光学超晶格中红外光参量振荡器研究进展
下载: 1804次特邀综述
Significance A 2--5 μm mid-infrared (MIR) laser has a broad application prospect and plays a significant role in applications such as remote sensing detection, atmospheric environmental monitoring, medical diagnosis, precision measurement, and photoelectric countermeasures. It covers the so-called “atmospheric window area”, that is, a transmission window with the maximum atmospheric transmittance. It has a strong penetrating ability for fog, smoke, and dust. Moreover, it has been widely used in the field of free-space optical communications. The spectral response range of infrared-guided missile detectors used in military applications is in the 2--5 μm band. As the applications of infrared detectors increase, the development of the corresponding interference technologies increases. A laser light source with a 2--5 μm band for photoelectric countermeasures against infrared seekers is urgently needed. The 2--5 μm band is also called the “molecular fingerprint area”, which covers most gas molecular absorption spectra. It finds important applications in the fields of air pollution monitoring, trace gas detection, precision spectral analysis, and molecular biomedicine. In addition, a 2--5 μm ultrastrong and ultrashort MIR laser can generate high-order harmonics, high-contrast attosecond light pulses, MIR frequency combs, and realize high brightness. It can also be used as an optical parametric oscillator (OPO) pump source for obtaining a 6--8 μm or even longer wavelength MIR laser. Therefore, because of the important application background and huge market demand of 2--5 μm MIR lasers, they have always been a desired topic of research in the field of all-solid-state laser technology.
Periodically-polarized optical superlattice crystals mainly consist of periodically-polarized lithium niobate (PPLN), periodically-polarized lithium tantalate, and periodically-polarized KTP. They possess the advantages of a large nonlinear coefficient, a wide tuning range, diversified wavelength tuning methods, and a compact structure. When applied to a MIR OPO, they can realize wide tuning, narrow linewidths, and high-power MIR lasers. An OPO based on an optical superlattice crystal is the most efficient way to generate MIR laser sources operating within the 2--5 μm wavelength range. We review the recent progress of optical-superlattice-based OPOs operating within the 2--5 μm wavelength band and analyse the structural features, advantages, and development prospects of OPOs operating in the continuous-wave, nanosecond, and picosecond regimes. The development tendency of optical-superlattice-based OPOs is also highlighted, indicating that high power, wide tunability, low power consumption, small size, and light weight are important development directions. Moreover, the optical superlattice crystals with high quality and large size, pump sources with better performances, and a reliable engineering designation are the key techniques for future development.
Progress The PPLN crystal was first prepared by applying the electric field polarization technique in 1993 by Yamada et al. In 1995, Myers et al. developed the room-temperature electric field polarization technique and effectively improved the size and quality of optical superlattice crystals. They realized the effective operation of a PPLN-based single-resonant OPO for the first time. Moreover, they obtained a tunable laser output ranging from 1.66 μm to 2.95 μm, which greatly promoted the development of the nonlinear frequency conversion technology, especially OPOs. At that point, the OPO based on optical superlattice crystals began to appear on the stage of history and shine. Operating mode of an OPO is determined by pump light, including a continuous-wave (CW) nanosecond, picosecond, and femtosecond. The current study introduces the research progress of the 2--5 μm MIR OPO based on optical superlattice crystals in a CW, nanosecond, and picosecond operation regime.
CW widely-tunable 2--5 μm MIR lasers have important applications in precision spectral analysis, optical sensing and detection, gas monitoring, free-space optical communications, and photoelectric countermeasures. Compared with other nonlinear optical crystals, optical-superlattice-based CW OPOs can not only effectively reduce the threshold, but also enhance the conversion efficiency and MIR output power. So far, the maximum output power, the narrowest linewidth of an optical-superlattice-based CW OPO is 71.6 W@2.907 μm and 1 kHz@2.7--4.2 μm. He et al. applied high-power single-frequency (linewidth of about 20 kHz) all-solid-state laser operation at 1064.2 nm as the pump source, and realized a broad tunable (1344.6--5103.2 nm), narrow-wavelength (~10 MHz) CW laser with a four-mirror ring cavity based on two PPLN crystals with periods from 25.5 μm to 32.0 μm and a period interval of 0.5 μm.
Compared with CW lasers, nanosecond pulsed lasers have much higher peak power and are easy to help achieve high-efficiency nonlinear frequency conversion. It is also the most widely studied operation mode since the invention of OPOs. Until now, the maximum output power and the widest tunable range of optical-superlattice-based nanosecond OPOs is 74.6 W@2.68 μm and 2128.6--5076.8 nm. He et al. used a high-power nanosecond 1064-nm laser as a pump source and a PPLN crystal with a thickness of 2 mm, a length of 50 mm, and a period of 32.0 μm. They achieved high-power and high-efficiency degeneracy point OPO with the output power of 33.3 W under the pump power of 60.9 W. The power instability RMS and the light-to-light conversion efficiency are 0.5% and 54.7%, respectively. The beam quality in the horizontal and vertical directions are 1.45 and 1.62, respectively. Using two multi-period PPLN crystals, 2--5 μm wide-tunable MIR OPO with a wavelength range from 2128.6 nm to 5076.8 nm was demonstrated using a nanosecond fiber laser as the pump source.
The 2--5 μm wide-tunable MIR picosecond laser has broad application prospects in laser ranging, Lidar, atomic and molecular dynamics, and time-domain spectroscopy. Compared with CW and nanosecond OPOs, picosecond OPOs need a synchronous pumping mechanism, which requires a fairly precise match of a pump pulse repetition rate and the round-trip frequency of an OPO resonator. Thus far, for the maximum output power of 7.1 W@2.1 μm, the widest tunable range of an optical-superlattice-based picosecond OPO is 2.7--5.3 μm. He et al. used a hybrid-slab amplifier to obtain a high-power picosecond laser with an idle frequency optical tuning range of 3362--4290 nm.
Conclusions and Prospects An OPO based on an optical superlattice crystal is the most efficient way to generate MIR laser sources operating within the 2--5 μm wavelength range. In this study, we mainly review the recent progress of optical-superlattice-based OPOs operating within the 2--5 μm wavelength band. We analyze the structural features, advantages and development prospects of OPOs operating in the CW, nanosecond, and picosecond regimes. The development tendency of optical-superlattice-based OPOs is also highlighted, indicating that high power, wide tunability, low power consumption, small size, and light weight are important development directions. Moreover, the optical superlattice crystals with high quality and large size, pump sources with better performances, and a reliable engineering designation are the key techniques for future development.
1 引言
表 1. 常用的中红外OPO非线性光学材料特性
Table 1. Characteristics of commonly mid-infrared OPO nonlinear optical materials
|
2~5 μm中红外激光在遥感探测、大气环境监测、医疗诊断、精密测量以及光电对抗等领域中具有广阔的应用前景和不可替代的重要作用[1-7]。3~5 μm中红外波段被称为“大气窗口区”,是大气透过率最高的传输窗口,在大雾、烟尘等中的穿透能力强,被广泛应用于自由空间光通信领域[8]。**上红外制导导弹探测器的光谱响应范围在2~5 μm波段,随着红外探测器应用量的逐年增加,相应的干扰技术也在加速开发,针对红外导引头的光电对抗技术迫切需要该波段的激光光源[9]。2 μm和3 μm附近的中红外激光位于水分子强吸收带内,在人体组织中的穿透深度浅,对周围组织的热损伤小,对组织具有较高的消融、切除和汽化能力;2 μm激光本身具有止血能力,被广泛应用于生物医疗领域;2~3 μm中红外激光还广泛应用于工业加工、激光雷达等领域。2~5 μm波段同时被称为“分子指纹区”,大量气体分子的吸收谱线位于该波段内,该波段激光在大气污染监测、微量气体探测、精密光谱分析和分子生物医疗等领域具有非常重要的应用价值[10]。此外,2~5 μm超强超短中红外激光可以产生高次谐波,进而实现高亮度、高对比度的阿秒光脉冲和中红外频率梳,也可作为光参量振荡器(OPO)泵浦源产生6~8 μm甚至更长波长的中红外激光[11]。因此, 2~5 μm中红外激光一直都是全固态激光领域的研究热点。
目前,2~5 μm全固态中红外激光器从产生机理上大致可以分为两类:一类是基于激光增益介质的受激辐射,主要包括半导体激光器、过渡金属或稀土离子掺杂的固体或光纤激光器等[12-19];另一类是基于非线性频率变换技术,主要包括中红外拉曼激光器、中红外超连续谱激光器、中红外差频激光器和中红外OPO等[20-30]。与其他技术方案相比,OPO具有输出功率高、转换效率高、光束质量好、调谐范围宽以及可实现多种运转方式(连续波、纳秒脉冲、皮秒脉冲甚至飞秒脉冲)等优点。特别是随着近年来近红外全固态激光技术(尤其是光纤激光器)的迅猛发展和大尺寸、高质量非线性光学晶体材料制备工艺技术的日益完备,OPO成为当前制备2~5 μm中红外激光光源最有效的技术手段。
用于中红外OPO的非线性光学晶体材料主要包括本征体块晶体和周期极化的光学超晶格两类。本征体块晶体主要包括磷酸钛氧钾(KTiOPO4,KTP)、砷酸钛氧钾(KTiOAsO4,KTA)、磷锗锌(ZnGeP2,ZGP)、硫镓银(AgGaS2)和硒镓银(AgGaSe2)等[31-39],晶体的主要特性如
本文简要介绍了OPO和准相位匹配技术原理,对基于光学超晶格的2~5 μm中红外OPO的国内外研究进展进行了总结,重点分析了连续波、纳秒脉冲和皮秒脉冲运转模式下光学超晶格中红外OPO的实现方式和结构特点,最后对光学超晶格中红外OPO的发展趋势进行了展望。
2 OPO和准相位匹配技术
OPO技术利用晶体的二阶非线性光学效应实现激光频率下转换,包括泵浦源、非线性参量晶体和谐振腔三部分。在满足相位匹配条件下,可以实现“任意”波长的参量光输出,并且入射的泵浦光能量均以光辐射(信号光和闲频光)的形式发射,没有废热产生。准相位匹配技术通过周期性地改变非线性晶体极化率值的符号来实现非线性效应的增强,它克服了双折射相位匹配技术空间走离效应导致的非线性作用长度有限、一般不能利用非线性晶体最大的有效非线性系数以及特定波长变换需对应晶体特定匹配角度等缺陷。
2.1 OPO的基本原理
根据初始和反馈条件不同,光参量效应可分为光参量发生(OPG)、光参量放大(OPA)和光参量振荡,三种作用示意图如

图 1. 光参量效应示意图。(a) OPG和OPA;(b) OPO
Fig. 1. Schematic of optical parametric effects. (a) OPG and OPA; (b) OPO
2.2 准相位匹配技术
为了克服折射率相位匹配的局限性,Armstrong 等[49]于1962年提出了准相位匹配(QPM)概念,即采用某种特定手段对非线性晶体的二阶极化率进行周期性调制以补偿光波相互作用过程产生的相位失配,最终实现高效率非线性频率变换。如果不考虑相位匹配,当入射强激光束在晶体中传播了一个相干长度Lc时,相互作用的光波之间会产生π的相位差,然后能流方向将会发生逆转,如

图 2. QPM过程。(a)光学超晶格中非线性频率变换光强度随相互作用长度的变化;(b)外电场周期极化后铌酸锂电畴的极化方向示意图
Fig. 2. Process of QPM. (a) Intensity of nonlinear frequency conversion light in optical superlattices versus interaction length; (b) schematic of polarization direction of lithium niobate domain after periodic polarization in external electric field
3 基于光学超晶格晶体的2~5 μm中红外OPO的研究进展
激光产生后不久,OPO的概念和理论模型在1963年由Korll[51]提出,随后Giordmaine等[52]于1965年首次在实验上进行了验证。之后由于缺乏高性能激光泵浦源特别是高质量非线性晶体,OPO的发展相对比较缓慢。直到20世纪80年代中后期,以KTP、LiB3O5(LBO)和β-BaB2O4(BBO)等为代表的一大批优质非线性光学晶体的出现为OPO的快速发展奠定了坚实的基础。Yamada等[53]于1993年通过外加电场极化的方法首次制备了周期极化的LiNbO3晶体;Myers等[54]于1995年在室温下对样品进行外加电场极化,大大降低了周期极化晶体的难度和成本,提高了周期极化晶体的质量和尺寸,并首次实现了基于QPM的单谐振OPO有效运转,获得了1.66~2.95 μm的调谐激光输出,极大地推动了非线性频率变换技术特别是OPO的发展。至此,基于光学超晶格晶体的OPO开始登上历史舞台并大放异彩。OPO参量光输出模式与泵浦光的工作模式相近,输出模式涵盖连续波到超短脉宽。本文主要介绍了连续波、纳秒脉冲和皮秒脉冲三种工作模式下基于光学超晶格晶体的2~5 μm中红外OPO的研究进展。
3.1 基于光学超晶格晶体的2~5 μm连续波中红外OPO的研究进展
2~5 μm连续波可调谐中红外激光在精密光谱分析、光学传感与探测、气体监测、自由空间光通信以及光电对抗等领域具有重用的应用价值。利用准相位匹配技术,泵浦光和参量光在整个晶体长度内都可以进行有效的参量耦合,晶体的最大有效非线性系数和长度可得到最大化利用。因此,与其他本征非线性光学晶体相比,基于光学超晶格的连续波OPO可以有效降低连续波OPO的振荡阈值,提高参量光的转化效率和输出功率,并可以通过改变周期和晶体温度来实现宽波段波长调谐,是产生2~5 μm连续波中红外激光的主要技术手段。
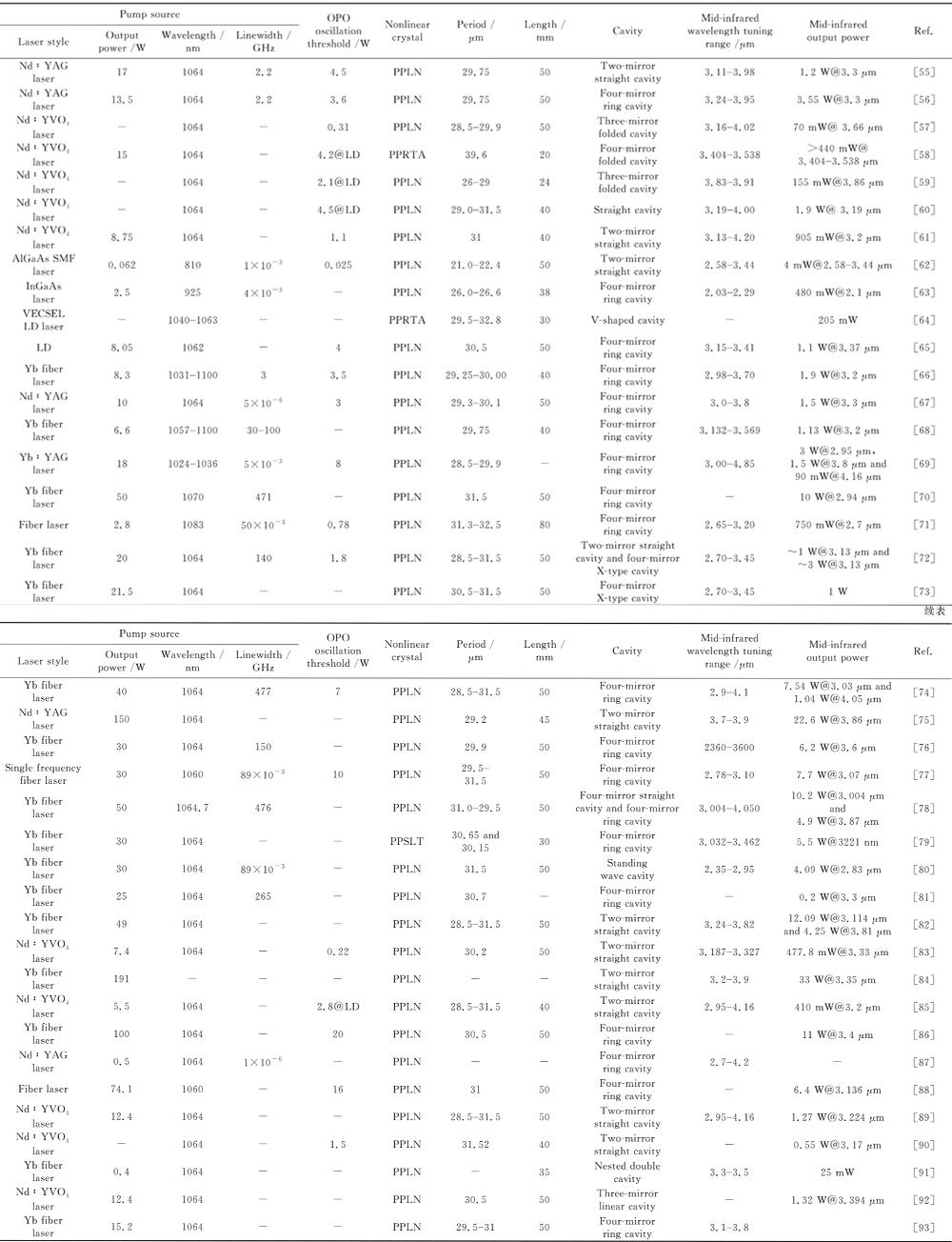
图 3. 基于光学超晶格晶体的2~5µm连续波中红外OPO的主要进展及性能参数
Fig. 3. Main progress and performance parameters of 2-5 µm CW mid-infrared OPOs based on optical superlattice crystals
![基于光学超晶格晶体的2~5 μm连续波中红外OPOs。(a)LD泵浦Nd∶YVO4内腔单谐振三镜L型复合腔OPO结构及(b)对应的3.66 μm闲频光的功率输出曲线[57];(c)LD泵浦Nd∶YVO4内腔单谐振两镜线形复合腔OPO结构及(d)闲频光的输出功率和波长调谐曲线[60];(e)VECSEL泵浦扇形PPLN内腔OPO结构及(f)闲频光输出功率曲线[64];(g)DFB光纤激光器泵浦窄线宽PPLN-OPO结构示意图及(h)基于该光源测试的CO2吸收谱线[71]](/richHtml/zgjg/2021/48/5/0501008/img_4.jpg)
图 4. 基于光学超晶格晶体的2~5 μm连续波中红外OPOs。(a)LD泵浦Nd∶YVO4内腔单谐振三镜L型复合腔OPO结构及(b)对应的3.66 μm闲频光的功率输出曲线[57];(c)LD泵浦Nd∶YVO4内腔单谐振两镜线形复合腔OPO结构及(d)闲频光的输出功率和波长调谐曲线[60];(e)VECSEL泵浦扇形PPLN内腔OPO结构及(f)闲频光输出功率曲线[64];(g)DFB光纤激光器泵浦窄线宽PPLN-OPO结构示意图及(h)基于该光源测试的CO2吸收谱线[71]
Fig. 4. 2--5 μm CW mid-infrared OPOs based on optical superlattice crystals. (a) LD-pumped Nd∶ YVO4 intracavity single resonant three-mirror L-type composite cavity OPO structure and (b) corresponding output power curves of 3.66 μm idle light [57]; (c) LD-pumped Nd∶ YVO4 intracavity single resonator two-mirror linear composite cavity OPO structure and (d) output power and wavelength tuning curves of idle light [60]; (e) VECSEL-pumped fan-shaped PPLN intracavity OPO structure and (f) output power curves of idle light [64]; (g) structural diagram of narrow-linewidth PPLN-OPO pumped by DFB fiber laser and (h) CO2 absorption spectral lines measured by it [71]
随着LD功率的不断提高,采用LD直接泵浦光学超晶格材料可以得到小型化中红外OPO。2001年,Lindsay等[62]采用810 nm的AlGaAs LD泵浦PPLN晶体,并采用泵浦增强单谐振结构,实现了2.58~3.44 μm的宽调谐连续波中红外激光输出,阈值只有25 mW左右。1999年,Klein等[63]采用InGaAs半导体激光器泵浦PPLN晶体,并采用四镜环型腔,实现了480 mW的2.1 μm 中红外激光输出,调谐范围为2.03~2.29 μm。2009年,Stothard等[64]采用VECSEL半导体激光器泵浦扇形PPLN晶体(周期为29.5~32.8 μm),OPO的实验装置示意图如
与全固态激光器和半导体激光器相比,光纤激光器具有结构灵活紧凑、可靠性高、环境适用性好、光束质量好和成本低等优点,基于光纤激光器泵浦的光学超晶格 OPO为新一代小型化高功率宽调谐中红外激光光源提供了新的发展思路。2002年,Gross等[66]利用Yb连续波激光器泵浦PPLN晶体,实现了1.9 W的连续波中红外激光输出。2005年, Chen等[70]采用50 W的Yb连续波光纤激光器泵浦PPLN晶体,获得了10 W的2.94 μm中红外激光输出。2010年,Henderson等[76]采用同样的结构获得了6.2 W的3.6 μm连续波中红外输出。Powers 等[77]报道了光纤激光泵浦连续波PPLN-OPO,在3070 nm中红外激光下输出功率达到7.7 W,光束质量<1.24。Zhao等[74]利用1064.7 nm的光纤激光器泵浦PPLN晶体,实现了2.9~4.1 μm宽调谐中红外激光输出,在3.03 μm处输出功率为7.54 W,在4.05 μm处输出功率为1.04 W。2013年,刘磊等[82]采用Yb光纤激光器泵浦PPLN晶体,获得了12.09 W的3.414 μm连续波激光输出,量子转换效率达到79.2%;在波长为3.81 μm时输出功率达到4.25 W,同时实现了3.24~3.82 μm宽调谐输出。2013年,许晓军等[84]采用光纤激光泵浦PPLN晶体,实现了3.2~3.9 μm宽调谐连续波激光输出,最高功率为34.2 W。
为了满足气体探测、精密光谱分析等对单频、宽调谐中红外连续激光的需求,人们开展了基于光学超晶格晶体的窄线宽连续波中红外OPO研究。由于非线性晶体的增益带宽较宽(nm级),在一般的谐振腔长度下,会有多个纵模同时振荡。因此,为了得到窄线宽连续波OPO输出,需要采取一定的措施对信号光或闲频光的线宽进行控制,一般采用四镜环形腔加标准具结构。van Herpen 等[67]采用单频连续波Nd∶YAG激光作为泵浦源,实现了3~5 μm宽调谐单频连续波中红外激光输出。为了满足气体探测对单频连续波中红外激光快速调谐输出的要求,Vainio等[73]采用单频连续可调的钛宝石激光作为泵浦源,实现了2.5~3.5 μm和3.4~4.4 μm范围的单频连续波中红外激光输出,频率扫描范围达到40 GHz。2006年,Henderson等[71]采用1083 nm的单频光纤激光器作为泵浦源,实现了2.65~3.20 μm波段单频连续波中红外激光输出,窄线宽OPO实验结构示意图如
山东大学何京良教授课题组采用高功率1064.2 nm全固态单频(线宽约为20 kHz)激光器作为泵浦源,采用四镜环型腔双谐振结构,实现了宽调谐窄线宽2~5 μm中红外激光输出[28],实验装置图如
![基于多周期PPLN的2~5 μm窄线宽连续波OPO[28]。(a) OPO实验结构示意图;(b)实验得到的最长闲频光波长的输出光谱;(c)窄线宽连续波OPO的波长调谐曲线;(d)泵浦功率为27.5 W时闲频光的输出功率和光子转换效率随波长的变化曲线;(e)采用延时自零差法测试得到的信号光线宽;(f) 3497.1 nm闲频光的输出功率为3.3 W时的光束质量](/richHtml/zgjg/2021/48/5/0501008/img_5.jpg)
图 5. 基于多周期PPLN的2~5 μm窄线宽连续波OPO[28]。(a) OPO实验结构示意图;(b)实验得到的最长闲频光波长的输出光谱;(c)窄线宽连续波OPO的波长调谐曲线;(d)泵浦功率为27.5 W时闲频光的输出功率和光子转换效率随波长的变化曲线;(e)采用延时自零差法测试得到的信号光线宽;(f) 3497.1 nm闲频光的输出功率为3.3 W时的光束质量
Fig. 5. 2--5 μm narrow-linewidth CW OPO based on multi-period PPLN[28]. (a) Experimental setup of OPO; (b) experimentally obtained output spectrum of idle light with longest wavelength; (c) wavelength tuning curves of narrow-linewidth CW OPO; (d) output power and photon conversion efficiency of idle light versus wavelength at pump power of 27.5 W; (e) linewidth of signal light measured by delay self-zero difference method; (f) beam quality of 3497.1 nm idle light at output power of 3.3 W
连续波的激光功率密度低,与体块本征非线性光学相比,光学超晶格晶体的有效非线性系数大,可利用的晶体长度长,因此在连续波OPO方面更具优势。目前,基于光学超晶格的2~5 μm 连续波OPO的最窄线宽为1 kHz@2.7~4.2 μm[87],最宽调谐范围为2128.4~5103.2 nm。连续波OPO可以有效避免脉冲激光泵浦OPO引起的光损伤等问题,但与脉冲OPO相比,功率密度低,因此转换效率相对较低。连续波OPO在精密光谱分析、气体探测等方面具有重要的应用价值,并且随着半导体激光器和光纤激光器的飞速发展,基于半导体激光直接泵浦和光纤激光泵浦的连续波光学超晶格OPO在输出功率、调谐范围、体积、功耗和重量等方面有着巨大的优势,已引起国内外研究人员的广泛关注。
3.2 基于光学超晶格晶体的2~5 μm纳秒中红外OPO的研究进展
纳秒脉冲中红外激光在遥感、医疗、**对抗和无线光通信等领域具有非常重要的应用价值。相比连续波激光,纳秒脉冲激光具有很高的峰值功率,易于实现高效率的非线性频率变换,得到了广泛的研究。相比于其他非线性参量晶体,基于光学超晶格晶体的纳秒OPO具有输出功率高、转换效率高、波长调谐范围宽、调谐方式简便等优点,成为高功率宽调谐纳秒中红外激光光源的主流发展方向。
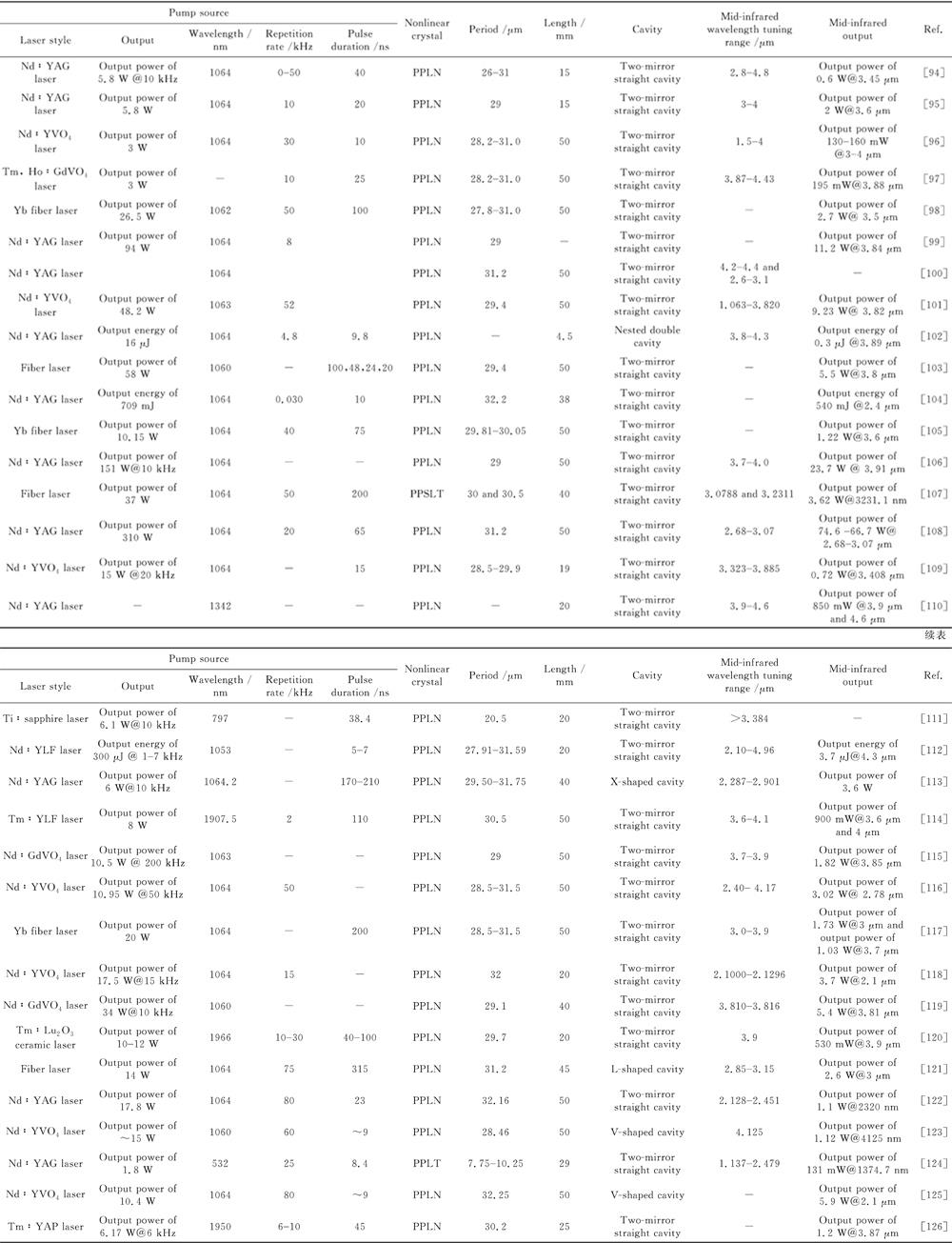
图 6. 基于光学超晶格的2~5µm纳秒中红外OPO的主要进展及性能参数
Fig. 6. Main progress and performance parameters of 2-5 µm ns mid-infrared OPOs based on optical superlattice crystals
1996年, Bosenberg等[94]利用具有25个极化周期(超晶格的周期为26~32 μm,间隔为0.25 μm)的PPLN晶体,实现了1.36~4.83 μm宽调谐输出。1997年,Myers等 [95]利用纳秒Nd∶YAG激光作为泵浦源,并采用单谐振结构,在3~4 μm范围内实现了大于3 W的调谐中红外激光输出。2004年,Th Bekman等[96]实现了波长调谐范围为3.7~4.0 μm的中红外激光输出,斜效率为15%。2007年,Zhang等[97]利用10.2 W的纳秒Nd∶YAG激光泵浦PPLT晶体,分别得到了1.0 W的4.03 μm和0.2 W的4.685 μm中红外激光输出。2009年,Alam等[98]采用光纤脉冲激光器泵浦PPLN,得到了2.7 W的3.5 μm中红外激光输出。彭跃峰等[99]采用1064 nm声光调Q的Nd∶YAG侧泵模块激光器泵浦PPLN晶体,为了避免晶体损伤,采用了椭圆形光斑,实现了16.7 W的3.84 μm中红外参量光输出,但是光束质量较差;到2010年,Wei等[100]又将中红外输出功率提升到22.6 W@3.86 μm,依然采用椭圆的泵浦光斑,但水平方向和竖直方向的光束质量因子分别提高到1.74和4.86。2010年, Wu等[101]利用半外腔结构的补偿热效应,在52 kHz重复频率下得到了9.2 W的3.8 μm中红外激光,光-光转换效率和斜效率分别为19.2%和29.4%,接近量子转换极限。2011年,Hardy等[102]采用Nd∶YAG被动调Q微片激光器作为泵浦源,利用嵌套双腔结构实现了3.8~4.3 μm单频脉冲中红外激光输出。2012年,Lin等[103]进一步提高泵浦源的功率,在58 W泵浦下获得了5.5 W的3.82 μm中红外激光输出。Ishizuki等[104]采用10 mm厚的PPLN晶体,得到了540 mJ的脉冲参量光输出,光-光转换效率达到76%。Wu等[105]采用纳秒光纤激光器泵浦多周期PPLN晶体,在3.43,3.63,3.72,3.83,3.99 μm处分别实现了4.7,4.3,4.1,3.3,2.1 W的中红外激光输出。Peng等[106]采用3 mm厚的PPLN晶体作为参量晶体,在泵浦功率为151 W时实现了27.4 W的3.91 μm中红外激光输出,斜效率为20.9%,光束质量因子<2.3,并且在3 μm处的平均输出功率高于40 W。2015年,赵刚等[107]利用光纤激光器泵浦双通道PPSLT晶体,有效避免了泵光能量的浪费,实现了四波长近-中红外参量光的同时输出,实验装置如
![基于光学超晶格晶体的2~5 μm纳秒中红外OPOs。(a)四波近-中红外OPO实验示意图和(b)对应的双周期OPO的波长调谐范围[107];(c)高功率 OPO+OPA实验结构示意图和(d)对应的OPA输出功率曲线[108]](/richHtml/zgjg/2021/48/5/0501008/img_7.jpg)
图 7. 基于光学超晶格晶体的2~5 μm纳秒中红外OPOs。(a)四波近-中红外OPO实验示意图和(b)对应的双周期OPO的波长调谐范围[107];(c)高功率 OPO+OPA实验结构示意图和(d)对应的OPA输出功率曲线[108]
Fig. 7. 2--5 μm ns mid-infrared OPOs based on optical superlattice crystals. (a) Experimental setup of four-wavelength near-mid-infrared OPO and (b) corresponding wavelength tuning range of double-period OPO[107]; (c) experimental setup of high power OPO+OPA and (d) corresponding output power curve of OPA[108]
除了采用成熟的1 μm激光作为泵浦源外, 2 μm激光泵浦PPLN-OPO可以使输出的信号光和闲频光均落在3~5 μm波段,大大提高中红外OPO的转换效率。2012年,Xu等[114]采用1.9 μm的Tm∶YLF激光器作为泵浦源,获得了900 mW的3~5 μm波段信号光和闲频光输出,总的光-光转换效率为31%。2020年,本课题组采用1.94 μm声光调Q的Tm∶YAP激光器泵浦PPLN,在泵浦功率为6.17 W时获得了1.2 W的3.87 μm中红外激光输出,光-光转换效率达到19.4%[126]。
山东大学何京良教授课题组采用高功率纳秒1064 nm激光器作为泵浦源,并采用2 mm厚的PPLN晶体,实现了高功率高效率的简并点OPO参量光输出[28],实验装置如
![基于2 mm厚的PPLN的高功率2.1 μm简并点纳秒OPO[28]。(a) OPO实验结构示意图;(b) OPO的输出功率曲线;(c) OPO的输出波长随温度和泵浦功率的变化;(d)最高输出功率下的光束质量;(e) 最高输出功率下5 h功率稳定性测试结果](/richHtml/zgjg/2021/48/5/0501008/img_8.jpg)
图 8. 基于2 mm厚的PPLN的高功率2.1 μm简并点纳秒OPO[28]。(a) OPO实验结构示意图;(b) OPO的输出功率曲线;(c) OPO的输出波长随温度和泵浦功率的变化;(d)最高输出功率下的光束质量;(e) 最高输出功率下5 h功率稳定性测试结果
Fig. 8. High power 2.1 μm degenerate point ns OPO based on 2 mm thick PPLN[28]. (a) Experimental setup of OPO; (b) output power curves of OPO; (c) output wavelength of OPO versus temperature and pump power; (d) beam quality at highest output power; (e) test result of power stability @5 h at maximum output power
采用纳秒光纤激光器作为泵浦源,并采用多周期PPLN晶体,实现了纳秒宽调谐中红外OPO输出,实验装置如
![基于多周期PPLN的2~5 μm纳秒宽调谐OPO[28]。(a)OPO的实验结构示意图;(b)OPO的波长调谐曲线;(c)泵浦功率为18 W时闲频光的输出功率随波长的变化曲线](/richHtml/zgjg/2021/48/5/0501008/img_9.jpg)
图 9. 基于多周期PPLN的2~5 μm纳秒宽调谐OPO[28]。(a)OPO的实验结构示意图;(b)OPO的波长调谐曲线;(c)泵浦功率为18 W时闲频光的输出功率随波长的变化曲线
Fig. 9. 2--5 μm wide-tuning ns OPO based on multi-period PPLN[28]. (a) Experimental setup of OPO; (b) wavelength-tuning curves of OPO; (c) output power of idle light versus wavelength at pump power of 18 W
采用纳秒激光泵浦源,可以有效提高OPO的转换效率,获得高功率中红外激光输出,该波段激光在遥感探测、医疗、**对抗等方面具有重要的应用价值。但是,纳秒脉冲激光的峰值功率高,要特别注意高功率运转情况下超晶格晶体材料的光损伤、热畸变和光束质量控制等关键问题。目前,基于光学超晶格晶体的2~5 μm纳秒OPO的最高输出功率达到74.6 W@2.68 μm(OPO+OPA结构)[108],最宽调谐范围为2128.6~5076.8 nm。高质量大尺寸(厚度)的光学超晶格晶体结合OPO+OPA结构的级联方案是实现高功率纳秒中红外输出的关键。随着光纤激光器的飞速发展,基于光纤激光泵浦的纳秒光学超晶格OPO有利于实现激光器的小型化、模块化和集成化,具有重要的科学意义和**应用价值。
3.3 基于光学超晶格晶体的2~5 μm皮秒中红外OPO的研究进展
2~5 μm宽调谐中红外皮秒激光在激光测距、激光雷达、原子分子动力学和时域光谱学等领域具有广阔的应用前景。同步泵浦光学超晶格OPO是产生中红外皮秒脉冲的有效手段,传统锁模激光器不能直接得到该波段脉冲。与连续波和纳秒OPO不同,皮秒脉冲的持续时间短,参量光在泵浦脉冲持续时间内无法在谐振腔中多次往返振荡,难以达到光参量振荡阈值。皮秒OPO基于同步泵浦原理,即OPO谐振腔腔长与泵浦激光器腔长匹配,保证参量光脉冲在OPO腔内往返一次的时间与泵浦光脉冲序列的重复周期相同,从而使参量光脉冲与入射到非线性晶体的泵浦光脉冲持续发生相互作用,参量光能量被不断放大。同步泵浦技术一般适用于较高重复频率场合下,低重复频率泵浦光下同步泵浦OPO的腔长太长,光学元件太多,不易实现参量光振荡。
由于高重复频率的连续波锁模皮秒脉冲激光器的峰值功率较低,因此早期的皮秒OPO大多采用振荡阈值较低的双谐振结构。近年来,随着皮秒脉冲激光的产生和放大技术的发展,基于光学超晶格晶体的单谐振OPO已得以实现,它代表了皮秒OPO最实用、最稳定的工作模式。1996年,Butterworth等[127]采用105 MHz、2.4 ps的Nd∶YLF激光泵浦PPLN晶体,获得了92 mW的2.37 μm中红外激光输出。1998年,Lefort等[128]采用120 MHz、4 ps的Nd∶YLF激光泵浦PPLN晶体,获得了2.7~5.3 μm宽调谐皮秒中红外激光输出,在5.3 μm处的输出功率约为20 mW,阈值仅为7.5 mW。Dearborn等[129]采用100 ps、76 MHz的Nd∶YAG激光脉冲光泵浦0.5 mm厚、12 mm长的PPLN晶体,实验装置如
![基于光学超晶格晶体的2~5 μm皮秒中红外OPO。(a)同步泵浦OPO实验示意图和(b)对应的功率输出曲线[129]; (c)驻波腔同步泵浦OPO实验示意图和(d)对应的输出功率曲线[134]](/richHtml/zgjg/2021/48/5/0501008/img_10.jpg)
图 10. 基于光学超晶格晶体的2~5 μm皮秒中红外OPO。(a)同步泵浦OPO实验示意图和(b)对应的功率输出曲线[129]; (c)驻波腔同步泵浦OPO实验示意图和(d)对应的输出功率曲线[134]
Fig. 10. 2--5 μm ps mid-infrared OPO based on optical superlattice crystal. (a) Experimental setup of synchronously-pumped OPO and (b) corresponding output power curves[129]; (c) experimental setup of synchronously-pumped OPO based on standing-wave cavity and (d) corresponding output power curves[134]
连续波锁模全固态激光振荡器的输出功率往往较低,导致全固态激光器泵浦皮秒OPO的输出功率较低。为了提高皮秒OPO的输出功率,需要对全固态锁模激光器产生的皮秒激光进行放大,以提高皮秒激光泵浦源的功率。2015年,山东大学何京良课题组利用混合腔板条放大器,得到了高功率皮秒激光[138],然后通过泵浦多周期PPLN晶体,实现了闲频光波长调谐范围为3362~4290 nm的中红外激光输出,并且在60%的波长调谐范围内,输出功率超过0.4 W。在此基础上,将OPO的腔长压缩为泵浦源腔长的1/2,信号光脉冲的重复频率从116.9 MHz增加到233.8 MHz, 在16 W泵浦下,获得了4.18 W的2896 nm OPO输出,对应的转换效率为32%。
皮秒脉冲激光的持续时间短,无法在谐振腔中往返多次而达到振荡阈值,这给皮秒OPO谐振腔的设计提出了更高的要求,即需要保证泵浦光脉冲时间和参量光脉冲时间交叠,实现同步泵浦。目前,基于光学超晶格晶体的2~5 μm皮秒OPO的最高输出功率为7.1 W@2.1 μm,最宽调谐范围为2.7~5.3 μm [136]。皮秒激光同步泵浦光学超晶格OPO产生的2~5 μm宽调谐中红外激光在医疗、光谱学、激光雷达、遥感探测等领域具有重要的应用价值,随着皮秒激光泵浦源功率的进一步提升,皮秒脉冲宽调谐中红外激光器的研制具有重要的科学意义和**价值。

图 11. 基于光学超晶格晶体的2~5µm皮秒中红外OPO的主要进展及性能参数
Fig. 11. Main progress and performance parameters of 2-5 µm ps mid-infrared OPOs based on optical superlattice crystals
4 总结与展望
2~5 μm中红外激光是重要的大气传输窗口,覆盖了多种气体分子的特征吸收峰,并包含多种红外探测器的响应波段,在**对抗、遥感探测、大气监测、空间光通信、生物工程等领域具有广泛的应用需求。OPO基于晶体的二阶非线性光学效应,实现了激光频率下转换,是实现高功率中红外激光光源的有效技术途径。非线性光学晶体是OPO的关键核心元件,以PPLN为代表的光学超晶格晶体具有非线性系数大、波长应用范围宽、作用距离长和使用灵活等特点,并可以通过改变温度和周期来实现宽调谐输出,是目前2~5 μm波段高功率宽调谐中红外OPO的最佳选择。OPO的泵浦源可以是连续波激光,也可是纳秒或皮秒脉冲激光,泵浦源参数直接决定了OPO的运转模式、振荡阈值、效率、输出功率、光束质量、稳定性以及应用范围。泵浦源可以是半导体激光器、半导体激光泵浦的全固态激光器以及光纤激光器。
基于光学超晶格晶体的OPO是产生高功率宽调谐2~5 μm中红外激光的有效技术手段。主要总结了近年来连续波、纳秒波和皮秒波运转模式下的光学超晶格OPO的研究进展, 2~5 μm高功率宽调谐中红外激光输出已得以实现。纵观国内外OPO的发展现状,提高输出功率、光束质量和稳定性以及实现低功耗、小型化和轻量化是基于光学超晶格晶体的2~5 μm中红外OPO未来发展的必然趋势,展望如下。
1) 大尺寸、高质量光学超晶格晶体是实现高功率OPO激光输出的关键。随着晶体生长技术和超晶格制备技术的不断发展,通过制备通光孔径更大(厚度>2 mm)、质量更高的光学超晶格晶体,并结合高功率的激光泵浦源,可以得到更高功率的中红外OPO输出。
2) OPO与OPA结合的技术方案是实现高功率中红外OPO运转的有效技术途径。光学超晶格晶体材料本身对长波长中红外激光的吸收会引起热效应,这会导致OPO转换效率降低、光束质量恶化、稳定性降低并限制输出功率的提高。采用OPO+OPA技术方案,可以在保证光束质量以及稳定性的情况下大大提高中红外激光的输出功率。
3)窄线宽连续波中红外激光在痕量气体探测和监测方面具有重要应用,可大大提高气体探测的灵敏度。通过快速调谐泵浦光和锁定信号光,可实现中红外波段闲频光的快速扫频激光输出,闲频光有望在中红外激光气体探测领域实现重要的应用。
4)设计新型的OPO谐振腔及控温和散热结构,缓解超晶格晶体中的热不稳定性,补偿热透镜效应,提高输出功率、光束质量和稳定性是光学超晶格OPO未来的研究重点;同时,更紧凑的谐振腔设计易于实现工程化,也是OPO未来发展的必然趋势。
5)针对中红外激光在**领域的特殊应用需求,半导体激光器和光纤激光器泵浦光学超晶格OPO是实现低功率、小型化和轻量化中红外激光光源的重要途经。
[1] 钟鸣, 任钢. 3~5 μm中红外激光对抗武器系统[J]. 四川兵工学报, 2007, 28(1): 3-6.
[3] 任国光, 黄裕年. 用激光红外干扰系统保护军用和民航机[J]. 激光与红外, 2006, 36(1): 1-6.
[5] Ebrahim-Zadeh M, Vodopyanov K. Mid-infrared coherent sources and applications: introduction[J]. Journal of the Optical Society of America B, 2016, 33(11): MIC1.
[6] 方聪, 王思博, 惠勇凌, 等. 掺铒中红外激光器的进展[J]. 激光与光电子学进展, 2019, 56(18): 180002.
[7] 令维军, 孙锐, 陈晨, 等. 基于反射式MoS2可饱和吸收体调Q锁模Tm: LuAG激光器[J]. 中国激光, 2019, 46(8): 0808002.
[9] 刘尊洋, 卞进田, 邵立, 等. 中红外激光技术研究进展[J]. 激光与红外, 2013, 43(8): 853-858.
Liu Z Y, Bian J T, Shao L, et al. Progress of mid-infrared laser technology[J]. Laser & Infrared, 2013, 43(8): 853-858.
[11] 任钢. 中红外光参量振荡器及其应用技术的研究[D]. 成都: 四川大学, 2006: 28- 36.
RenG. Studies on the mid-infrared optical parametric oscillators and their applications[D]. Chengdu: Sichuan University, 2006: 28- 36.
[12] Faist J, Capasso F, Sivco D L, et al. Quantum cascade laser[J]. Science, 1994, 264(5158): 553-556.
[14] Garbuzov D Z, Lee H, Khalfin V, et al. 2.3--2.7 μm room temperature CW operation of InGaAsSb-AlGaAsSb broad waveguide SCH-QW diode lasers[J]. IEEE Photonics Technology Letters, 1999, 11(7): 794-796.
[18] Jackson S D. Single-transverse-mode 2.5-W holmium-doped fluoride fiber laser operating at 2.86 μm[J]. Optics Letters, 2004, 29(4): 334-336.
[20] 张斌, 杨未强, 侯静, 等. 1.9~4.3 μm全光纤中红外超连续谱光源[J]. 中国激光, 2013, 40(11): 1102013.
[21] 殷科, 张斌, 蔡振, 等. 光纤抽运2.0~5.5 μm光谱平坦型中红外超连续谱光源[J]. 中国激光, 2016, 43(12): 1215001.
[22] 魏星斌. 中红外PPLN光参量振荡技术研究[D]. 北京: 中国工程物理研究院, 2010: 72- 77.
Wei XB. Study on mid-infrared PPLN optical parametric oscillation technology[D]. Beijing : China Academy of Engineering Physics, 2010: 72- 77.
[23] Savage N. Optical parametric oscillators[J]. Nature Photonics, 2010, 4(2): 124-125.
[24] 姚文明. 连续波光学参量振荡器及受激拉曼散射现象的研究[D]. 北京: 中国科学院, 2013.
Yao WM. Study on the continuous wave optical parametric oscillator and coexistent stimulated Raman scattering phenomenon[D]. Beijing: Chinese Academy of Sciences, 2013.
[25] 沈德元, 范滇元. 中红外激光器[M]. 北京: 国防工业出版社, 2015: 172- 177.
Shen DY, Fan DY. Mid-infrared lasers[M]. Beijing: National Defense Industry Press, 2015: 172- 177.
[26] 黄楠. 中红外及太赫兹波段的光学频率变换技术及应用[D]. 西安: 中国科学院大学, 2017.
HuangN, The technology and application of optical frequency conversion in the mid-infrared and terahertz band[D]. Xi’an:University of Chinese Academy of Sciences, 2017.
[27] 尚亚萍. 高功率光纤激光泵浦中红外光学参量振荡器研究[D]. 长沙: 国防科学技术大学, 2017.
Shang YP. High-power fiber lasers pumped mid-infrared optical parametric oscillator[D]. Changsha: National University of Defense Technology, 2017.
[28] 宁建. 光学超晶格中红外光参量振荡器研究[D]. 济南: 山东大学, 2017.
NingJ. Mid-IR optical parametric oscillator based on optical superlattice[D]. Jinan: Shandong University, 2017.
[29] 陈滔. 基于准相位匹配技术的高性能光参量振荡器研究[D]. 杭州: 浙江大学, 2014: 54- 59.
ChenT. Investigation on the advanced quasi-phase-matching optical parametric oscillators[D]. Hangzhou: Zhejiang University, 2014: 54- 59.
[30] 吴爽. 基于MgO: PPLN晶体的3.8 μm中红外光参量放大器研究[D]. 长春: 长春理工大学, 2020.
WuS. Study on 3.8 μm mid-IR optical parametric amplifier based on MgO: PPLN crystal[D]. Changchun: Changchun University of Science and Technology, 2020.
[31] Hansson G, Karlsson H, Wang S H, et al. Transmission measurements in KTP and isomorphic compounds[J]. Applied Optics, 2000, 39(27): 5058-5069.
[32] Bosenberg WR, Cheng LK, Bierlein JD. Optical parametric frequency conversion properties of KTiOAsO4 (KTA)[C] //Advanced Solid State Lasers, February 1,1993,New Orleans, Louisiana. Washington, D.C.: OSA, 1993: NF12.
[33] 张伟, 万勇, 陈鸿鸣, 等. 中红外脉冲KTA光参量振荡器实验研究[J]. 激光与红外, 2011, 41(7): 742-746.
[34] Vodopyanov K L, Ganikhanov F, Maffetone J P, et al. ZnGeP2 optical parametric oscillator with 3.8--12.4 μm tunability[J]. Optics Letters, 2000, 25(11): 841-843.
[36] 李充, 谢冀江, 潘其坤, 等. 中红外光学参量振荡器技术进展[J]. 中国光学, 2016, 9(6): 615-624.
[38] 贾宁, 王善朋, 陶绪堂. 中远红外非线性光学晶体研究进展[J]. 物理学报, 2018, 67(24): 244203.
[43] 王振友, 吴海信. 8~12 μm长波红外非线性晶体研究进展[J]. 人工晶体学报, 2019, 48(1): 34-46,53.
Wang Z Y, Wu H X. Research progress of nonlinear crystals for 8--12 μm long-wave IR generation[J]. Journal of Synthetic Crystals, 2019, 48(1): 34-46,53.
[46] 贾宁. LiInSe2单晶的生长与性能研究[D]. 济南: 山东大学, 2019.
JiaN. Study on the single crystal growth and properties of LiInSe2[D]. Jinan: Shandong University, 2019.
[47] 张国栋, 王善朋, 陶绪堂. 红外非线性光学晶体研究进展[J]. 人工晶体学报, 2012, 41(S1): 17-23.
[48] TiihonenM, PasiskeviciusV, LaurellF. Spectral and spatial limiting in idler-resonant optical parametric oscillator with PPKTP[C] //Advanced Solid-State Photonics 2004, February 1-4, 2004, Santa Fe, New Mexico, United States. Washington, D.C.:OSA, 2004: 456.
[49] Armstrong J A, Bloembergen N, Ducuing J, et al. Interactions between light waves in a nonlinear dielectric[J]. Physical Review, 1962, 127(6): 1918-1939.
[50] 李港. 激光频率的变换与扩展[M]. 北京: 科学出版社, 2005: 137.
LiG. Transformation and expansion of laser frequency[M]. Beijing: Science Press, 2005: 137.
[51] Kroll N M. Parametric amplification in spatially extended media and application to the design of tuneable oscillators at optical frequencies[J]. Proceedings of the IEEE, 1963, 51(1): 110-114.
[53] Yamada M, Nada N, Saitoh M, et al. First-order quasi-phase matched LiNbO3 waveguide periodically poled by applying an external field for efficient blue second-harmonic generation[J]. Applied Physics Letters, 1993, 62(5): 435-436.
[55] Bosenberg W R, Drobshoff A, Alexander J I, et al. Continuous-wave singly resonant optical parametric oscillator based on periodically poled LiNbO3[J]. Optics Letters, 1996, 21(10): 713-715.
[56] Bosenberg W R, Drobshoff A, Alexander J I, et al. 93% pump depletion, 3.5 W continuous-wave, singly resonant optical parametric oscillator[J]. Optics Letters, 1996, 21(17): 1336-1338.
[57] Stothard D J M, Ebrahimzadeh M, Dunn M H. Low-pump-threshold continuous-wave singly resonant optical parametric oscillator[J]. Optics Letters, 1998, 23(24): 1895-1897.
[58] Stothard D J M, -Y Fortin P, Carleton A, et al. Comparison of continuous-wave optical parametric oscillators based on periodically poled LiNbO3 and periodically poled RbTiOAsO4 pumped internal to a high-power Nd∶ YVO4 laser[J]. Journal of the Optical Society of America B, 2003, 20(10): 2102-2108.
[61] 檀慧明, 林洪沂, 张搏麟. 基于PPMgLN的中红外全固态可调谐光学参量振荡器[J]. 中国激光, 2010, 37(9): 2303-2308.
[63] Klein M E, Lee D H, Meyn J P, et al. Singly resonant continuous-wave optical parametric oscillator pumped by a diode laser[J]. Optics Letters, 1999, 24(16): 1142-1144.
[64] Stothard D J, Hopkins J M, Burns D, et al. Stable, continuous-wave, intracavity, optical parametric oscillator pumped by a semiconductor disk laser (VECSEL)[J]. Optics Express, 2009, 17(13): 10648-10658.
[65] Nieuwenhuis A F, Lee C J, Sumpf B, et al. One-Watt level mid-IR output, singly resonant, continuous-wave optical parametric oscillator pumped by a monolithic diode laser[J]. Optics Express, 2010, 18(11): 11123-11131.
[66] Gross P, Klein M E, Walde T, et al. Fiber-laser-pumped continuous-wave singly resonant optical parametric oscillator[J]. Optics Letters, 2002, 27(6): 418-420.
[67] van Herpen M, Te Lintel Hekkert S, Bisson S E, et al. Wide single-mode tuning of a 3.0--3.8 μm, 700-mW, continuous-wave Nd∶ YAG-pumped optical parametric oscillator based on periodically poled lithium niobate[J]. Optics Letters, 2002, 27(8): 640-642.
[68] Klein M E, Gross P, Boller K J, et al. Rapidly tunable continuous-wave optical parametric oscillator pumped by a fiber laser[J]. Optics Letters, 2003, 28(11): 920-922.
[70] Chen DW, Rose TS. Low noise 10-W CW OPO generation near 3 μm with MgO doped PPLN[C] //Conference on Lasers and Electro-Optics, May 22--27, 2005, Baltimore, MD, USA. New York: IEEE, 2005: 1829- 1831.
[71] Henderson A, Stafford R. Low threshold, singly-resonant CW OPO pumped by an all-fiber pump source[J]. Optics Express, 2006, 14(2): 767-772.
[72] Vainio M, Peltola J, Persijn S, et al. Singly resonant cw OPO with simple wavelength tuning[J]. Optics Express, 2008, 16(15): 11141-11146.
[73] Vainio M, Siltanen M, Peltola J, et al. Continuous-wave optical parametric oscillator tuned by a diffraction grating[J]. Optics Express, 2009, 17(9): 7702-7707.
[76] Henderson A, Tankala K, Esquinasi P . 23-watt 77% efficient CW OPO pumped by a fiber laser[J]. Proceedings of SPIE, 2010, 7580: 75800D.
[77] Powers P E, Kulp T J, Bisson S E. Continuous tuning of a continuous-wave periodically poled lithium niobate optical parametric oscillator by use of a fan-out grating design[J]. Optics Letters, 1998, 23(3): 159-161.
[78] 赵家群. 基于MgO:PPLN的中红外连续波光学参量振荡器技术的研究[D]. 哈尔滨: 哈尔滨工业大学, 2011: 137.
Zhao JQ. Study on mid-infared continuous wave optical parametric oscillator technique based on MgO: PPLN crystal[D]. Harbin: Harbin Institute of Technology, 2011: 137.
[82] 刘磊. 光纤激光泵浦的连续波光参量振荡器研究[D]. 长沙: 国防科学技术大学, 2013: 16- 23.
LiuL. Research of fiber laser pumped continuous-wave optical parametric oscillator[D]. Changsha: National University of Defense Technology, 2013: 16- 23.
[83] 姚文明, 檀慧明, 田玉冰, 等. 宽波段连续调谐全固态CW PPMgLN光学参量振荡器[J]. 强激光与粒子束, 2013, 25(8): 2021-2026.
[84] 许晓军, 李霄, 尚亚萍, 等. 光纤激光抽运34.2 W连续波中红外光学参量振荡器[J]. 中国激光, 2013, 40(8): 0809002.
[87] RicciardiI, MoscaS, ParisiM, et al. Sub-kHz-linewidth mid-infrared optical parametric oscillator[C] //2014 Conference on Lasers and Electro-Optics (CLEO) - Laser Science to Photonic Applications, June 8-13, 2014, San Jose, CA, USA. New York: IEEE, 2014: 14846534.
[90] 李宇昕, 李世凤, 居盼盼, 等. 高稳定紧凑型内腔连续光参量振荡器[J]. 红外与激光工程, 2017, 46(4): 0406004.
[92] 尉鹏飞, 张永昶, 张静, 等. 三镜直腔结构MgO:PPLN 高效连续光参量振荡器[J]. 光学精密工程, 2019, 27(1): 45-50.
[93] Peng Y F, Wei X B, Nie Z, et al. High-power, narrow-bandwidth mid-infrared PPMgLN optical parametric oscillator with a volume Bragg grating[J]. Optics Express, 2015, 23(24): 30827-30832.
[94] Bosenberg WR, DrobshoffA, Myers LE. High-power, high-repetition-rate optical parametric oscillator based on periodically-poled LiNbO3[C] //Advanced Solid State Lasers 1996, January 31,1996 ,San Francisco, California. Washington, D.C.: OSA, 1996: QM1.
[95] Myers L E, Bosenberg W R. Periodically poled lithium niobate and quasi-phase-matched optical parametric oscillators[J]. IEEE Journal of Quantum Electronics, 1997, 33(10): 1663-1672.
[96] Th Bekman H H P, van den Heuvel J C, van Putten F J M, et al. Development of a mid-infrared laser for study of infrared countermeasures techniques[J]. Proceedings of SPIE, 2004, 5615: 27-38.
[97] Zhang X B, Yao B Q, Ju Y L, et al. A 2.048-μm Tm,Ho:GdVO4 laser pumped doubly resonant optical parametric oscillator based on periodically poled lithium LiNbO3[J]. Chinese Physics Letters, 2007, 24(7): 1953-1954.
[98] Alam S U, Chen K K, Lin D J, et al. Externally modulated diode-seeded Yb 3+-doped fiber MOPA pumped high power optical parametric oscillator[J]. Proceedings of SPIE, 2009, 7197: 71970H.
[99] 彭跃峰, 王卫民, 谢刚, 等. 11.2 W中红外3.8 μm激光器[J]. 红外与激光工程, 2008, 37(S3): 82-85.
Peng Y F, Wang W M, Xie G, et al. 3.8 μm mid-infrared laser with 11.2 W output power[J]. Infrared and Laser Engineering, 2008, 37(S3): 82-85.
[101] Wu B, Kong J, Shen Y. High-efficiency semi-external-cavity-structured periodically poled MgLN-based optical parametric oscillator with output power exceeding 9.2 W at 3.82 μm[J]. Optics Letters, 2010, 35(8): 1118-1120.
[102] Hardy B, Berrou A, Guilbaud S, et al. Compact, single-frequency, doubly resonant optical parametric oscillator pumped in an achromatic phase-adapted double-pass geometry[J]. Optics Letters, 2011, 36(5): 678-680.
[107] 赵刚, 蒋旭东, 吕新杰, 等. 基于光学超晶格的四波长近-中红外光参量振荡器[J]. 中国激光, 2015, 42(5): 0502004.
[108] Peng Y, Wei X, Luo X, et al. High-power and widely tunable mid-infrared optical parametric amplification based on PPMgLN[J]. Optics Letters, 2016, 41(1): 49-51.
[110] Smith D E, Bowers M S, Schunemann P G. Multiwavelength mid-infrared zinc germanium phosphide and PPLN optical parametric oscillator[J]. Proceedings of SPIE, 2003, 4972: 78-81.
[111] Ding X, Zou L, Wen W Q, et al. All-solid-state quasi-continuous-wave tunable Ti: sapphire laser and pump-tuning PPLN-OPO[J]. Proceedings of SPIE, 2005, 5646: 400-403.
[113] Ghavami Sabouri S, Khorsandi A. Optical parametric oscillator pumped by relaxation oscillation pulses of a mechanically Q-switched Nd∶YAG laser[J]. Applied Physics B, 2013, 110(3): 425-431.
[114] Xu L, Zhang S Y, Chen W B. Tm: YLF laser-pumped periodically poled MgO-doped congruent LiNbO3 crystal optical parametric oscillators[J]. Optics Letters, 2012, 37(4): 743-745.
[117] Liu S D, Wang Z W, Zhang B T, et al. Wildly tunable, high-efficiency MgO: PPLN mid-IR optical parametric oscillator pumped by a Yb-fiber laser[J]. Chinese Physics Letters, 2014, 31(2): 024204.
[119] 李海速, 刘在洲, 郑建奎, 等. 高功率中红外MgO∶PPLN光参量振荡器[J]. 光学与光电技术, 2015, 13(1): 64-67.
[120] AntipovO, KositsyinR, Kal'Yanov D, et al. 3.9 μm optical parametric oscillator based on MgO∶PPLN pumped at 1966 nm using a high-repetition-rate Tm 3+: Lu2O3 ceramic laser [C] //The European Conference on Lasers and Electro-Optics 2017, June 25-29, 2017, Munich, Germany. New York: IEEE, 2017: CD_P_50.
[121] 邢廷伦, 王礼, 胡舒武, 等. 3 μm低阈值MgO∶PPLN-OPO布拉格体光栅腔谱宽压窄研究[J]. 中国激光, 2017, 44(1): 192-197.
Xing T L, Wang L, Hu S W, et al. Cavity-linewidth narrowing of 3 μm low threshold MgO∶PPLN-OPO by volume Bragg grating[J]. Chinese Journal of Lasers, 2017, 44(1): 192-197.
[122] Nandy B, Chaitanya Kumar S, Canals Casals J, et al. Tunable high-average-power optical parametric oscillators near 2 μm[J]. Journal of the Optical Society of America B, 2018, 35(12): C57.
[123] 王宇恒, 刘贺言, 王泽宇, 等. 4.1 μm高功率中红外内腔光参量振荡器[J]. 光子学报, 2019, 48(8): 0823002.
[127] Butterworth S D, Pruneri V, Hanna D C. Optical parametric oscillation in periodically poled lithium niobate based on continuous-wave synchronous pumping at 1.047 μm[J]. Optics Letters, 1996, 21(17): 1345-1347.
[128] Lefort L, Puech K, Butterworth S D, et al. Efficient, low-threshold synchronously-pumped parametric oscillation in periodically-poled lithium niobate over the 1.3 μm to 5.3 μm range[J]. Optics Communications, 1998, 152(1/2/3): 55-58.
[129] Dearborn M E, Koch K, Moore G T, et al. Greater than 100% photon-conversion efficiency from an optical parametric oscillator with intracavity difference-frequency mixing[J]. Optics Letters, 1998, 23(10): 759-761.
[130] Hoyt C W, Sheik-Bahae M, Ebrahimzadeh M. High-power picosecond optical parametric oscillator based on periodically poled lithium niobate[J]. Optics Letters, 2002, 27(17): 1543-1545.
[132] 赵刚, 居盼盼, 吕新杰, 等. 基于光学超晶格的皮秒光参量可调谐激光器[J]. 量子光学学报, 2014, 20(2): 172-176.
[133] Kokabee O, Esteban-Martin A, Ebrahim-Zadeh M. Efficient, high-power, ytterbium-fiber-laser-pumped picosecond optical parametric oscillator[J]. Optics Letters, 2010, 35(19): 3210-3212.
[134] Kienle F, Chen K K, Alam S U, et al. High-power, variable repetition rate, picosecond optical parametric oscillator pumped by an amplified gain-switched diode[J]. Optics Express, 2010, 18(8): 7602-7610.
[135] Chaitanya Kumar S, Wei J, Debray J, et al. High-power, widely tunable, room-temperature picosecond optical parametric oscillator based on cylindrical 5%MgO: PPLN[J]. Optics Letters, 2015, 40(16): 3897-3900.
[136] Chaitanya Kumar S, Ebrahim-Zadeh M. Yb-fiber-based, high-average-power, high-repetition-rate, picosecond source at 2.1 μm[J]. Laser & Photonics Reviews, 2016, 10(6): 970-977.
[139] Lamour T P, Kornaszewski L, Sun J H, et al. Yb: fiber-laser-pumped high-energy picosecond optical parametric oscillator[J]. Optics Express, 2009, 17(16): 14229-14234.
[140] KokabeeO, Esteban-MartinA, Ebrahim-ZadehM. High-power, fiber-laser-pumped picosecond optical parametric oscillator for the near- to mid-infrared[C] //Conference on Lasers and Electro-Optics 2010, May 16-21,2010,San Jose, California. Washington, D.C.: OSA, 2010: CThY1.
[141] 刘通, 汪晓波, 刘磊, 等. 基于周期极化掺镁铌酸锂晶体的中红外同步抽运皮秒光参量振荡器[J]. 中国激光, 2011, 38(12): 1202003.
[142] Kienle F, Lin D J, Alam S U, et al. Green-pumped, picosecond MgO: PPLN optical parametric oscillator[J]. Journal of the Optical Society of America B, 2011, 29(1): 144-152.
[145] Han K Z, Ning J, He J L, et al. High-efficiency mid-infrared picosecond MgO: PPLN single resonant optical parametric oscillator[J]. Chinese Physics Letters, 2015, 32(5): 054203.
[147] Nandy B, Chaitanya Kumar S, Ebrahim-Zadeh M. Phase-locked picosecond optical parametric oscillator[J]. Optics Letters, 2020, 45(14): 3981-3984.
Article Outline
聂鸿坤, 宁建, 张百涛, 杨克建, 赵刚, 吕新杰, 何京良. 光学超晶格中红外光参量振荡器研究进展[J]. 中国激光, 2021, 48(5): 0501008. Hongkun Nie, Jian Ning, Baitao Zhang, Kejian Yang, Gang Zhao, Xinjie Lü, Jingliang He. Recent Progress of Optical-Superlattice-Based Mid-Infrared Optical Parametric Oscillators[J]. Chinese Journal of Lasers, 2021, 48(5): 0501008.