百微焦飞秒光纤啁啾脉冲放大激光系统
下载: 1428次封面文章
报道了一套基于棒状光子晶体光纤放大器的高脉冲质量飞秒啁啾脉冲放大激光系统。该系统主要由锁模光纤振荡器、脉冲展宽器、脉冲选单单元、脉冲延时单元、掺镱光纤预放大器、棒状光子晶体光纤主放大器以及光栅压缩器组成。通过精细调节展宽器和压缩器中的光栅位置及入射角度优化系统的色散,当输出脉冲的重复频率为500 kHz时,获得了平均功率为51.5 W、单脉冲能量为103 μJ、脉冲宽度为251.7 fs的高脉冲质量高稳定性的飞秒激光输出。在1 MHz的重复频率下,获得了平均功率为61.5 W、脉冲宽度为273 fs的高脉冲质量飞秒激光输出;使用偏硼酸钡(BBO)晶体进行非线性频率变换,获得了平均功率超过20 W的517 nm绿光激光输出。
Objective In general, a single-mode polarization-maintaining fiber can serve as the stretcher and the Treacy-type grating pairs can serve as the compressor in the fiber chirped pulse amplification (FCPA) system. Although the positive group delay dispersion (GDD) introduced by the fiber stretcher can be compensated by the negative GDD introduced by the compressor, but the signs of the third-order dispersion (TOD) introduced by the stretcher and the compressor are the same, resulting in the deterioration of pulse quality due to the accumulation of TOD during the pulse amplification process. To solve the above problem, a segment of negative TOD fiber, high-order mode dispersion fiber or chirped fiber Bragg grating (CFBG) is usually introduced into the fiber stretcher to compensate the TOD in the system. However, the residual TOD in the system cannot be accurately measured, and the length of the dispersion fiber introduced in the stretcher can only be estimated. Therefore, several experiments have attempted to obtain the best dispersion compensation effect. However, these dispersion compensation methods are complicated and time-consuming. Here, we report an FCPA system that is composed of a mode-locked fiber oscillator, a pulse stretcher, a pulse picker, a pulse delay unit, an Yb-doped fiber preamplifier, a rod-shaped photonic-crystal fiber main amplifier, and a grating compressor. Among them, the pulse stretcher and compressor are based on block gratings. By finely adjusting the position and incidence angle of the gratings in the stretcher and compressor, a real-time dispersion compensation in the system is realized and a high-quality pulse is obtained. Compared with the other methods, it is a more convenient dispersion compensation method. However, in previous studies, the single-pulse energy of the CPA system with a rod-shaped photonic-crystal fiber served as the gain medium was generally lower than 100 μJ, and the pulse width was limited to 400 fs. To further reduce the pulse width and simultaneously obtain the hundred-microjoule chirped pulse-amplification laser system with high pulse-quality, further research works are done. Subsequently, a nonlinear frequency-doubled experiment is carried out with a BBO crystal.
Methods First, a set of home-made passive mode-locked fiber oscillator based on the nonlinear polarization evolution (NPE) principle was constructed. The output of the oscillator was directed to a Martinez-type pulse stretcher. The stretched pulse duration was measured to be approximately 1 ns. Then, the stretched pulse from the stretcher was amplified step by step through four ytterbium doped fiber preamplifier stages with the forward pumping scheme. Meanwhile, a fiber coupled acoustic optical modulator (AOM) was used to reduce the pulse repetition rate of the system from 62.8 MHz to 1 MHz@500 kHz. The output laser at the fourth fiber preamplifier stage was coupled to that at the main amplifier stage with the backward pumping scheme by means of space coupling. Then, to compress the laser pulse to the femtosecond level, the laser beam was directed to a Treacy-type pulse compressor. High pulse quality and stability femtosecond laser pulses were obtained by controlling the dispersion, which was optimized by finely adjusting the position and incidence angle of the gratings in the stretcher and compressor in the system. At the same time, the second harmonic generation frequency resolution optical gate (SHG-FROG) was used to observe the pulse duration and phase information online. In addition, a nonlinear frequency-doubled experiment was carried out with a BBO crystal. A half wavelength plate (HWP) placed before the BBO crystal was used to adjust the polarization state of the fundamental laser beam. Simultaneously, the phase-matching condition between the fundamental and the frequency-doubled lasers was optimized to obtain a high average power of green laser.
Results and Discussions The mode-locked ytterbium (Yb)-doped fiber oscillator that was designed to have a repetition rate of 62.8 MHz delivered pulses with an average output power of 12 mW, the central wavelength of 1031 nm, and a bandwidth of 15.8 nm (Fig. 2). The output of the oscillator was directed to a Martinez-type pulse stretcher. The stretched pulse duration was measured to be approximately 1 ns (Fig. 4). Owing to the losses from diffraction gratings and multiple reflections on the mirrors, the average power and spectral bandwidth of the stretcher decreased to 2.87 mW and 13.5 nm, respectively (Fig. 2). Then, to ensure the spectral quality, the average power of the stretcher was enhanced step by step through four ytterbium-doped fiber preamplifier stages with the forward pumping scheme and a main amplifier with the backward pumping scheme (Fig. 2). Device parameters for different stages in the system were listed in detail (Table 1). To avoid excessive gain narrowing, the average power of the fourth fiber preamplifier stage was limited to 0.86 W at a pulse repetition rate of 500 kHz and 2.0 W at a pulse repetition rate of 1 MHz, respectively. The optical spectra were recorded after different amplification stages. Only minor gain narrowing effect was visible (Fig. 2). This could be attributed to the distribution of the amplification gain over multiple stages of the amplifiers. With the increase of pumping power, the average powers of the main amplification and compressor were recorded (Fig. 5). The stability of the system was studied to meet the long-term test requirements of some special experiments. During the process of the 2.5 h continuous test, the laser operated normally, the output average power maintained high stability, and the root mean square(RMS) of the power is 0.31%(61.23 W@1 MHz) or 0.21%(50.4 W@500 kHz) (Fig. 6). The beam quality was measured at the average power of 50 W and a pulse repetition rate of 1 MHz in the system, showing a Gaussian distribution of near-field with M2=1.22 (Fig. 6). The pulse duration was characterized by SHG-FROG. At a repetition rate of 1 MHz in the system, a high-quality femtosecond laser pulse with an average power of 61.5 W and pulse duration of 273 fs was obtained (Fig. 7). A nonlinear frequency-doubled experiment was carried out with a BBO crystal, and more than 20 W average green light power at 517 nm was obtained (Fig. 8).
Conclusions The FCPA laser system based on transmission diffraction gratings serving as stretcher and compressor, was experimentally studied. By controlling and optimizing the spectral shape of the seed source, the stretcher and all stages of fiber pre-amplifiers without distortion are used to ensure that the spectral shape of the main amplifier was smooth and the distortion of spectral shape after de-chirped was avoided. To obtain a high-quality pulse, real-time compensation of the dispersion of the system was conducted by finely adjusting the position and incidence angle of the gratings in the stretcher and compressor. Finally, the high pulse quality and stability femtosecond laser with pulse duration of 251.7 fs, average power of 51.5 W, and 103 μJ of energy per pulse at repetition rate of 500 kHz was obtained. At a repetition rate of 1 MHz in the system, a high quality femtosecond laser pulse with average power of 61.5 W and pulse duration of 273 fs was obtained. By carrying out the nonlinear frequency-doubled experiment with a BBO crystal, a green laser with average power of over 20 W at 517 nm was generated. The CPA device will be applied in femtosecond laser processing, medical treatment, national defense, and other fields. The UV light source prepared by this system can be used in photoelectron spectroscopy experiments and has important practical value in the field of material surface science research.
1 引言
随着激光器件及技术的不断发展,高平均功率、高峰值功率的超快飞秒激光器逐渐成为研究热点,被广泛应用于精密加工[1-2]、基于高次谐波技术的二次光源开发及应用[3]、临床医疗[4-6]、超快生物医学光子学[7]、气相化学[8]、超快光谱学[9]以及****应用[10]等领域。与传统的固体激光器相比,超快光纤激光器由于具有操作方便、集成度高、稳定性好、抗干扰能力强、散热性能出色等优点而备受青睐。
在高功率飞秒光纤激光器中,由于光纤中的脉冲在被直接放大时受到受激拉曼散射、受激布里渊散射、自相位调制、交叉相位调制等多种非线性效应的影响,峰值功率的提升受到限制[11]。为了减小非线性效应,提高飞秒激光脉冲的峰值功率,主要采取以下两种技术方案:在时间上,通过啁啾脉冲放大(CPA)技术降低光纤内脉冲的峰值功率[12-13];在空间上,通过使用大模场面积的光子晶体光纤或者双包层光纤,降低非线性效应,提高脉冲峰值功率[14]。在高平均功率高峰值功率飞秒光纤放大器方面,德国科学家走在了世界的前列。2018年, Müller等[15]使用4路掺镱光纤放大器进行相干光束合成,获得了平均功率为3.5 kW、脉宽为430 fs、重复频率为80 MHz的激光输出。2020年,Müller等[16]又使用12路掺镱光纤放大器进行相干光束合成,获得了平均功率为10.4 kW、脉宽为254 fs、重复频率为80 MHz的激光输出。然而,我们注意到,在多路相干合成技术中,单路放大技术是非常重要的一项单元技术。因此,研究高功率、高脉冲能量的光纤啁啾脉冲放大激光系统具有重要的技术价值。
目前,高功率的光纤啁啾脉冲放大(FCPA)系统大多数以光子晶体光纤(PCF)作为增益介质,并且取得了一系列的研究成果。2007年,德国的Röser等[17]使用光子晶体光纤获得了重复频率为900 kHz、平均功率为90 W、单脉冲能量为100 μJ,脉冲宽度为500 fs的激光输出。2011年,该课题组利用CPA技术结合非线性气体压缩的方法获得了重复频率为1 MHz、单脉冲能量为93 μJ、脉冲宽度为亚百飞秒的激光输出[18]。2013年,美国的Wan等[19]使用单通棒状光子晶体光纤作为主放大级,获得了重复频率为100 kHz、单脉冲能量为0.85 mJ、脉冲宽度为705 fs的激光输出。2015年,美国的Kim等[20]使用大模场、高增益的掺镱光纤作为放大级,获得了平均功率为25 W、重复频率为400 kHz、单脉冲能量为62 μJ、脉冲宽度为亚400 fs的激光输出。2016年, Zhao[21]等使用棒状光子晶体光纤作为主放大器,获得了重复频率为1 MHz、脉冲宽度为270 fs、单脉冲能量为100 μJ的激光输出。2019年, Manchee等[22]使用棒状光子晶体光纤作为主放大器,获得了放大后重复频率为500 kHz、平均功率为100 W的激光输出。国内在该领域的研究起步较晚,与国外还存在一定的差距。2018年,中国工程物理研究院的向祥军等[23]采用棒状光子晶体光纤作为主放大器,啁啾体布拉格光栅(CVBG)作为脉冲压缩器,获得了重复频率为500 kHz、平均功率为104 W、脉冲宽度为767 fs,光束质量为1.56的激光输出。2018年,西安电子科技大学的Yang等[24]获得了重复频率为1 MHz、单脉冲能量为32 μJ的激光输出;在32 W的输出功率下使用自相关仪测得脉冲宽度为153 fs,但是由于脉冲中含有未完全补偿的残余色散,自相关函数曲线具有较高的基座。2018年,上海理工大学的周锋全等[25]采用大模场掺镱光子晶体光纤作为主放大级,获得了重复频率为300 kHz、单脉冲能量为4.5 μJ、脉冲宽度为202 fs的激光输出。2020年,北京工业大学的Li等[26]使用非线性放大环形镜技术(NALM)搭建锁模种子源,以棒状光子晶体光纤作为主放大级,获得了重复频率为4.2 MHz、平均功率为51 W、单脉冲能量为12.1 μJ、脉冲宽度为14 ps的激光输出。2020年,中国科学院的Bai等[27]使用含有块状光栅基的展宽器和压缩器,以棒状光子晶体光纤作为主放大器,获得重复频率为500 kHz、平均功率为33.2 W、脉冲宽度为272 fs、单脉冲能量为66.4 μJ的激光输出。2020年,中国科学院物理所的Zhang等[28]以棒状光子晶体光纤作为主放大器,高色散啁啾镜作为脉冲压缩器,获得了重复频率为50 MHz、平均功率为101.2 W、单脉冲能量约为2 μJ、脉冲宽度为47 fs的激光输出。
在上述工作中,以棒状光子晶体光纤作为主放大级的CPA系统,其输出激光的单脉冲能量普遍低于100 μJ[18,20,24-28],脉冲宽度限制在400 fs[17,19-20,23,26]。虽然Röser等[17,19,23]获得了超过100 μJ的单脉冲能量,但是未获得较窄的脉冲宽度。为了进一步降低脉冲宽度,同时获得百微焦量级、高脉冲质量的啁啾脉冲放大激光系统,本课题组进行了更加深入的研究。
一般而言,FCPA系统采用单模保偏光纤作为展宽器,Treacy光栅对作为压缩器。尽管光纤展宽器引入的正的二阶色散(GDD)可由压缩器引入的负GDD补偿,但是展宽器和压缩器引入的三阶色散(TOD)的符号相同,在脉冲放大过程中TOD的累积导致脉冲质量劣化[29]。为了解决上述问题,通常在光纤展宽器中引入一段负三阶色散的光纤[30]、高阶模色散光纤[31]或者使用啁啾光纤布拉格光栅(CFBG)[23,32],用来补偿系统中的三阶色散。在上述方案中,系统中残余的三阶色散无法得到精确测量,引入的色散光纤也只能给出估算长度,研究者只能在实验中通过多次尝试以获得最佳的色散补偿效果。三阶色散补偿的调节自由度低,耗时费力。相比上述方案,采用块状光栅基脉冲展宽器和压缩器的CPA系统,补偿色散时调节自由度更高。在理想情况下,当展宽器和压缩器使用相同的光栅和相同的入射角度时,全部的色散阶都可以完全匹配[33]。即使在放大系统中,由于脉冲经过增益介质和其他的光学元件后会引入额外的材料色散,也可以通过精细调节展宽器和压缩器中的光栅位置和入射角优化系统的色散,获得较高质量的脉冲输出。
综合以上考虑,本文报道了一种采用块状透射式光栅的Martinez型双通展宽器[34]和Treacy型双通压缩器[35]作为色散调控器件,以大模场直径的棒状PCF作为主放大器的FCPA系统。当系统输出脉冲的重复频率为500 kHz时,实验获得了平均功率为51.5 W、单脉冲能量为103 μJ的高脉冲质量、高稳定性的飞秒激光输出。在1 MHz的重复频率下,获得了平均功率为61.5 W、脉冲宽度为273 fs的高脉冲质量飞秒激光输出;使用偏硼酸钡(BBO)晶体进行二倍频实验,获得了超过20 W的绿光激光输出。
2 实验装置
该套CPA系统由光纤锁模振荡器、脉冲展宽器、脉冲选单单元、脉冲延时单元、四级光纤预放大器、主放大器和脉冲压缩器组成,实验装置结构如
表 1. 系统中不同阶段的器件参数
Table 1. Device parameters at different stages in system
|
使用非线性偏振旋转(NPE)技术搭建环形腔光纤锁模振荡器,其中心波长为1031 nm,重复频率为62.8 MHz,高斯型光谱半峰全宽为15.8 nm。将光纤锁模振荡器产生的锁模激光注入到展宽器中进行脉冲展宽。实验使用的展宽器为基于单光栅的折叠式Martinez型脉冲展宽器。脉冲展宽器主要由透射式衍射光栅、透镜、角反射镜和端面反射镜组成。使用线偏振输出的激光源,旋转其偏振方向,使其与光栅刻线方向(TE)相同,获得了最大97.5%的衍射效率。随后,将展宽器输出的脉冲激光逐级通过四个正向泵浦抽运的掺镱光纤预放大级进行功率放大。一级光纤放大器采用一段长度为37 cm、纤芯和包层直径分别为6 μm和125 μm的单包层掺镱光纤作为增益介质。泵浦源采用单模光纤输出的半导体碟形泵浦源,中心波长为976 nm,最高输出功率为500 mW。第二级光纤放大器采用长度为190 cm的掺镱保偏双包层光纤(DCF)作为增益介质,纤芯直径为10 μm,第一包层直径为125 μm。泵浦源采用多模光纤输出的半导体泵浦源,光纤纤芯直径为105 μm,最高输出功率为9 W@976 nm。通过(2+1)×1保偏光纤合束器,泵浦光被耦合到双包层增益光纤的第一包层中,信号光被耦合到双包层增益光纤的纤芯中。为了消除包层的残余泵浦光,在双包层增益光纤后熔接一个匹配的泵浦剥离器。为了隔离反向回光、避免信号串扰并降低放大器相关器件损坏的可能性,各级光纤放大器之间均熔接了与之匹配的保偏光纤隔离器。为了降低激光器的重复频率,在二级光纤放大器之后熔接了光纤耦合的声光调制器(AOM)。AOM的驱动器由种子源输出的信号进行触发。在驱动器被触发前,采用光电探测器探测种子源信号,并通过分频器和数字延时发生器获得同步信号输出。为了弥补声光调制器降频带来的功率损失,采用第三级光纤放大器进一步提高激光器的输出功率。第三级光纤放大器使用长度为130 cm的掺镱保偏单模光纤作为增益介质,纤芯直径为6 μm,包层直径为125 μm。泵浦源采用单模光纤输出的半导体碟形泵浦源,中心波长为976 nm,最高输出功率为500 mW。第四级光纤放大器与第二级光纤放大器的结构相同,使用纤芯直径为10 μm、第一包层直径为125 μm、长度为60 cm的掺镱保偏双包层光纤作为增益介质。
为了进一步放大功率,最后一级主放大器采用长度为80 cm的掺镱棒状光子晶体光纤模块。该光子晶体光纤为双包层结构,其外径为1 mm,内包层直径为261 μm,纤芯直径为85 μm,模场直径为65 μm,模场面积为3300 μm2@1040 nm,泵浦光的数值孔径(NA)为0.58@950 nm,吸收系数为15 dB@976 nm。为了保证棒状光纤的正常运转,需要外加水冷保持其工作温度。主放大级采用后向泵浦的方式进行泵浦,泵浦源为多模光纤输出的半导体激光器,光纤纤芯直径为200 μm,数值孔径为0.22,最大输出功率为163 W@976 nm。在主放大之前放置半波片以调节入射激光的偏振态方向。利用由刻线密度为1740 line/mm的透射式衍射光栅对构成的双通Treacy型压缩器对主放大器输出的脉冲进行压缩。为了直观了解该套实验装置,
为了获得倍频激光,在CPA系统的输出端使用BBO(β-BaB2O4,8 mm×8 mm×1 mm)晶体进行频率变换实验。基频光经过焦距为250 mm的透镜会聚进入BBO晶体。同时,使用半波片调整入射到晶体内的光束的偏振方向。精细调节晶体的位置及角度以获得高效率的倍频激光输出。
3 实验结果与分析
为了保证在每一级的放大过程中有较高的光束质量,避免脉冲和光谱的畸变,高质量种子源至关重要。
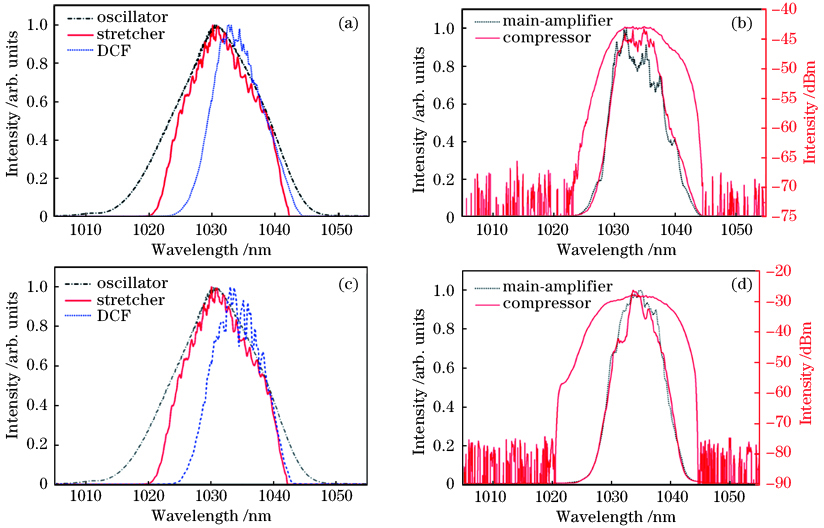
图 2. 系统中信号光在不同阶段的光谱。(a)信号光经过振荡器、展宽器、四级光纤放大器后的光谱(脉冲重复频率为500 kHz);(b)信号光经过主放大器、压缩器后的光谱(脉冲重复频率为500 kHz);(c)信号光经过振荡器、展宽器、四级光纤放大器后的光谱(脉冲重复频率为1 MHz);(d)信号光经过主放大器、压缩器后的光谱(脉冲重复频率为 1 MHz)
Fig. 2. Spectrum of signal light at each stage in system. (a) Spectra after oscillator, stretcher, and DCF(pulse repetition frequency: 500 kHz); (b) spectra after main-amplifier and compressor(pulse repetition frequency: 500 kHz); (c) spectra after oscillator, stretcher, and DCF(pulse repetition frequency: 1 MHz); (d) spectra after main-amplifier and compressor(pulse repetition frequency: 1 MHz)
将掺镱光纤锁模振荡器产生的激光脉冲注入到双通Martinez型脉冲展宽器中进行脉冲展宽。经过展宽器后,输出脉冲的平均功率为2.87 mW,输出光谱的半峰全宽为13.5 nm,如

图 3. 信号光在无折叠Martinez型脉冲展宽器中不同位置处的光束尺寸
Fig. 3. Beam diameter of signal light at each position in un-folded Martinez-type pulse stretcher
脉冲经过双通折叠型Martinez脉冲展宽器后的时域展宽量[36]为
式中:f为透镜的焦距;x为透镜和光栅之间的距离;m为衍射级次;λ为中心波长;Δλ为光谱的半峰全宽;c为光速;d为光栅的刻线密度;θ为中心波长对应的衍射角。
通过计算可知,经过展宽器后的锁模脉冲宽度为970 ps。使用带宽为16 GHz、采样率为50×109 sampling/s的高速示波器配合12 GHz的光电探测器测得经过光纤预放大器后的脉冲宽度为1.1 ns,如

图 4. 信号光经过振荡器和预放大器后的锁模脉冲宽度
Fig. 4. Mode-locked pulse width after oscillator or pre-amplifier
随后,展宽器输出的信号光经过各级光纤预放大器进行逐级功率提升。通过第一级光纤放大器后,在200 mW的泵浦功率下获得了平均功率为12 mW的激光输出。通过第二级光纤放大器后,当泵浦功率为1.05 W时,获得了150 mW的激光输出。在声光调制器之后获得了平均功率为0.5 mW、重复频率为500 kHz的激光输出。为了弥补声光调制器的功率损失,采用第三级光纤放大器进一步提高信号光的输出功率。第三级光纤放大后,信号光的平均功率提高到20 mW。在第四级光纤放大过程中,为了避免严重的增益脉冲窄化现象的发生,泵浦功率没有设置为最大值。当泵浦功率为4 W时,获得了0.86 W的激光输出。
主放大之前的光谱形状对于放大后的光谱质量至关重要。系统中各阶段所对应的光谱如
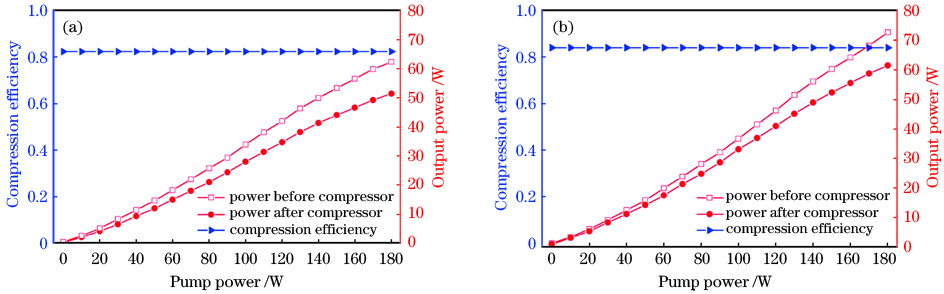
图 5. CPA系统的主放大器、压缩器的输出功率和压缩效率。(a) 脉冲重复频率为500 kHz; (b) 脉冲重复频率为1 MHz
Fig. 5. Output powers of main-amplifier and compressor in CPA system as well as compression efficiencies. (a) Pulse repetition frequency is 500 kHz; (b) pulse repetition frequency is 1 MHz
为了研究系统在不同重复频率下的工作状态,通过调整脉冲选单单元,使系统输出脉冲的重复频率变为1 MHz。在保证前三级光纤预放大器的参数不变的情况下,当四级光纤放大器的泵浦功率为9 W时,获得了2.0 W的激光输出。在主放大器的最高泵浦功率下获得了72.8 W的平均功率,压缩后最大61.5 W的激光输出,如
当系统输出脉冲的重复频率为500 kHz时,主放大器和压缩器的输出光谱如
为了满足某些实验的长时间测试需求,并保证激光器的安全运行,在室温(22 ℃)条件下分别对工作在500 kHz和1 MHz两种重复频率下的激光器稳定性进行测试,结果如

图 6. CPA系统的功率稳定性(插图为光束质量)
Fig. 6. Power stability of CPA system (beam quality shown in inset)
FCPA系统的色散控制也是实现高质量脉冲输出的重要因素。在实验中,输入到展宽器的光谱中心波长为1031 nm,以利特罗(Littrow)角作为入射角,此时展宽器引入的GDD为43.25 ps2,TOD为-0.615 ps3。在放大系统之后,与展宽器输出的光谱相比,光谱的中心波长红移到1034 nm。所以,在脉冲压缩阶段,使用与展宽器相同的光栅入射角度,压缩器引入的GDD为-47.35 ps2,TOD为0.715 ps3,压缩后的脉冲必定会产生残余色散。为了消除残余色散,获得高质量的脉冲输出,通过精细调节展宽器和压缩器中光栅的入射角度,优化压缩器中光栅对的间距,同时使用二次谐波频率分辨光学门(SHG-FROG)在线观测输出脉冲的脉冲宽度和相位信息,最终获得了具有较高光束质量的脉冲输出。
当系统输出脉冲的重复频率为1 MHz、平均功率为50 W时,二次谐波频率分辨光学门测得的系统输出的脉冲宽度为273.6 fs,如
为了获得二倍频激光,当CPA系统输出脉冲的重复频率为1 MHz时,使用BBO晶体进行倍频实验。为了获得更高的倍频激光转化效率,使用焦距为250 mm的透镜对入射到晶体内的基频激光进行光束整形。同时,使用半波片调整入射到晶体内的光束的偏振方向。精细调节晶体的位置及角度,使其满足倍频的I类相位匹配条件(相位匹配角为23.2°)。倍频激光的输出功率和二次谐波(SHG)效率随着红外(IR)激光输入功率的变化规律如
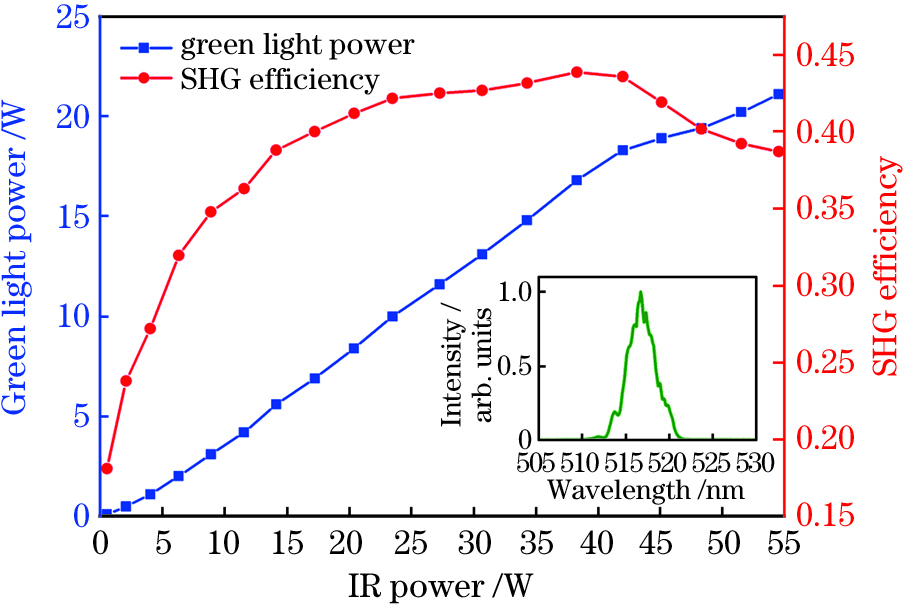
图 8. 绿光功率和SHG效率随IR激光输入功率的变化(插图为绿光光谱)
Fig. 8. Green light power and SHG efficiency versus IR laser input power (green light spectrum shown in inset)
4 结论
实验研究了以透射式衍射光栅作为展宽器和压缩器的高功率飞秒激光放大系统。通过控制和优化种子源,保证展宽器和各级光纤放大器的光谱形状不发生畸变,主放大后的光谱形状平滑,避免了去啁啾之后的光谱形状的畸变。为了获得高质量的脉冲输出,通过精细调节展宽器和压缩器中的光栅位置和入射角度,实现了系统内色散量的实时补偿。最终获得重复频率为500 kHz、平均功率为51.5 W、压缩效率为82.3%、单脉冲能量为103 μJ的高脉冲质量飞秒激光输出。在1 MHz的重复频率下,获得了平均功率为61.5 W、脉冲宽度为273 fs、光束质量为1.22的高脉冲质量飞秒激光输出;使用BBO晶体进行频率变换,获得了平均功率超过20 W、最大转化效率为44.3%的517 nm绿光激光输出。该套CPA技术在飞秒激光加工、医疗、**等领域具有良好的应用前景。该系统制备的紫外光源可用于光电子能谱实验,在材料表面科学研究领域具有重要的实用价值。
[3] Reduzzi M, Carpeggiani P, Kühn S, et al. Advances in high-order harmonic generation sources for time-resolved investigations[J]. Journal of Electron Spectroscopy and Related Phenomena, 2015, 204: 257-268.
[6] Morin F, Druon F, Hanna M, et al. Microjoule femtosecond fiber laser at 1.6 μm for corneal surgery applications[J]. Optics Letters, 2009, 34(13): 1991-1993.
[9] Gurevich E L, Hergenröder R. Femtosecond laser-induced breakdown spectroscopy: physics, applications, and perspectives[J]. Applied Spectroscopy, 2007, 61(10): 233A-242A.
[10] Franssen G C, Schleijpen H M A, van den Heuvel J C, et al. Femtosecond lasers for countermeasure applications[J]. SPIE, 2009, 7483: 748309.
[11] Fermann M E, Hartl I. Erratum: ultrafast fibre lasers[J]. Nature Photonics, 2013, 7(12): 1006.
[13] Backus S, Durfee C G III, Murnane M M, et al. High power ultrafast lasers[J]. Review of Scientific Instruments, 1998, 69(3): 1207-1223.
[14] Maurer RD, Optical waveguide light source: US3809549[P]. 1972- 03- 30.
[17] Röser F, Schimpf D, Schmidt O, et al. 90 W average power 100 μJ energy femtosecond fiber chirped-pulse amplification system[J]. Optics Letters, 2007, 32(15): 2230-2232.
[18] Rothhardt J, Hädrich S, Carstens H, et al. 1 MHz repetition rate hollow fiber pulse compression to sub-100-fs duration at 100 W average power[J]. Optics Letters, 2011, 36(23): 4605-4607.
[20] Kim K, Peng X, Lee W, et al. Monolithic polarization maintaining fiber chirped pulse amplification (CPA) system for high energy femtosecond pulse generation at 1.03 μm[J]. Optics Express, 2015, 23(4): 4766-4770.
[21] Zhao Z G, Kobayashi Y. Ytterbium fiber-based, 270 fs, 100 W chirped pulse amplification laser system with 1 MHz repetition rate[J]. Applied Physics Express, 2016, 9(1): 012701.
[22] Manchee C P K, Möller J, Miller R J D. Highly stable, 100 W average power from fiber-based ultrafast laser system at 1030 nm based on single-pass photonic-crystal rod amplifier[J]. Optics Communications, 2019, 437: 6-10.
[23] 向祥军, 李剑彬, 周丹丹, 等. 高峰值功率光纤脉冲激光系统[J]. 中国激光, 2018, 45(6): 0601002.
[24] Yang P L, Hao T, Hu Z Q, et al. Highly stable Yb-fiber laser amplifier of delivering 32-μJ, 153-fs pulses at 1-MHz repetition rate[J]. Applied Physics B, 2018, 124(8): 1-6.
[25] 周锋全, 袁帅, 郭政儒, 等. 高功率小型光纤飞秒激光放大系统[J]. 激光与光电子学进展, 2018, 55(10): 103201.
[29] Limpert J, Clausnitzer T, Liem A, et al. High-average-power femtosecond fiber chirped-pulse amplification system[J]. Optics Letters, 2003, 28(20): 1984-1986.
[30] 郝静宇, 刘博文, 宋寰宇, 等. 基于三阶色散补偿的光纤飞秒激光放大系统[J]. 激光与光电子学进展, 2018, 55(5): 051404.
[32] 牛佳, 刘博文, 宋寰宇, 等. 基于光谱控制与色散优化的飞秒啁啾脉冲放大系统[J]. 中国激光, 2020, 47(1): 0101006.
[33] 安德鲁·温纳。 超快光学[M]. 郑铮, 赵欣, 刘建胜, 等, 译. 北京: 国防工业出版社, 2015: 308- 309.
AndrewW. Ultrafast optics[M]. Zheng Z,Zhao X,Liu J S,et al, Transl. Beijing: National Defense Industry Press, 2015: 308- 309.
[34] Martinez O. 3000 times grating compressor with positive group velocity dispersion: application to fiber compensation in 1.3-1.6 μm region[J]. IEEE Journal of Quantum Electronics, 1987, 23(1): 59-64.
[37] Cheriaux G, Rousseau P, Salin F, et al. Aberration-free stretcher design for ultrashort-pulse amplification[J]. Optics Letters, 1996, 21(6): 414-416.
赵其锴, 丛振华, 刘兆军, 张行愚, 赵智刚. 百微焦飞秒光纤啁啾脉冲放大激光系统[J]. 中国激光, 2021, 48(7): 0701001. Zhao Qikai, Cong Zhenhua, Liu Zhaojun, Zhang Xingyu, Zhao Zhigang. Hundred Microjoule Femtosecond Fiber Chirped Pulse Amplification Laser System[J]. Chinese Journal of Lasers, 2021, 48(7): 0701001.