涡旋光束轨道角动量的一种新型干涉检测方法
下载: 1429次
1 引言
涡旋光束是一种相位结构为exp(i
虽然涡旋光束具有螺旋相位结构,但在强度分布上只能观察到其圆环形结构,并不能直接判断涡旋光束的OAM,因此,涡旋光束的OAM检测成为了一项重要的必备技术。进行OAM检测时,通常利用Mach-Zehnder干涉仪使平面波与涡旋光束发生干涉,根据叉形干涉条纹的分支数判断涡旋光束的拓扑荷[15-19]。此外,球面波与涡旋光束干涉形成的分支螺旋干涉条纹[20-21],球面波与涡旋光束镜像光束干涉产生的径向螺旋干涉图[22-23],通过环状多针孔产生的特殊干涉图,以及利用多针孔相位恢复算法、窄环带孔径形成的径向环带分布干涉图和透过锥透镜干涉图等都可以实现OAM定量测量。除了干涉法外,光栅法[24]、Dove棱镜法[22]、圆形或多边形振幅/相位光阑法[25-27]、椭圆光阑法[28]、光学转换法[29-30]、柱透镜法[31-32]以及计算机全息法[33-34]都可以检测涡旋光束的OAM。
本文提出一种简单的干涉法检测涡旋光束的OAM,利用反射式相位型空间光调制器(SLM)的不完全调制[35]特性,将部分未调制的高斯光束与调制的涡旋光束直接发生干涉,从而产生花瓣状的干涉图案,结果可直观反映参与干涉涡旋光束的OAM。基于该原理,研究了Bessel-Gaussian光束的干涉模式,分析了具有径向和角向因子的高阶多环Laguerre-Gaussian光束的干涉图案。
2 实验方案
2.1 实验装置
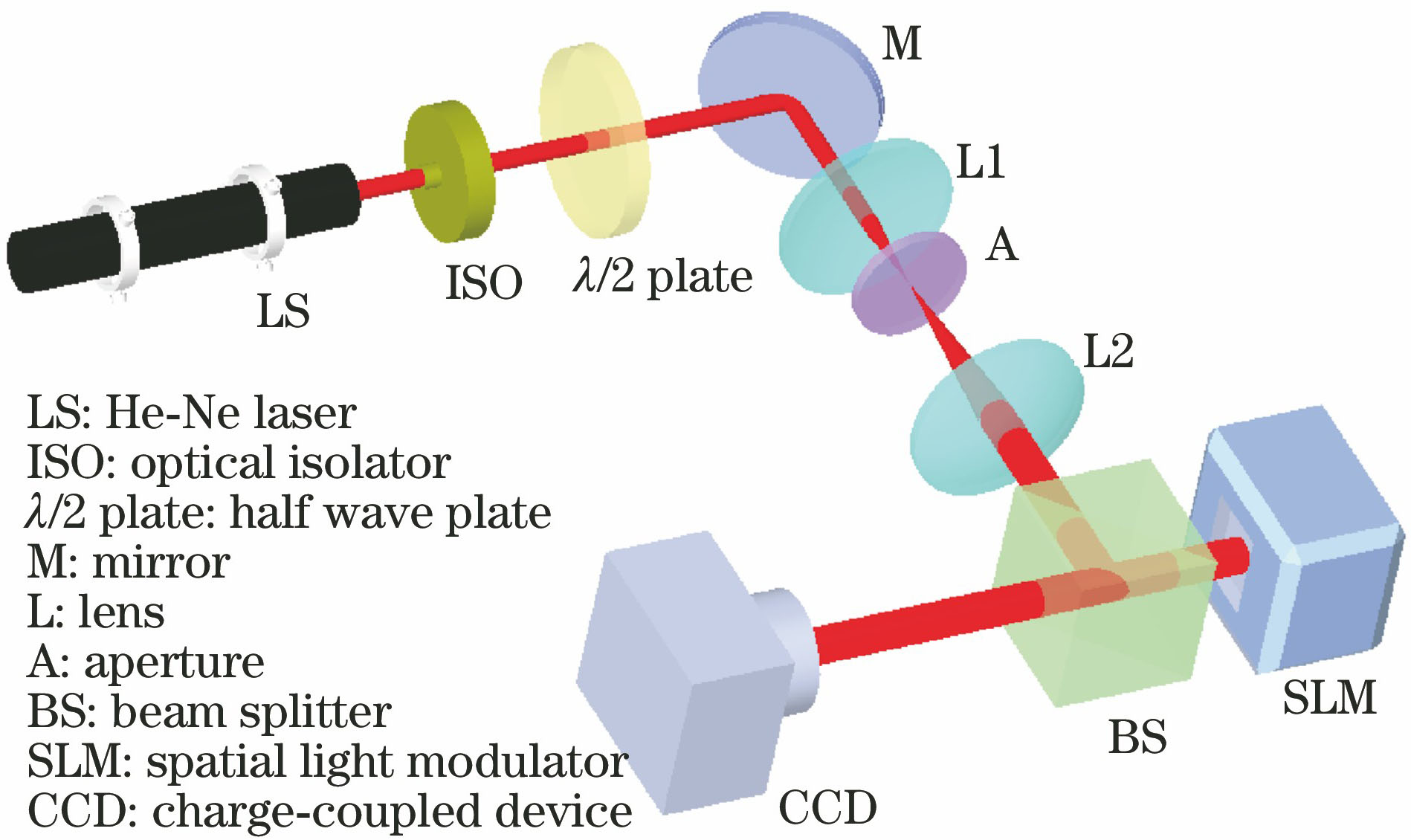
图 1. 产生和检测涡旋光束OAM的实验装置图
Fig. 1. Experimental device diagram for generating and detecting OAM of vortex beams
2.2 实验原理
目前,SLM是一种产生涡旋光束最常用的工具,理论上高斯光束通过某一相位板后可得到与该相位板对应的调制光束。然而,由于SLM工作原理[35] 的限制,在加载相应的相位板后,尽管入射线偏振光束的偏振方向与其液晶屏幕的长边平行,空间光调制器对光的位相调制效率最高,但是仍然有部分入射光无法完全调制,因此,在相应的相位板上需要额外增加一个条形衍射光栅,将未调制的高斯光束移开,这样才能得到需要的光束。以L
基于SLM的工作原理可以发现,当使用SLM产生的涡旋光束对应的相位板不叠加条形衍射光栅时,未调制的高斯光束可与涡旋光束直接发生干涉,产生花瓣状的干涉图案,进而可以检测该涡旋光束的OAM。
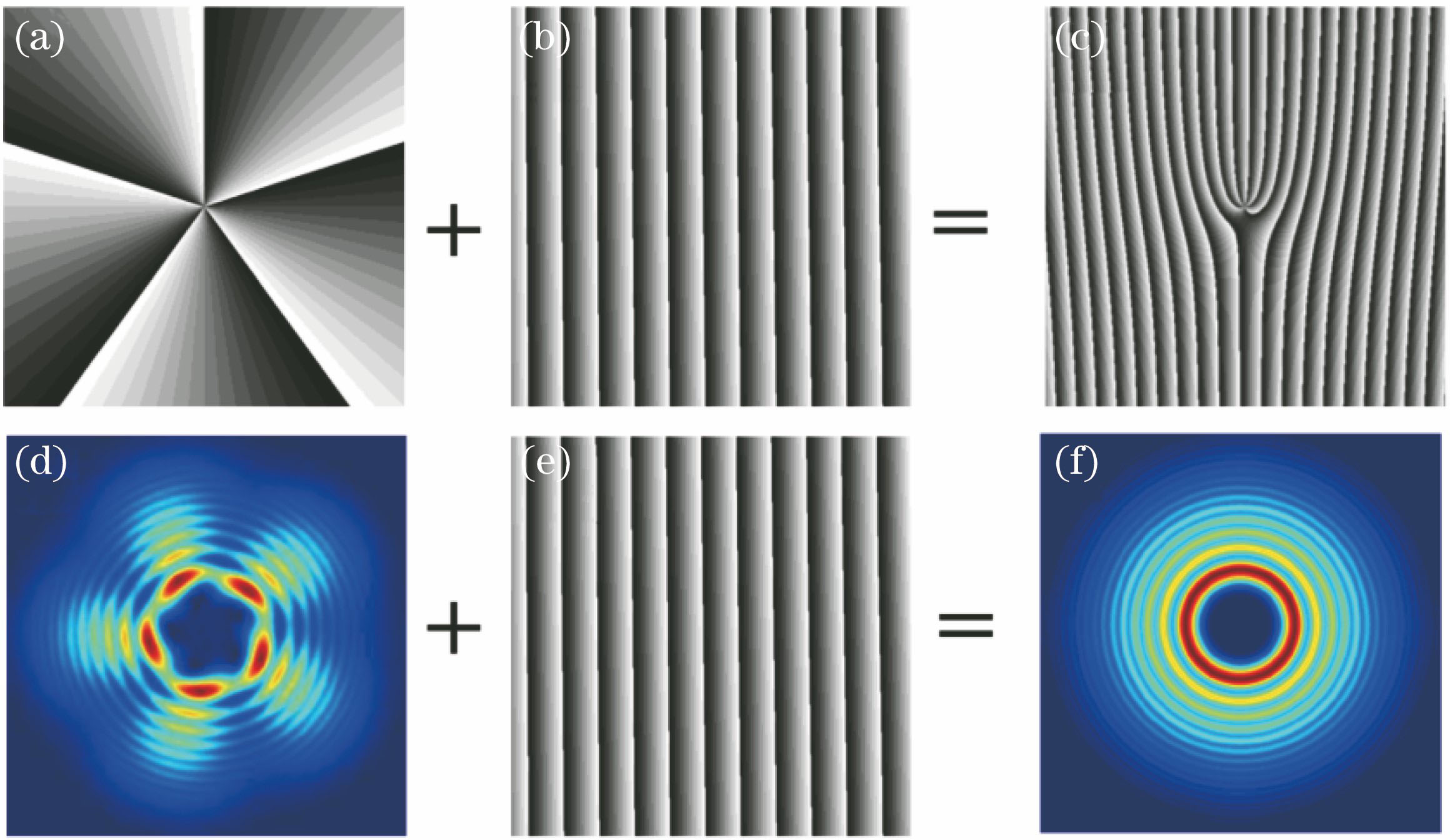
图 2. 干涉模式的产生原理图。(a) LG05光束对应的螺旋相位板;(b)条形衍射光栅;(c)叉形光栅; (d) LG05光束的干涉花瓣模式;(e)圆环形的LG05光束
Fig. 2. Schematic of generation of interference mode. (a) Spiral phase plate corresponding to LG05 beam; (b) strip diffraction grating; (c) fork grating; (d) interference petal pattern of LG05 beam; (e) circular LG05 beam
实验得到的干涉是空间光调制器自身不完全调制的结果,是未被调制的高斯光束与调制后的光束直接发生的干涉,由此可见,入射光束满足空间光调制的偏振要求,即调制部分和未调制部分光束的偏振方向一致,这也是干涉图案能够清晰稳定的主要原因。由于两束光的光强并非连续可调,因此,首先必须测量SLM调制涡旋光束与未调制高斯光束的光强之比,分析对实验结果判断的影响。具体过程如下。
分别对
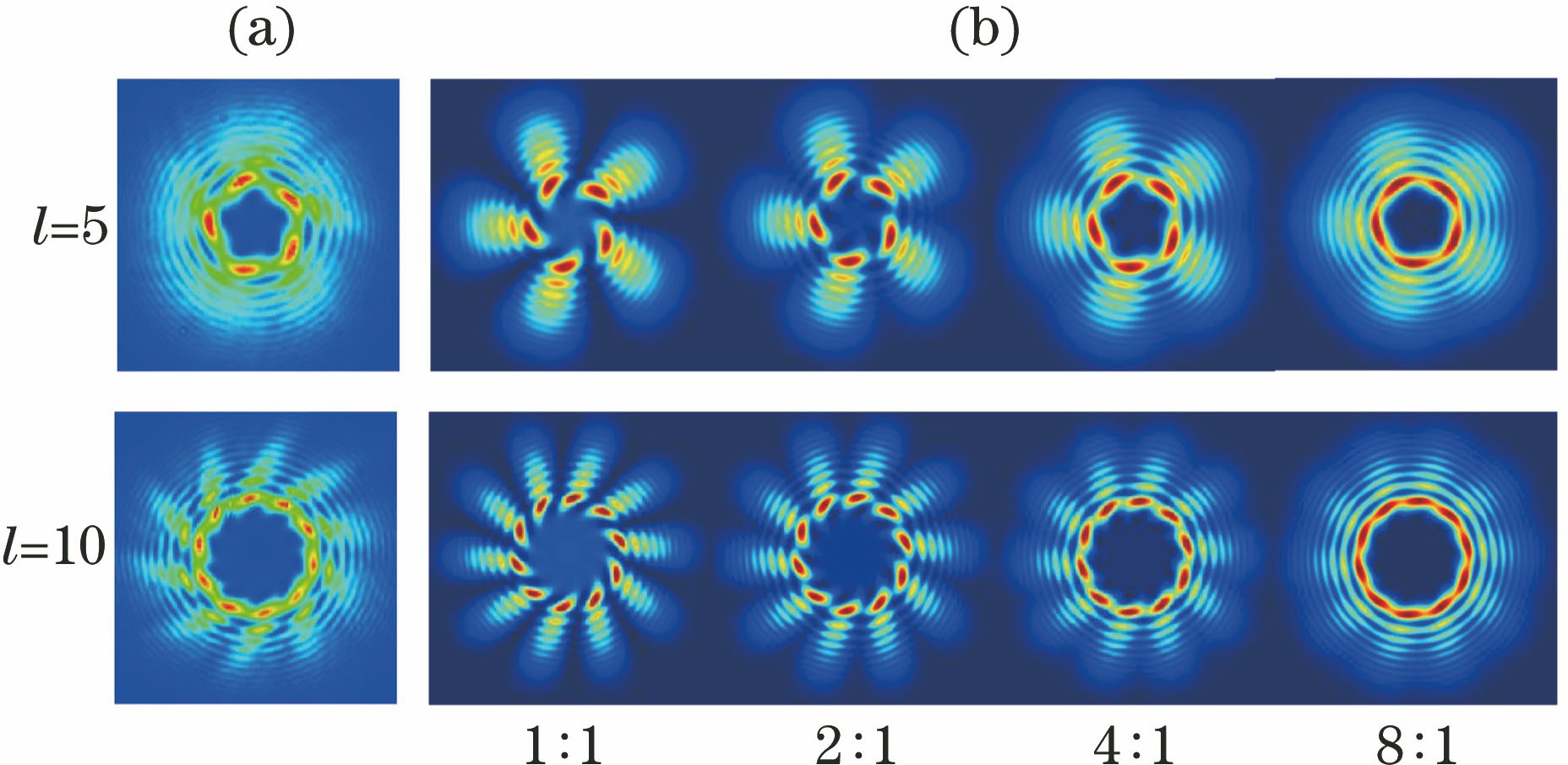
图 3. 干涉图案的实验和理论对比图。 (a)实验结果;(b)理论结果
Fig. 3. Comparison of experimental and theoretical results of interference figures. (a) Experimental results; (b) theoretical results
3 实验与结果
3.1 Bessel-Gaussian光束OAM检测
利用实验装置产生Bessel-Gaussian光束,采用干涉法对OAM进行检测。Bessel-Gaussian光束具有近似Bessel函数的强度分布,且高阶Bessel-Gaussian光束在光轴上具有无衍射暗核的特点,传输函数[36]可以描述加载在SLM上的Bessel-Gaussian光束的相位全息图,即
式中:
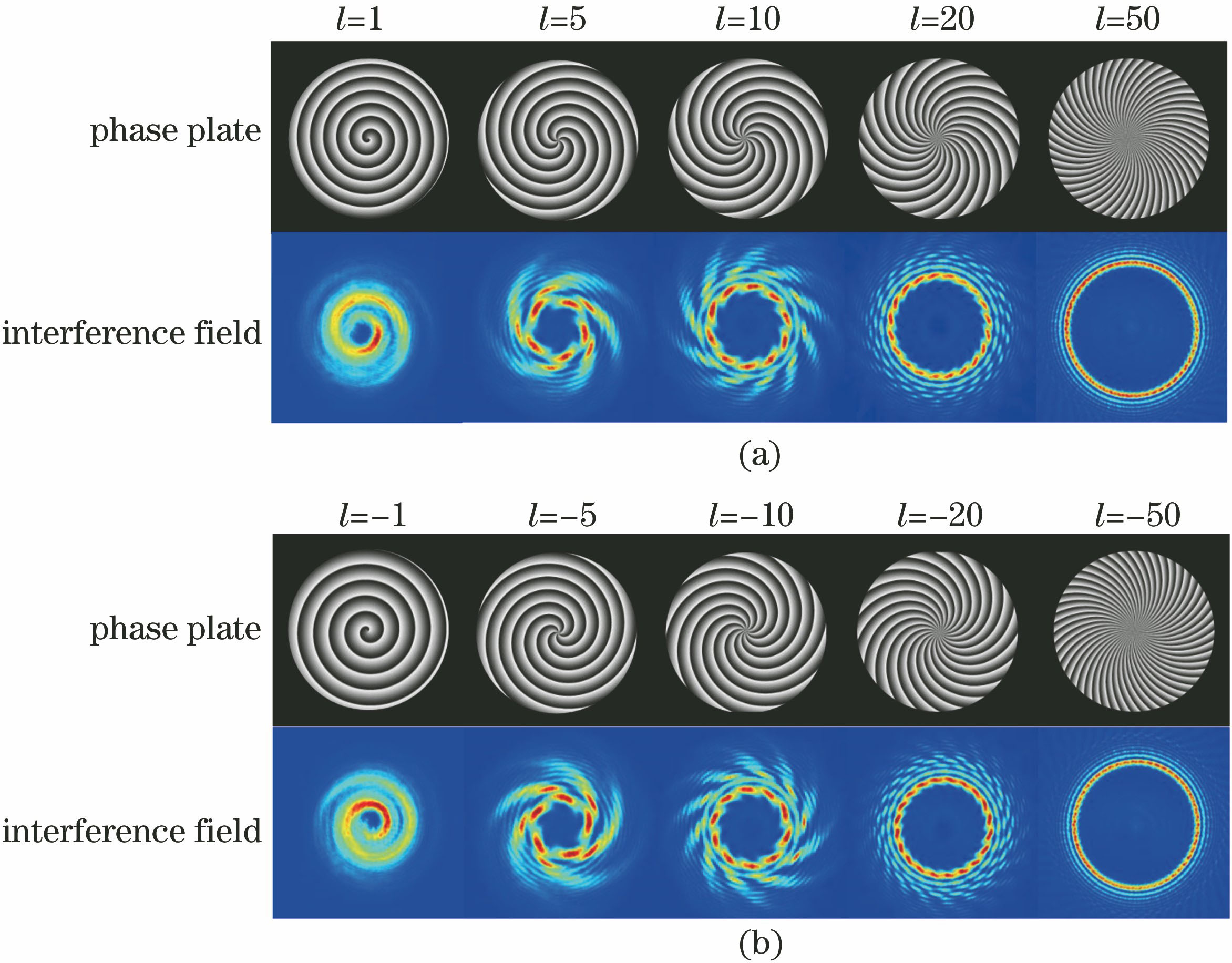
图 4. 产生不同OAM的Bessel-Gaussian光束的相位板和花瓣状干涉光场分布。(a) OAM为正;(b) OAM为负
Fig. 4. Phase plates to generate Bessel-Gaussian beams with different OAMs and corresponding petal-like interference field distributions. (a) OAM is positive; (b) OAM is negative
3.2 高阶多环Laguerre-Gaussian光束OAM检测
对于Laguerre-Gaussian光束OAM的检测,其相位板和光场结构都更复杂,因此首先根据Laguerre-Gaussian光束相位模式设计相位板,然后才能进行实验。将实验所得的高阶多环Laguerre-Gaussian光束光强分布与理论结果进行对比,检验结果的正确性。由理论可知,传输函数[17]可以得到L
式中:
利用
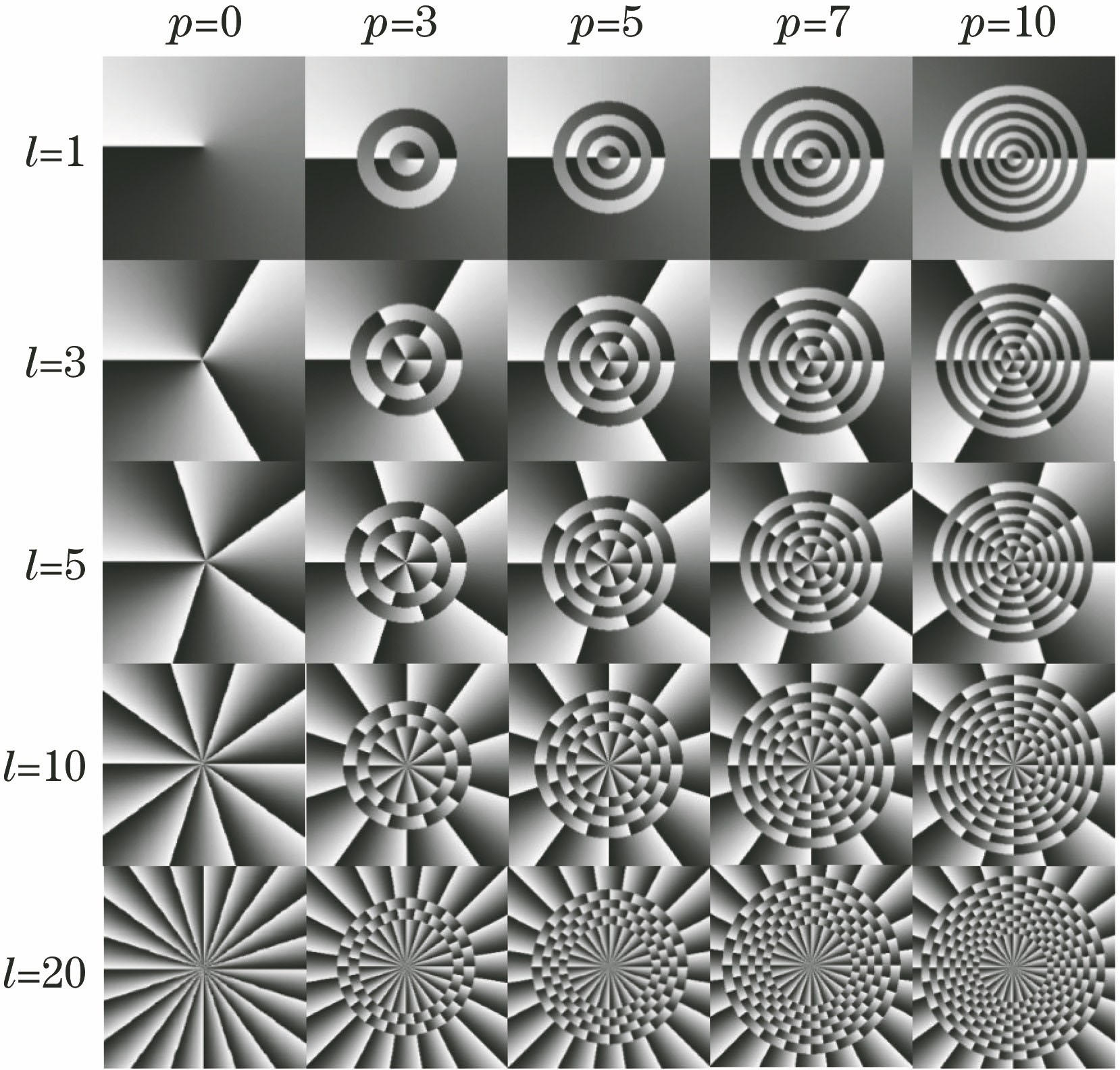
图 5. 高阶多环Laguerre-Gaussian光束对应的相位板
Fig. 5. Phase plates corresponding to high-order multi-ring Laguerre-Gaussian beam
式中:光束半径
由于高阶多环Laguerre-Gaussian光束及其干涉的光场分布复杂,因此
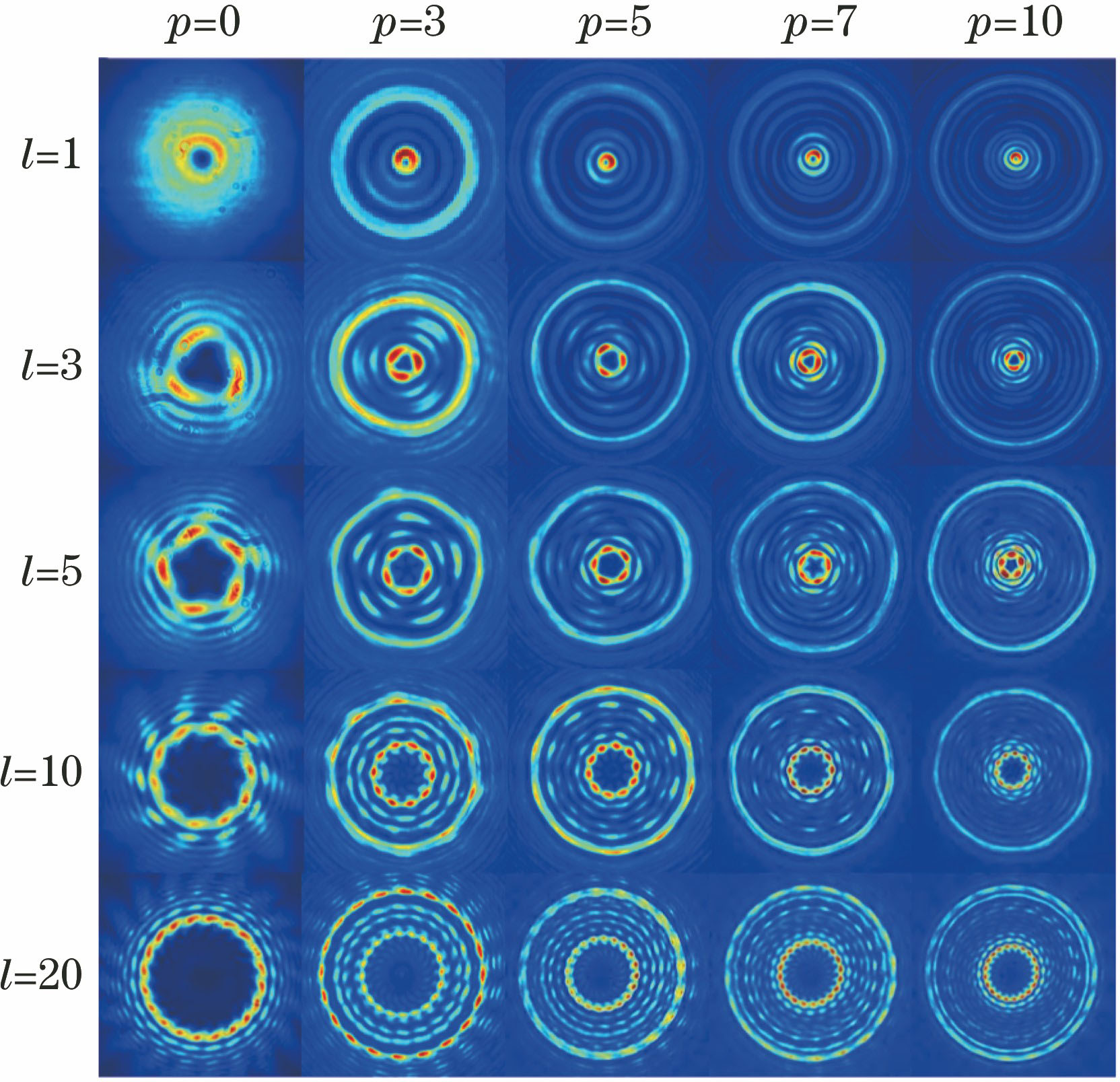
图 6. 高阶多环Laguerre-Gaussian光束的干涉图案
Fig. 6. Interference figures of high-order multi-ring Laguerre-Gaussian beams
4 结论
本文得到的干涉图案具有完美的对称性和高清晰度,对于Bessel-Gaussian光束,即使OAM值达到±50甚至更高,也可以轻易辨别,这是传统干涉方法无法达到的结果。
传统干涉法中都必须将光路分成不同光学装置调制的两部分——涡旋光束和参考光束,最后进行合束干涉,双光路干涉对光路的稳定性和同轴性要求很高,激光偏振、相位差和光路的微小波动或者高斯光束与涡旋光束的传播轴略有差别都会导致干涉模式不稳定,因此,传统方法需要对干涉光路进行精确控制和调节,而在已经报道的采用Mach-Zehnder干涉仪方法测量光束角动量的实验中并没有对光路两臂相位差进行稳定锁定和精确控制。如果不对干涉仪两个臂的光束相位差进行稳定锁定和精确控制,由分束器(或偏振分束器)叠加的两束光束干涉图案会不稳定,甚至输出模式可能表示为两束光束强度(或不同偏振分量)的叠加,并非真正的干涉。故双光路干涉方法很难得到稳定且对称的干涉图案。
此外,空间光调制器是目前产生涡旋光束最常用的方法,虽然在理论上涡旋光束的OAM与相位板信息相对应,但实际中实验的非理想性,如光束未准直以及光路出现未知的误差等因素,都会使得涡旋光束与加载的相位板信息不完全对应,尤其是对于较复杂的光场模式如高阶多环Laguerre-Gaussian光束,所得结果更不理想。同时,要产生相应的涡旋光束需要精细操控,而以往在使用空间光调制器产生涡旋光束时,仅由圆环形强度分布的涡旋光束不能判断光束是否为对应的涡旋光束。
因此,本文利用高斯光束和待测涡旋光束进行同轴同偏振干涉测量,只有一条光路,可以保证两束光之间的相位差恒定,从而形成稳定的干涉图案。以往实验中没有发现这一现象的原因是螺旋相位板上直接叠加了条形光栅相位,未调制的高斯光束被移开,得到了环形强度分布的涡旋光束,而没有观察到干涉图案。
本文方案的检测方法不仅可以通过空间光调制器直接产生涡旋光束,还可以验证产生的轨道角动量光束的准确性,从而达到检验并快速调整光路的目的。该方案具有光路简单、易操控、现象明显、稳定性好、对称性高和检测范围宽等优点,可以对传统的干涉法进行很好的补充,为涡旋光束的后续使用提供了可靠保证和便利。
[1] Allen L, Beijersbergen M W. Spreeuw R J C, et al. Orbital angular momentum of light and the transformation of Laguerre-Gaussian laser modes[J]. Physical Review A, 1992, 45(11): 8185-8189.
[2] 张洪. 涡旋光轨道角动量的产生与检测技术的研究[D]. 成都: 电子科技大学, 2016: 1- 6.
ZhangH. Research of the generation and detection about vortex beams[D]. Chengdu: University of Electronic Science and Technology of China, 2016: 1- 6.
[3] Grier D G. A revolution in optical manipulation[J]. Nature, 2003, 424(6950): 810-816.
[4] Paterson L. MacDonald M P, Arlt J, et al. Controlled rotation of optically trapped microscopic particles[J]. Science, 2001, 292(5518): 912-914.
[5] Tao S H, Yuan X C, Lin J, et al. Fractional optical vortex beam induced rotation of particles[J]. Optics Express, 2005, 13(20): 7726-7731.
[6] Li M M, Yan S H, Yao B L, et al. Optically induced rotation of Rayleigh particles by vortex beams with different states of polarization[J]. Physics Letters A, 2016, 380(1/2): 311-315.
[10] Vallone G. D'Ambrosio V, Sponselli A, et al. Free-space quantum key distribution by rotation-invariant twisted photons[J]. Physical Review Letters, 2014, 113(6): 060503.
[11] Torner L, Torres J P, Carrasco S. Digital spiral imaging[J]. Optics Express, 2005, 13(3): 873-881.
[12] 曾军, 陈亚红, 刘显龙, 等. 部分相干涡旋光束研究进展[J]. 光学学报, 2019, 39(1): 0126004.
[14] AndrewsD, BabikerM. The angular momentum of light [M]. Cambridge: Cambridge University Press, 2012.
[15] Harris M, Hill C A, Tapster P R, et al. Laser modes with helical wave fronts[J]. Physical Review A, 1994, 49(4): 3119-3122.
[16] Clifford M A, Arlt J, Courtial J, et al. High-order Laguerre-Gaussian laser modes for studies of cold atoms[J]. Optics Communications, 1998, 156(4/5/6): 300-306.
[17] Ohtake Y, Ando T, Fukuchi N, et al. Universal generation of higher-order multiringed Laguerre-Gaussian beams by using a spatial light modulator[J]. Optics Letters, 2007, 32(11): 1411-1413.
[18] Matsumoto N, Ando T, Inoue T, et al. Generation of high-quality higher-order Laguerre-Gaussian beams using liquid-crystal-on-silicon spatial light modulators[J]. Journal of the Optical Society of America A, 2008, 25(7): 1642-1651.
[20] Sueda K, Miyaji G, Miyanaga N, et al. Laguerre-Gaussian beam generated with a multilevel spiral phase plate for high intensity laser pulses[J]. Optics Express, 2004, 12(15): 3548-3553.
[21] Padgett M, Arlt J, Simpson N, et al. An experiment to observe the intensity and phase structure of Laguerre-Gaussian laser modes[J]. American Journal of Physics, 1996, 64(1): 77-82.
[22] Leach J, Padgett M J, Barnett S M, et al. Measuring the orbital angular momentum of a single photon[J]. Physical Review Letters, 2002, 88(25): 257901.
[23] Slussarenko S. D'Ambrosio V, Piccirillo B, et al. The polarizing Sagnac interferometer: a tool for light orbital angular momentum sorting and spin-orbit photon processing[J]. Optics Express, 2010, 18(26): 27205-27216.
[24] 柯熙政, 谢炎辰, 张颖. 涡旋光束轨道角动量检测及其性能改善[J]. 光学学报, 2019, 39(1): 0126017.
[25] Hickmann J M. Fonseca E J S, Soares W C, et al. Unveiling a truncated optical lattice associated with a triangular aperture using light's orbital angular momentum[J]. Physical Review Letters, 2010, 105(5): 053904.
[26] Mourka A, Baumgartl J, Shanor C, et al. Visualization of the birth of an optical vortex using diffraction from a triangular aperture[J]. Optics Express, 2011, 19(7): 5760-5771.
[27] Mazilu M, Mourka A, Vettenburg T, et al. Simultaneous determination of the constituent azimuthal and radial mode indices for light fields possessing orbital angular momentum[J]. Applied Physics Letters, 2012, 100(23): 231115.
[28] 毛宁, 韦宏艳, 蔡冬梅, 等. 复合涡旋光束拓扑荷数测量的仿真研究[J]. 中国激光, 2019, 46(1): 0104008.
[29] Berkhout G C G, Lavery M P J, Courtial J, et al. . Efficient sorting of orbital angular momentum states of light[J]. Physical Review Letters, 2010, 105(15): 153601.
[30] Lavery M P J, Berkhout G C G, Courtial J, et al. . Measurement of the light orbital angular momentum spectrum using an optical geometric transformation[J]. Journal of Optics, 2011, 13(6): 064006.
[31] Alperin S N, Niederriter R D, Gopinath J T, et al. Quantitative measurement of the orbital angular momentum of light with a single, stationary lens[J]. Optics Letters, 2016, 41(21): 5019-5022.
[32] Alperin S N, Siemens M E. Angular momentum of topologically structured darkness[J]. Physical Review Letters, 2017, 119(20): 203902.
[33] Khonina S N, Kotlyar V V, Skidanov R V, et al. Gauss-Laguerre modes with different indices in prescribed diffraction orders of a diffractive phase element[J]. Optics Communications, 2000, 175(4/5/6): 301-308.
[34] Gibson G, Courtial J, Padgett M J, et al. Free-space information transfer using light beams carrying orbital angular momentum[J]. Optics Express, 2004, 12(22): 5448-5456.
[35] Wang Z, Yan Y, Arbabi A, et al. Orbital angular momentum beams generated by passive dielectric phase masks and their performance in a communication link[J]. Optics Letters, 2017, 42(14): 2746-2749.
[36] Yang L, Qian D D, Xin C, et al. Two-photon polymerization of microstructures by a non-diffraction multifoci pattern generated from a superposed Bessel beam[J]. Optics Letters, 2017, 42(4): 743-746.
[37] Pan S Z, Pei C Y, Liu S, et al. Measuring orbital angular momentums of light based on petal interference patterns[J]. OSA Continuum, 2018, 1(2): 451-461.
[38] Dennis MR, O'Holleran K, Padgett M J. Singular optics: optical vortices and polarization singularities[M] ∥Wolf E. Progress in Optics. Amsterdam, Netherlands: Elsevier, 2009: 293- 363.
Article Outline
裴春莹, 茅志翔, 徐素鹏, 夏勇, 尹亚玲. 涡旋光束轨道角动量的一种新型干涉检测方法[J]. 激光与光电子学进展, 2019, 56(14): 140502. Chunying Pei, Zhixiang Mao, Supeng Xu, Yong Xia, Yaling Yin. Interferometric Detection Method for Orbital Angular Momentum of Vortex Beams[J]. Laser & Optoelectronics Progress, 2019, 56(14): 140502.