Advanced modulation formats for underwater visible light communications [Invited]
Download: 693次
Visible light communication (VLC) has become an attractive and promising technology for high-speed indoor wireless communication. Unlike radio frequency (RF) wireless communication, VLC utilizes the already installed light emitting diode (LED) as data transmitters and can be applied in environments where RF is not necessarily allowed, such as airplanes, military facilities, and underwater communication. In VLC, many advanced modulation formats are investigated to achieve high-speed transmission successfully[1
Table
Table 1. Summary of Research Results of VLC Systems
|
In 2008, the Visible Light Communications Association (VLCC), established in Japan in October 2003, researched and realized a 2 km indoor visible light transmission based on OOK modulation of 1.022 Mb/s[1]. In the same year, Oxford University increased the speed to 80 Mb/s with post-equalization at a distance of 10 cm[3]. In 2010, the German HHI began to study indoor visible light transmission based on discrete multitone (DMT) modulation, initially using a white LED to achieve a 513 Mb/s over 30 cm transmission[6]. By 2012, the German Heinrich Hertz Institute (HHI) had made advancements with RGB LEDs and wavelength division multiplexing (WDM) to achieve a 1.25 Gb/s over 10 cm transmission[8]. In the same year, Italy’s Scuola Superiore Sant’Anna (SSSUP) adopted the optimized DMT modulation method and RGB WDM to achieve 1.5 Gb/s in a single-LED single-channel over a 10 cm transmission, and the RGB three-color WDM multiplexing rate reached 3.4 Gb/s[10]. In 2014, SSSUP in Italy continued the study of DMT modulation, using a common RGBY lamp and WDM to achieve a downlink of 5.6 Gb/s and an uplink of 1.5 Gb/s over a 1.5 m transmission[13]. In 2015, the University of Cambridge used the μLED and pre-equalization method based on PAM4 modulation to achieve an indoor 2 Gb/s over 60 cm transmission[17]. Wang
From the comprehensive domestic and foreign research status, we can see that after more than 10 years of development, VLC has received more and more attention from all over the world, and an upsurge of VLC research and industrialization has also been set off internationally. At the same time, from the perspective of the development trend from 2010 to now, the key technology for realizing high-speed VLC has become the most important direction for the future development of VLC. How to break through the system bandwidth limitation and effectively compensate the system for multiple impairments is a major issue in improving the VLC capacity and transmission distance. The new high spectral efficiency modulation and equalization techniques are the key methods to solve the above problems. Domestic and foreign research institutes have already carried out a lot of fruitful research work here which can be seen from Table
Nowadays, underwater wireless information transmission is an emerging area that needs to be investigated. Wang
What is more, generally on-off-keying (OOK) digital modulation was used in past underwater transmission[29]. To realize high-speed UVLC transmission, multilevel QAM modulation must be considered subject to the stringent bandwidth of the LED. This kind of QAM modulation requires good linearity because relatively large nonlinearity will induce severe intersymbol interference (ISI) and will result in failure detection. Therefore, the nonlinearity mitigation is one of the significant challenges for the UVLC system using high-order QAM modulation. The environment in UVLC is more complex, which needs advanced modulation formats to resist the loss of complex environments, make the full use of modulated bandwidth, achieve high-speed transmission, as well as obtain a stable and reliable system.
Table
Table 2. Summary of Research Results of LED UVLC Systems
|
As a promising solution, the Volterra series-based equalizer plays significant role in mitigating the nonlinearity in the VLC[31]. In our group’s previous work, a blind post-equalization scheme called the cascaded Volterra modified multi-modulus algorithm was employed and demonstrated to compensate for linearity and mitigate the LED nonlinearity in the CAP modulation-based VLC system[32].
We choose three advanced modulation formats which are CAP, orthogonal frequency-division multiplexing (OFDM) and DFT-S OFDM modulation for UVLC. To the best of our knowledge, the performance comparison of these three modulation formats has not been reported, especially for UVLC. Such comparison is of great value considering the requirements of high-speed and valid transmission for underwater applications.
In this Letter, we present a comprehensive comparison of advanced modulation formats including CAP, OFDM, and DFT-S OFDM. We also discuss the corresponding digital signal processing (DSP). For each format, a post-equalizer structure consisting of two cascaded stages is suggested. For the first time, a post-equalizer based on novel recursive least square (RLS)- Volterra is presented to mitigate the nonlinear effect and improve the system performance of UVLC. The Volterra is used to compensate the nonlinearity and the RLS algorithm is used to update the tap coefficients of Volterra. Then these three typical advanced modulation formats are all realized at the same bit rate of 3 Gbit/s. The comparison is carried out to evaluate the performance of each modulation format in terms of peak-to-average power ratio (PAPR), nonlinear equalization, LED current, optical power, and peak-to-peak voltage. Finally, CAP-64 and DFT-S OFDM are successfully achieved over 1.2 m of UVLC transmission under a hard-decision forward error correction (HD-FEC) threshold of
In this part, we describe the signal generation flow and recovery flow of CAP-64, OFDM 64QAM, and DFT-S OFDM 64QAM. All of these three modulation formats need mapping and upsampling in the generation process. While CAP has an extra need for IQ separation and shaping filters at the cost of convolution. DFT-S requires an extra fast Fourier transform (FFT) and inverse fast Fourier transform (IFFT); and the first-level post-equalizer for each format is the RLS-Volterra in the time domain. The second-level post-equalizer for CAP is RLS-Volterra in the time domain, however, for DFT-S and OFDM it is zero-forcing (Z-F) in the frequency domain. Figure
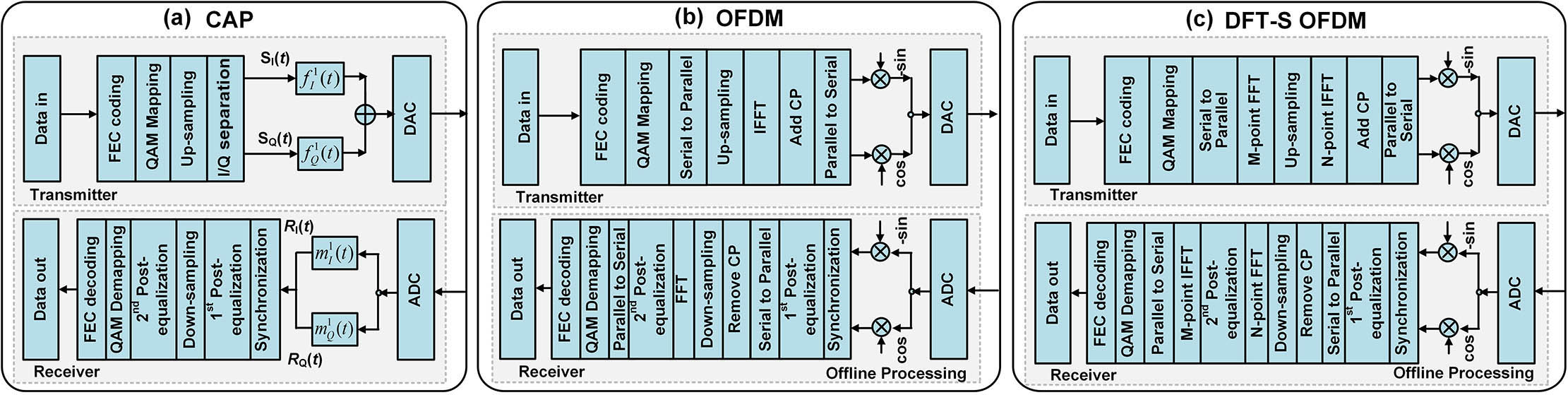
Fig. 1. Schematic diagram: (a) CAP-64 system; (b) OFDM 64QAM system; (c) DFT-S OFDM 64QAM system.
Figure
Figure
Figure
Then we compare three advanced formats in terms of simulation and experiment. First, to further study the effect of PAPR, we evaluate the PAPR of the three modulation formats, as shown in Fig.
We get further comparison by means of a practical experiment. In this experiment, the comparison is carried out under fair conditions with the same experimental setup. The experimental setup of the UVLC system and VLC system is shown in Fig.
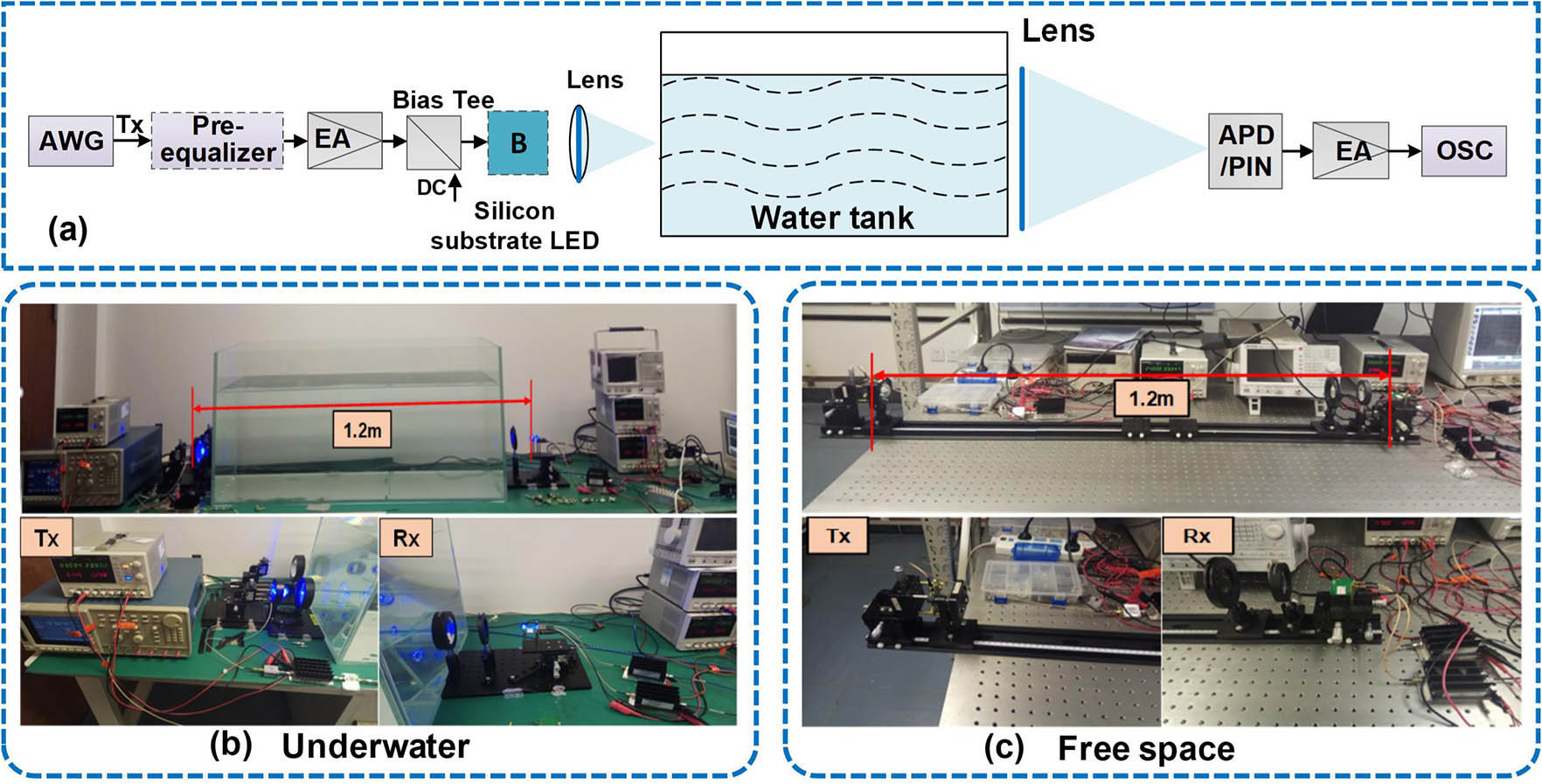
Fig. 4. Experimental setup of (a), (b) the underwater VLC system and (c) the free-space VLC system.
At the receiver, the lens is placed in front of the receiver to focus light. A photodiode (PIN, Hamamatsu 10784) is used to detect the received light signals by converting the optical signals to electrical signals. Amplifiers and digital storage oscilloscopes (OSC, Agilent DSO54855A) are used to amplify and quantize the received signals so that the acquired data can be processed offline. In offline processing, each format follows their own schematic diagram. Finally, the signals are de-mapped to obtain the original bit sequence and the system BER performance is calculated.
In the experiment, in order to compare three advanced formats on the same condition fairly, the same bandwidth is utilized. The sample rate of the AWG is set at 2 G Sa/s all the time. Due to the upsample factor being 4, the bandwidth is 500 Mbaud, and the order for the three advanced formats is 6. As a result, the maximum achievable transmission data rate is 3 Gb/s. The subcarriers of DFT-S OFDM and OFDM are 256.
Specifically, there are some differences among three advanced modulation formats in offline post-equalization. Figure
To investigate the effect of first-level post-equalization here, the spectrum maps at the transmitter, at the receiver, and after the first post-equalization in the CAP, DFT-S, and OFDM systems are presented in Fig.

Fig. 6. Spectrum maps for the UVLC system at the transmitter, at the receiver, and after the first post-equalization: (a) CAP at the transmitter; (b) DFT-S OFDM at the transmitter; (c) OFDM at the transmitter; (d) CAP at the receiver; (e) DFT-S OFDM at the receiver; (f) OFDM at the receiver; (g) CAP after the first post-equalization; (h) DFT-S OFDM after the first post-equalization; and (i) OFDM after the first post-equalization.
When considering the main source of nonlinearity in UVLC compared to traditional VLC, we focus on the effect of water. As a result, the comparison of three formats in both the UVLC and VLC systems has been investigated. The performance results are shown in Figs.
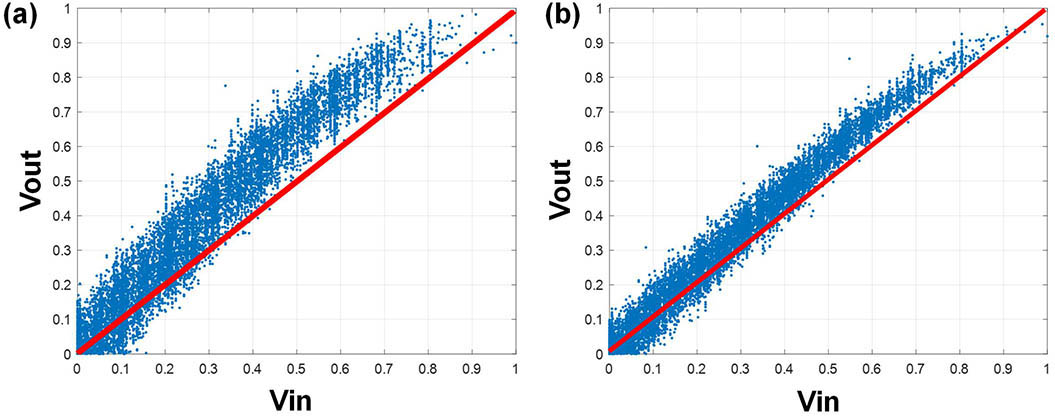
Fig. 7. Amplitude to amplitude (AM/AM) response of CAP modulation in (a) the UVLC system and (b) the VLC system.
The latest expression of the underwater channel only takes optical pass loss into consideration[40,41]. The real underwater VLC channel includes the frequency response of electronic devices (signal amplifier, pre-equalizer, LED driver, LED, etc.) and an optical channel. For the whole UVLC system, the expression of the underwater channel includes the transmitter, the underwater channel and the receiver. The nonlinearity in the UVLC system that arose from the LED, the transmitter driving circuits, PIN photodetector, and the receiver amplification circuits may introduce extra nonlinear noise and can cause detrimental effects to the signal reception. Therefore, it is an essential issue to measure the statistic characteristics of the nonlinear response of the whole UVLC system to achieve a better system performance.
In order to make a good evaluation of the nonlinearities that exist in UVLC and VLC, an amplitude to amplitude (AM/AM) response of CAP modulation was introduced in Ref. [42] in UVLC and the VLC system has been measured in Fig.
Figure
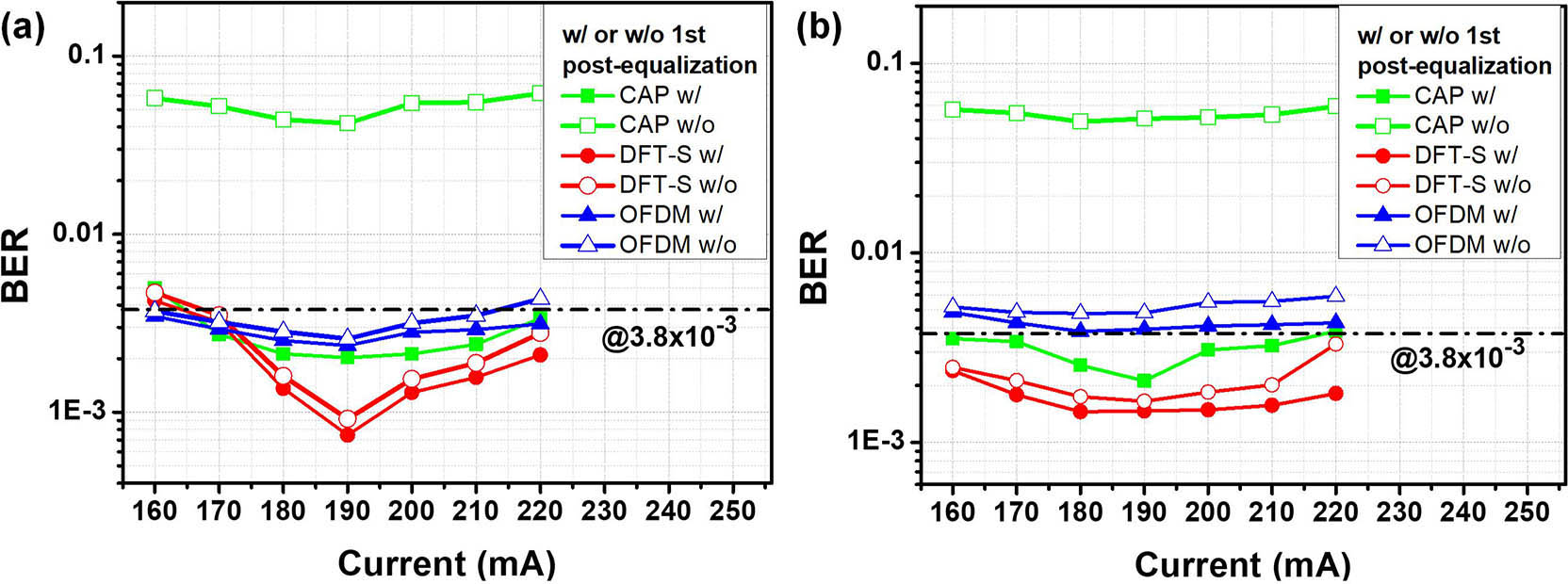
Fig. 8. BER versus LED current in (a) the traditional VLC system and (b) the UVLC system.
What is more, when comparing the experimental results between VLC and UVLC, the performance improvement caused by the first post-equalization is larger in the UVLC system. It is further explained that the nonlinearity effect is larger in the UVLC system, and for three modulation formats, the BER performance in VLC outperforms in UVLC. This can be explained by the fact that since the attenuation of water is relatively large, the SNR of the receiver decreases, so the BER is deteriorated. But for the acceptable operating range of LED current, we found that the operating range in UVLC is larger than in VLC. That is because when current increases, the optical power of the VLC system will increase very fast. The large optical power will soon saturate the receiver and result in failure detection. So in the case of large bias current, the BER in free-space deteriorates faster than underwater.
Regarding the performance improvement of three different modulation formats, CAP modulation presents a larger enhancement than the other two. The optimization for OFDM and DFT-S OFDM is relatively smaller than for CAP, which can be explained that for these two modulation formats, the major nonlinear equalization compensation is conducted by the second post-equalization (i.e., Z-F equalization). The impact of the second post-equalization for these two formats will be analyzed in Fig.
For a nonlinear system, we can use the Volterra series for characterization and use it for nonlinear compensation[15,16]. To get a further elaboration of the degree of nonlinearity in the VLC and the UVLC systems, we also compare the performance of three advanced modulation formats using Volterra for nonlinear compensation. We investigate the effect of the order of Volterra in the first post-equalization. When the order of Volterra is changed, the number of taps of RLS needs to be modified correspondingly to get an optimal BER value. The results are shown in Fig.

Fig. 9. BER versus order of Volterra in the UVLC and VLC systems with (a) CAP, (b) DFT-S, and (c) OFDM.
It indicates that besides the signal-to-signal beating interference (SSBI) and beat interference between signals, there is a certain 3-order nonlinear effect, including the cross-interference term among the three signals, the square term of the signals, and cross-interference of other signals. The BER performance in the UVLC system is higher than in the VLC system. These show clearly that in the UVLC system the nonlinearity is more severe than in the traditional VLC system. Experimental results show that the VLC system can achieve lower BER at the same transmission rate, and the UVLC system has more severe nonlinearity compared to a free-space channel. Also, the DFT-S OFDM modulation format performs better in either the UVLC or VLC system.
Since nonlinearity would cause greater ISI, we also discuss the comparison of the number of taps for three formats in the UVLC and the VLC systems. Figure

Fig. 10. BER versus number of taps in the UVLC and VLC systems with (a) CAP, (b) DFT-S, and (c) OFDM.
The comparison between the UVLC system and the traditional VLC system has been studied in Figs.
We compared the BER performance using sparse pilot to do linear interpolation for channel equalization and found that the performance would deteriorate. The results are shown in Fig.
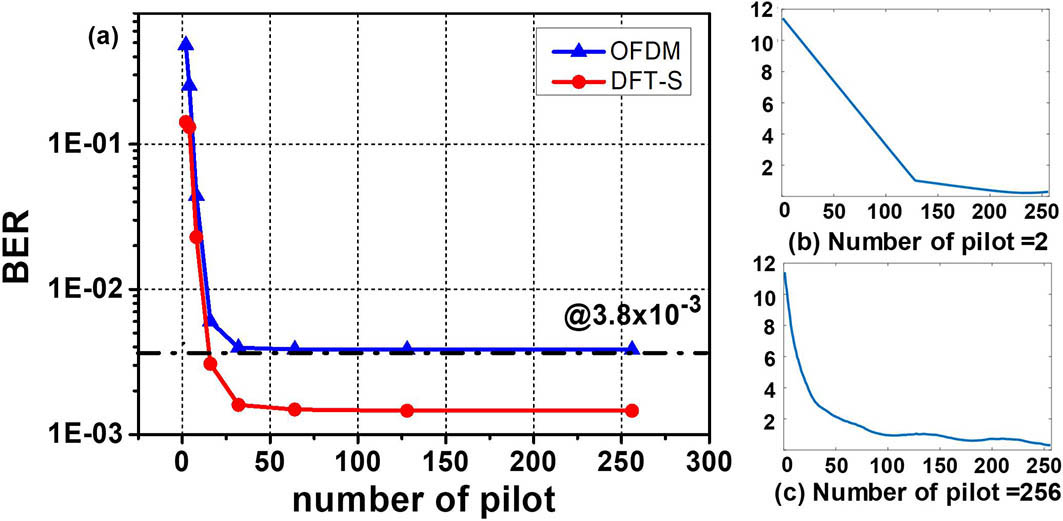
Fig. 11. BER performance of the 2nd post-equalization for OFDM and DFT-S OFDM in the UVLC system: (a) with different number of pilot, (b) channel curve when the number of pilot is 2, and (c) channel curve when the number of pilot is 256.
We compare the BER performance under different LED optical power in the UVLC system. The results are shown in Fig.
Figure
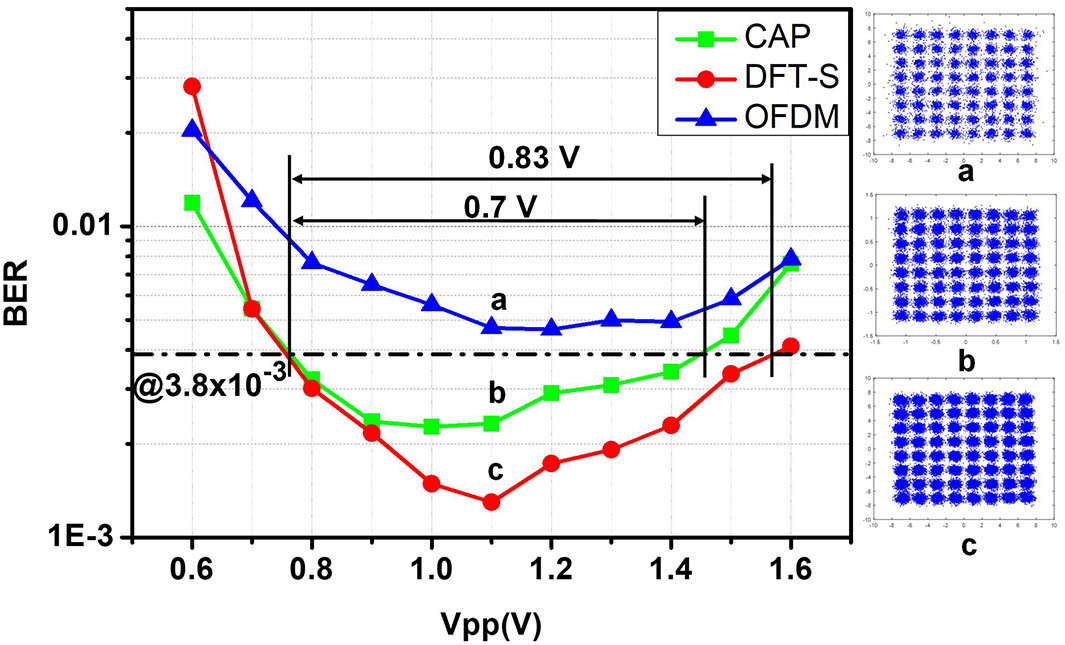
Fig. 13. BER versus peak-to-peak voltages (V pp) of the LED in CAP, DFT-S OFDM, and OFDM systems.
To investigate the influence of bandwidth, Fig.

Fig. 14. BER performance versus bandwidth of three advanced modulation formats in the UVLC system.
In this paper, a comprehensive comparison is presented among CAP-64, OFDM 64QAM, and DFT-S OFDM 64QAM with the RLS-Volterra post-equalizer in an UVLC system and traditional VLC system. We present a two-level hybrid post-equalizer to compare against both the linear distortion and nonlinear distortion. The first post-equalization is based on a novel RLS-Volterra. The performance improvement created by the first post-equalization is larger in the UVLC system compared to that in the VLC system. The UVLC system has a more severe nonlinearity than the traditional VLC system. The comparison is carried out to evaluate the performance of each modulation format in terms of PAPR, LED current, nonlinear equalization, optical power, and peak-to-peak voltage. A transmitting rate of 3.0 Gb/s over 1.2 m underwater VLC transmission is easily achieved by CAP-64 and DFT-S OFDM 64QAM. From the experimental results, DFT-S OFDM outperforms both the CAP and OFDM signals. It shows that DFT-S OFDM is a potential candidate in the future underwater VLC system.
[1] 1NakajimaA.SakoN.KamemuraM.WakayamaY.FukuzawaA.SugiyamaH.OkadaN., in Proceedings of the International Conference on Space Optical Systems and Applications (2012), p. 12.
[3] 3Le MinhH.O’BrienD.FaulknerG.ZengL.LeeK.JungD.OhY., in Proceedings of the European Conference on Optical Communication ECOC (2008), p. 1.
[4] 4GruborJ.RandelS.LangerK. D.WalewskiJ. W., in Proceedings of 6th International Symposium on Communication Systems, Networks and Digital Signal Processing CNSDSP (2008) p. 165.
[6] 6VucicJ.KottkeC.NerreterS.LangerK.-D.WalewskiJ. W., J. Lightwave Technol.28, 3512 (2010).JLTEDG0733-8724
[7] 7VucicJ.KottkeC.HabelK.LangerK.-D., in Proceedings of Optical Fiber Communication Conference (2011), paper OWB6.
[8] 8KottkeC.HiltJ.HabelK.VučićJ.LangerK.-D., in Proceedings of European Conference on Optical Communication ECOC (2012), paper We.3.B.4.
[9] 9CossuG.KhalidA. M.ChoudhuryP.CorsiniR.CiaramellaE., in Proceedings of European Conference on Optical Communication ECOC (2012), paper P4. 16.
[12] 12WuF.-M.LinC.-T.WeiC.-C.ChenC.-W.ChenZ.-Y.HuangK., in Proceedings of Optical Fiber Communication Conference OFC (2013), paper OTh1G.4.
[13] 13CossuG.WajahatA.CorsiniR.CiaramellaE., in Proceedings of European Conference on Optical Communication ECOC (2014), paper We.3.6.4.
[16] 16WangY.TaoL.HuangX.ShiJ.ChiN., IEEE Photon. J.7, 7904507 (2015).
[17] 17LiX.BamiedakisN.GuoX.McKendryJ. J. D.XieE.FerreiraR.GuE.DawsonM. D.PentyR. V.WhiteI. H., in Proceedings of European Conference on Optical Communication ECOC (2015), paper 0640.
[18] 18ChiN.ZhangM.ZhouY.ZhaoJ., Opt. Express24, 21663 (2016).OPEXFF1094-408710.1364/OE.24.021663
[19] 19ChiN.ShiJ.ZhouY.WangY.ZhangJ.HuangX., in Proceedings of Photonics Society Summer Topical Meeting Series (2016), p. 4.
[21] 21ZhuX.WangF.ShiM.ChiN.LiuJ.JiangF., in Proceedings of Optical Fiber Communications Conference and Exhibition (2018), paper M3K.3.
[22] 22ChiN.HaasH.KavehradM.LittleT. D. C.HuangX., in Proceedings of IEEE Wireless Communications (2015), p. 5.
[23] 23WangY.WangY.ChiN.YuJ.ShangH., Opt. Express21, 1203 (2013).OPEXFF1094-408710.1364/OE.21.001203
[24] 24WangZ.ZhangM.ChenS.ChiN., Chin. Opt. Lett.15, 030602 (2017).CJOEE31671-7694
[25] 25ShiM.WangF.ZhangM.WangZ.ChiN., in Proceedings of IEEE International Conference on Communications Workshops (2017), p. 337.
[26] 26QianH.YaoS. J.CaiS. Z.ZhouT., IEEE Photon. J.6, 7901508 (2014).10.1109/JPHOT.2014.2331242
[27] 27WangC.YuH.ZhuY., IEEE Photon. J.8, 7906311 (2017).
[28] 28WangY.TaoL.HuangX.ShiJ.ChiN., IEEE Photon. J.7, 7907907 (2015).
[29] 29TaoL.TanH.FangC.ChiN., in IEEE Progress in Electromagnetic Research Symposium (2016), p. 4863.
[34] 34DoniecM.VasilescuI.ChitreM.DetweilerC.Hoffmann-KuhntM.RusD., in Oceans IEEE Conference (2010), p. 1.
[35] 35CossuG.CorsiniR.KhalidA. M.BalestrinoS.CoppelliA.CaitiA.CiaramellaE., in International Workshop on Optical Wireless Communications Conference (2014), p. 11.
[39] 39DaumontS.BaselR.LouetY., in 3rd International Symposium on Communications, Control and Signal Processing (2008), p. 841.
[40] 40ElamassieM.MiramirkhaniF.UysalM., in IEEE International Conference on Communications Workshops (2018), p. 1.
[42] 42ZhouY.WangC.ZhangJ.ZhaoJ.ZengM.ChiN., in Optical Fiber Communications Conference and Exhibition (2017), paper W2A.40.
Nan Chi, Meng Shi. Advanced modulation formats for underwater visible light communications [Invited][J]. Chinese Optics Letters, 2018, 16(12): 120603.