, Part B: Hydrology, Oceans and Atmosphere, 2000, 25(10/11/12): 1005-1011.
[117] Kavaya M J, Beyon J Y, Koch G J, et al. The Doppler aerosol wind (DAWN) airborne, wind-profiling coherent-detection Lidar system: overview and preliminary flight results[J]. Journal of Atmospheric and Oceanic Technology, 2014, 31(4): 826-842.
[118] Yu J, Singh U N, Barnes N P, et al. 125-mJ diode-pumped injection-seeded Ho∶Tm∶YLF laser[J]. Optics Letters, 1998, 23(10): 780-782.
[119] Koch G J, Petros M, Barnes B W, et al. Validar: a testbed for advanced 2-micron Doppler lidar[J]. Proceedings of SPIE, 2004, 5412: 87-98.
[120] 李彦超. 2 μm激光测风雷达外差探测方法与关键技术研究[D]. 哈尔滨: 哈尔滨工业大学, 2012.
Li YC. Study on heterodyne method and key technology for 2-micrometer wind lidar[D]. Harbin: Harbin Institute of Technology, 2012.
[121] 步志超, 陈思颖, 张寅超, 等. 2 μm星载相干测风激光雷达风速及风向误差建模与分析[J]. 红外与毫米波学报, 2015, 34(4): 465-470.
[122] 朱振宇, 高昆, 韩璐, 等. 天基相干测风激光雷达技术分析[J]. 激光与光电子学进展, 2015, 52(10): 101201.
[123] Shun C M, Chan P W. Applications of an infrared Doppler lidar in detection of wind shear[J]. Journal of Atmospheric and Oceanic Technology, 2008, 25(5): 637-655.
[124] Dolfi-Bouteyre A, Canat G, Valla M, et al. Pulsed 1.5-μm LIDAR for axial aircraft wake vortex detection based on high-brightness large-core fiber amplifier[J]. IEEE Journal of Selected Topics in Quantum Electronics, 2009, 15(2): 441-450.
[125] Tucker S C, Senff C J, Weickmann A M, et al. Doppler lidar estimation of mixing height using turbulence, shear, and aerosol profiles[J]. Journal of Atmospheric and Oceanic Technology, 2009, 26(4): 673-688.
[126] Witschas B, Rahm S, Dörnbrack A, et al. Airborne wind lidar measurements of vertical and horizontal winds for the investigation of orographically induced gravity waves[J]. Journal of Atmospheric and Oceanic Technology, 2017, 34(6): 1371-1386.
Article Outline
周艳宗, 王冲, 刘燕平, 夏海云. 相干测风激光雷达研究进展和应用[J]. 激光与光电子学进展, 2019, 56(2): 020001. Yanzong Zhou, Chong Wang, Yanping Liu, Haiyun Xia. Research Progress and Application of Coherent Wind Lidar[J]. Laser & Optoelectronics Progress, 2019, 56(2): 020001.