基于表面等离子体共振的高灵敏度光纤微流控芯片
下载: 1630次
Objective Surface plasmon resonance (SPR) sensors based on Kretschmann prism are bulky and need to be equipped with mechanical movable parts, which is not conducive to the miniaturization and remote sensing of devices. Optical-fiber-based SPR technology has been widely used in food safety, environmental monitoring, and other fields owing to its label-free simple pretreatment, fast analysis, high sensitivity, and other excellent characteristics. However, most SPR sensors do not consider sample consumption in the process of sensor monitoring. The exposed optical fiber is fragile, which easily affects the sensor's stability. Highly sensitive optical fibers, such as tapered and D-shaped fibers, are vulnerable and unstable. In addition to the abovementioned problems, the sensitivity of existing SPR fiber sensors needs further improvement. As a high-throughput microscale analysis device, the microfluidic chip system has shown great potential in remote monitoring, biological detection, and other fields in recent years. In this paper, a microfluidic chip based on SPR fiber sensor is designed and used to measure the concentration of a solution. This chip has the advantages of small size, compact structure, and low sample consumption; it also reduces the fiber damage and effectively improves the sensing stability by embedding the sensing fiber into Polydimethylsiloxane (PDMS) substrate. Photonic crystal fiber (PCF) is a new type of optical fiber. PCF comprises a single dielectric, in which air holes are closely arranged in the two-dimensional direction but unchanged in the axial structure to form microstructure cladding. Moreover, it can stimulate a more substantial SPR effect for its unique light-guiding characteristics.
Methods In this paper, PDMS—which has good chemical inertia and good biocompatibility—is used as the main material to fabricate the microfluidic chip. After cooling and forming in 3D mold, PDMS is stably bonded with glass sheet to form the main structure of the chip. The microchip contains a microfluidic channel (diameter: 200nm), whose length is the same as that of the chip. A sensing structure of multimode fiber-photonic crystal fiber-multimode fiber (MMF-PCF-MMF), which is coated with 60-nm gold film on the surface, is embedded in the channel to stimulate SPR effect. Then, mixed solutions of glucose solid sample and deionized water in a certain proportion with refractive index of 1.338--1.425 are used as samples to be tested. The experiment is performed at room temperature (25 °C). The glucose solution in the syringe is injected into the microfluidic chip using a syringe pump at a constant speed; the glucose solution flows through the optical fiber sensing part. When the concentration of the solution injected into the microfluidic chip is changed, the light wave meeting the resonance conditions excites the gold film to produce surface plasmon resonance and the resonant valley is generated on the transmitted spectrum. The position of the resonant valley shifts in the samples with different refractive indexes. By recording the resonance spectra of a series of samples, the sensitivity of the sensor with respect to the change in refractive index can be calibrated.
Results and Discussions When the refractive index of the liquid injected into the microfluidic chip increases from 1.338 to 1.425, the resonant wavelength of SPR spectrum shifts to longer wavelength because of the SPR resonance effect. The relationship between the refractive index and resonant wavelength of the liquid to be measured is extracted, and the sensitivity curve can be obtained by taking the resonant wavelength at n=1.338 as the starting point. The increasing speed of the sensitivity curve is related to the chip sensitivity. The experimental results show that the refractive index sensitivity of PCF SPR sensors can reach up to 8240.6nm/RIU, in which RIU is refractive index unit, thereby showing that these have good high-sensitivity characteristics and meet the application requirements of high sensitivity.
Conclusions In this paper, a novel microfluidic chip embedded with SPR sensor is designed and manufactured by combining the optical fiber structure, SPR effect, and microfluidic system. The volume of chip is approximately 3.5cm×1cm×5cm, which is much smaller than that of the traditional measurement instrument and is conducive to the integration of sensor. After testing the MMF-PCF-MMF structure based on SPR effect, the sensor sensitivity obtained is high (up to 8240.6nm/RIU) in a wide refractive index measurement range of 1.338--1.425. The proposed structure has good refractive sensitivity, small size, acid resistance, and corrosion resistance; these characteristics enable a broader application prospect in the field of biochemistry.
1 引言
光纤表面等离子体共振(SPR)技术作为一种近代物理光学、材料化学等学科交叉结合的新兴传感技术,具有免标记、预处理简单、分析快速、高灵敏等优良特性,逐渐在食品安全、环境监测等众多领域中得到广泛使用[1-3] ,目前国内外已研制出多种基于SPR的浓度传感器。吉林大学高璐等[4]设计了一种基于Kretschmann型棱镜的SPR原理折射率检测实验系统,通过MATLAB仿真得到其理论检测灵敏度为6049nm/RIU(RIU为单位折射率),实际检测出来的实验结果与仿真结果近似相等。然而基于Kretschmann型棱镜型的SPR传感器体积庞大,需配备机械可动部件,不利于器件小型化和远程传感。光纤SPR传感器凭借其体积小、结构紧凑以及可实现远程测量的优势,越来越受到研究人员的广泛关注。南京信息工程大学蔡凯杰[5]设计并制作了一种基于D型光纤的SPR传感器,在介质折射率1.33~1.39范围内,基于金膜激励SPR效应时灵敏度达2851nm/RIU,基于银膜时灵敏度更高,达3319nm/RIU;南京信息工程大学郭志勇等[6]设计了一种基于多模-单模-多模(MSM)结构的光纤折射率传感器,并且在单模光纤表面涂覆二氧化钛/银(TiO2/Ag)复合膜构成传感单元,Ag膜厚度与TiO2膜厚度达到传感器性能最优条件时,在1.33~1.41环境折射率范围内,传感器灵敏度约为6875nm/RIU。基于上述方法研制的光纤SPR 折射率传感器,虽然灵敏度较高,但大多未考虑传感监测过程中样品的消耗量,且裸露的光纤易碎,传感器的稳定性不足。
微流控芯片系统作为高通量微尺度分析设备,近年来在环境检测、远距离监控、生物检测等多种领域展现出巨大的潜力[7]。本研究将光纤SPR与微流控通道相结合,形成简单的微量化系统,大幅减少了样品消耗量,并将传感光纤部分嵌入有机聚合材料聚二甲基硅氧烷(PDMS)基片,以防止其受到损坏而对传感器稳定性造成影响,同时使用光子晶体光纤(PCF)与多模光纤(MMF)熔接而成的异芯结构作为传感部分,在传感结构表面镀60nm厚的金膜,以激励SPR效应。其中,PCF是近年来出现的一种新型光纤,这种光纤通常由单一电介质构成,其微结构包层由二维方向上紧密排列而在轴向结构不变的波长量级空气孔组成,具有独特的导光特性,能够激发更强的SPR效应[8-9]。本文所提出的传感器的最高检测灵敏度为8240.6nm/RIU,在灵敏度方面有较大提升,另外微流控芯片具有体积小、结构轻便、便于携带、性能稳定等特点,即使在非实验室环境下测量仍具有较高准确度与可靠性,不易受环境的影响。
2 基本原理与实验过程
2.1 基本原理
SPR是金属表面存在的电荷密度波被激发并沿金属与介质表面传播的物理光学现象[10]。如
当入射光波的入射角固定时,发生共振的入射光频率与待测溶液的折射率相关。对于基于光子晶体光纤的表面等离子共振,只有水平方向的分量起作用。假定光波波矢k在x轴方向的分量大小kx为[11-12]
其中ω为入射光的角频率,c为光速,θ为可见光的
入射角,ξ0(λ)为纤芯的介电常数。金属和介质表面的等离子体波的波矢大小ksp可以表示为
其中ξ1(λ)为待测液体的介电常数,ξm(λ)为金属介质的介电常数。由(1)式和(2)式可以看出,入射光波长、纤芯的介电常数、入射角度、待测溶液的介电常数以及金属介质的介电常数等因素都会影响入射光波与等离子体的相位匹配。当ksp=kx时,等离子体模式与纤芯导模模式满足相位匹配条件,产生的等离子共振效应最强,对应的共振角度为
当宽带光源以一定角度入射光纤时,可通过检测输出光谱的特性曲线得到吸收峰对应的光波长。普通光纤用作传感光纤时存在耦合损耗大、保偏性差等缺点,限制了SPR传感器性能的进一步提高[13],而PCF可以通过向纤芯中引入中空微结构来降低模式折射率,因此可以方便地实现纤芯模和表面等离子模的相位匹配,获得更宽的检测范围和更高的分辨率[6]。本文所提出的PCF结构及其包层模式的光场能量分布如
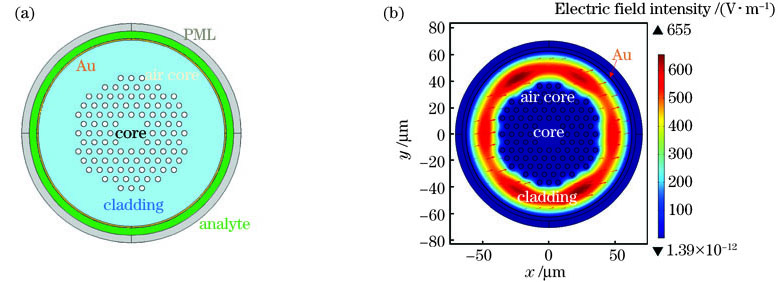
图 2. 仿真结果。(a)光子晶体光纤结构图 ;(b)包层模式的光场能量分布图
Fig. 2. Simulation results. (a) Schematic diagram of PCF; (b) light field energy distribution of the cladding mode
2.2 传感结构制作
光纤传感结构是由两段MMF与一段长约10mm的PCF熔接而成的多模干涉结构。制成的传感光纤结构如
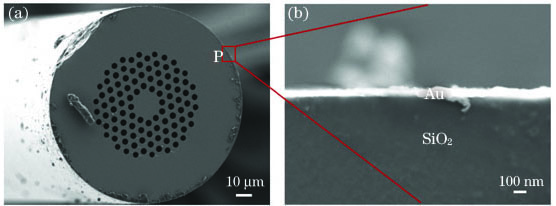
图 4. 光子晶体光纤结构图。(a)光子晶体光纤的SEM剖面图;(b) PCF边界上的位置缩放图
Fig. 4. Structure diagram of photonic crystal fiber. (a) SEM profile of PCF; (b) zoom on PCF
2.3 芯片设计
本课题组所设计的光纤SPR芯片结构如
芯片的制作材料选用具有良好化学惰性的可塑形有机材料PDMS。制作时首先将主剂与固化剂以10∶1混合均匀,缓缓倒入3D打印而成的树脂模具中。将倒满混合胶体的3D模具置于通风处1~2d,待其PDMS胶体静置凝固,切割取出PDMS芯片。最后将事先制作完成的传感光纤置于PDMS芯片上预留的微流控通道中,并与玻璃片稳定键合。
2.4 实验过程
实验装置如
3 分析与讨论
在上述实验条件下得到的SPR光谱曲线如
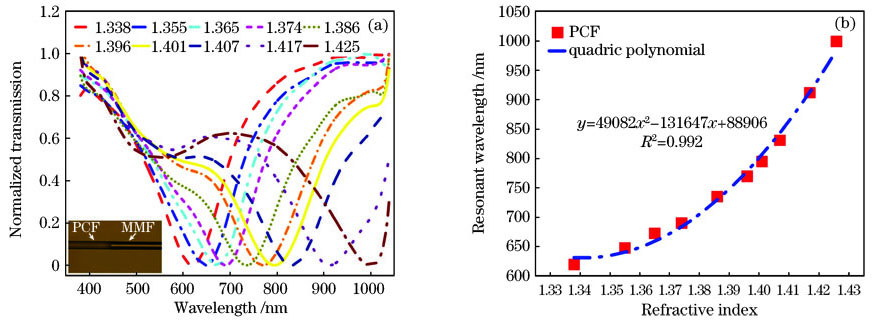
图 7. 实验结果。(a)基于PCF-SPR传感器的归一化透射光谱;(b) PCF-SPR结构的谐振波长与折射率的关系
Fig. 7. Experimental results. (a) Normalized transmission spectra of PCF-SPR sensors; (b) relationship between resonant wavelength and refractive index of PCF-SPR structure
4 结论
基于等离子体共振的光纤结构设计制造了一种新型的微流控芯片SPR传感器,体积为3.5cm×1cm×5cm,远小于传统的测量仪器。通过对基于表面等离子体共振的光子晶体光纤结构进行测试,在1.338~1.425的宽折射率测量范围内获得了高达8240.6nm/RIU的传感器灵敏度。该结构因其具有良好的折射率灵敏度,小巧的体积以及耐酸、抗腐蚀能力,将在生物化学领域具有更广阔的应用前景。
[1] 孙德藩, 刘鹏. 基于光纤光谱仪的溶液浓度测量系统[J]. 大学物理实验, 2019, 32(3): 82-85.
[2] 王旗. 基于光纤表面等离子共振传感器的制备及性能研究[D]. 南京: 南京邮电大学, 2019: 1- 3.
WangQ. The preparation and properties of optical fiber sensor based on surface plasmon resonance[D]. Nanjing: Nanjing University of Posts and Telecommunications, 2019: 1- 3.
[3] 赵静, 王英, 王义平. 氧化石墨烯增强型表面等离子体共振光纤传感器[J]. 激光与光电子学进展, 2019, 56(23): 230601.
[4] 高璐, 高文智, 罗政纯, 等. 面向海上溢油的SPR原理折射率检测实验系统[J]. 红外与激光工程, 2019, 48(8): 0813006.
[5] 蔡凯杰. 基于D型光纤的表面等离子体共振折射率传感器研究[D]. 南京: 南京信息工程大学, 2019: 9- 12.
Cai KJ. Research on surface plasmon resonance refractive index sensor based on D-type optical fiber[D]. Nanjing: Nanjing University of Information Science & Technology, 2019: 9- 12.
[6] 郭志勇, 葛益娴, 沈令闻, 等. 基于MSM结构的表面等离子体共振光纤折射率传感器[J]. 半导体光电, 2020, 41(2): 205-210.
[7] 李春赫, 马卓晨, 胡昕宇, 等. 微流控拉曼检测芯片的制备与应用[J]. 中国激光, 2021, 48(1): 0100001.
[8] 肖功利, 张开富, 杨宏艳, 等. D型对称双芯光子晶体光纤双谐振峰折射率传感器[J]. 光学学报, 2020, 40(12): 1206001.
[9] 刘海, 白冰冰, 张砚曾, 等. 基于SPR效应的金-PDMS涂覆光子晶体光纤高灵敏度温度测量[J]. 中国激光, 2020, 47(4): 0404003.
[10] 陈强华, 韩文远, 孔祥悦, 等. 基于光纤表面等离子体共振检测溶液折射率变化[J]. 中国激光, 2020, 47(8): 0804003.
[11] 郑一博. 多芯光子晶体光纤激光器及光子晶体光纤表面等离子体共振传感研究[D]. 天津: 天津大学, 2012: 74- 76.
Zheng YB. Research on multi-core photonic crystal fiber laser and surface plasmon resonance sensor with photonic crystal fiber[D]. Tianjin: Tianjin University, 2012: 74- 76.
[12] 王福成. 外层镀膜光子晶体光纤表面等离子体共振传感器的研究[D]. 秦皇岛: 燕山大学, 2019: 19- 22.
Wang FC. Study of outer coated photonic crystal fiber based surface plasmon resonance sensor[D]. Qinhuangdao: Yanshan University, 2019: 19- 22.
[13] 范振凯, 张子超, 王保柱, 等. 基于表面等离子体共振效应的光子晶体光纤折射率传感器的研究进展[J]. 激光与光电子学进展, 2019, 56(7): 070004.
Article Outline
李钢敏, 李致远, 李正冉, 王锦民, 夏历, 杨曌, 李微. 基于表面等离子体共振的高灵敏度光纤微流控芯片[J]. 中国激光, 2021, 48(1): 0106002. Gangmin Li, Zhiyuan Li, Zhengran Li, Jinmin Wang, Li Xia, Zhao Yang, Li Wei. High-Sensitivity Optical-Fiber Microfluidic Chip Based on Surface Plasmon Resonance[J]. Chinese Journal of Lasers, 2021, 48(1): 0106002.