C-band real-time 400/300 Gb/s OOK bidirectional interconnection over 20 km multicore fibers
With the increasing capacity demands fueled by the emerging heterogeneous and bandwidth-intensive applications, such as cloud computing, 4K/8K videos, and virtual reality (VR), the need for low-cost, power-efficient, and high-density short-reach optical interconnects operating at 100 Gb/s and beyond has drawn significant research efforts. Although advanced modulation formats[1,2], including multi-level pulse-amplitude modulation (PAM) and discrete multi-tone (DMT), have been frequently proposed, the simplest on-off keying (OOK) with intensity-modulation and direct-detection (IM/DD) seems to be overlooked. It has been shown that OOK can cost-effectively offer good transmission performance in short-reach (
On the other hand, as bidirectional transmission is ubiquitous in tremendously high-density fiber cable connections between servers and racks in data centers, a space congestion problem may happen. Therefore, reducing the physical footprint of the optical interconnects is especially important. Traditionally, separate optical fibers are used as full-duplex channels in a bidirectional transmission. Tempted by the advantages of high-density spatial channel count, integration compatibility, and lowcost, space division multiplexing (SDM) technologies based on novel fibers[68" target="_self" style="display: inline;">–
In this work, we further utilized the MCF-SDM scheme to demonstrate a quasi-full-duplex 400 Gb/s C-band OOK bidirectional transmission over a 20 km seven-core fiber, using 10G-class transceivers without any DSP or electrical equalization. To address the frequency selective power fading induced by the laser chirp and fiber dispersion, an optical delay interferometer (ODI) is employed as an optical equalizer for all four wavelength channels, which enables open and clear eyes even after a 20 km MCF transmission.
As shown in Fig.
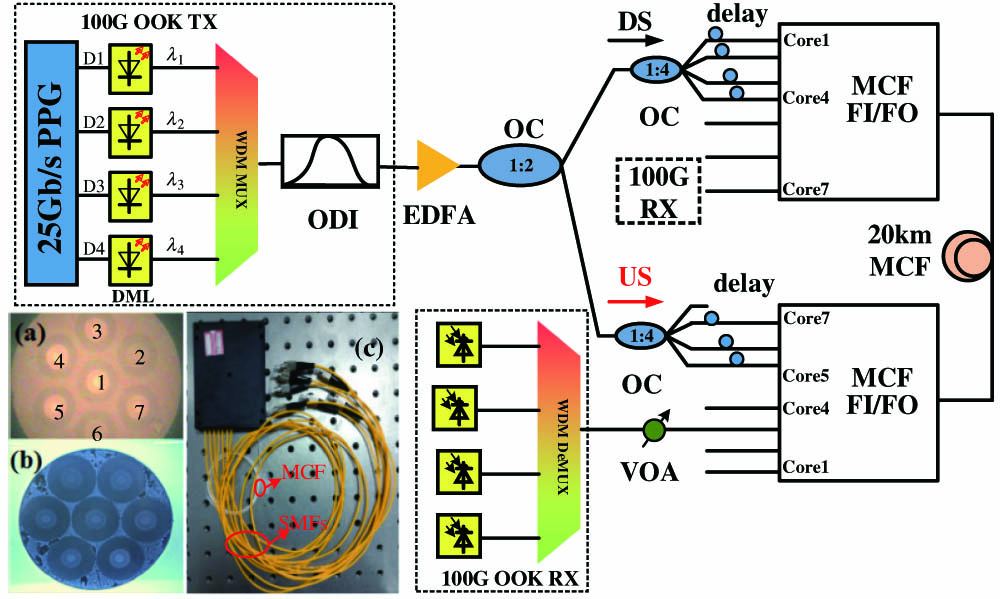
Fig. 1. Experimental setup of bidirectional MCF transmission system. The insets (a), (b), and (c) are the cross section view of the fabricated MCF, fan-in/fan-out, and picture of the whole fan-in/fan-out device, respectively. (OC, optical coupler; FI/FO, fan-in/fan-out; RX, receiver; Mux, multiplexer; DeMux, demultiplexer.)
After 20 km MCF transmission, the spatial channels are demultiplexed by another fan-in/fan-out device so that the 100 Gb/s OOK signal in each core can be adapted to an SMF. For each of the spatial channels, a WDM demultiplexer follows to separate and extract the individual 25 Gb/s spectral channels. In order to adjust the received optical power (ROP), a variable optical attenuator (VOA) is inserted before the receiver. For each wavelength, a 10G-class PIN photodetector (PD) is used to detect the 25 Gb/s per
During the experiments, first, we measured the end-to-end insertion loss of our MCF link, including a pair of fan-in/fan-out devices and 20 km MCFs. The main parameters for the design and fabrication of the MCF and fan-in/fan-out can be found in our previous work[12]. With an optimized manufacturing process, the performances of our fabricated seven-core fiber and fan-in/fan-out devices have been improved a lot. The average loss per core is about 7 dB, and the inter-core crosstalk is less than −45 dB per 100 km, which is low enough for SDM transmission. The chromatic dispersion parameter of our fabricated MCF is comparable with that of an SMF and other optical parameters, including zero dispersion wavelength, mode field diameter, and bending loss, which can be found in our recent work[17].
Then, we turned on DML 1, while keeping the other three DMLs off, and measured the optical spectra of the DS OOK signals with and without ODI in the optical back-to-back (OB2B) case. With careful alignment of the center wavelength of the DML, one of the notch bands of the ODI can be well-fitted to the long wavelength sideband of the OOK signal. Of course, this procedure can be further optimized to acquire a better eye diagram. As shown in Fig.
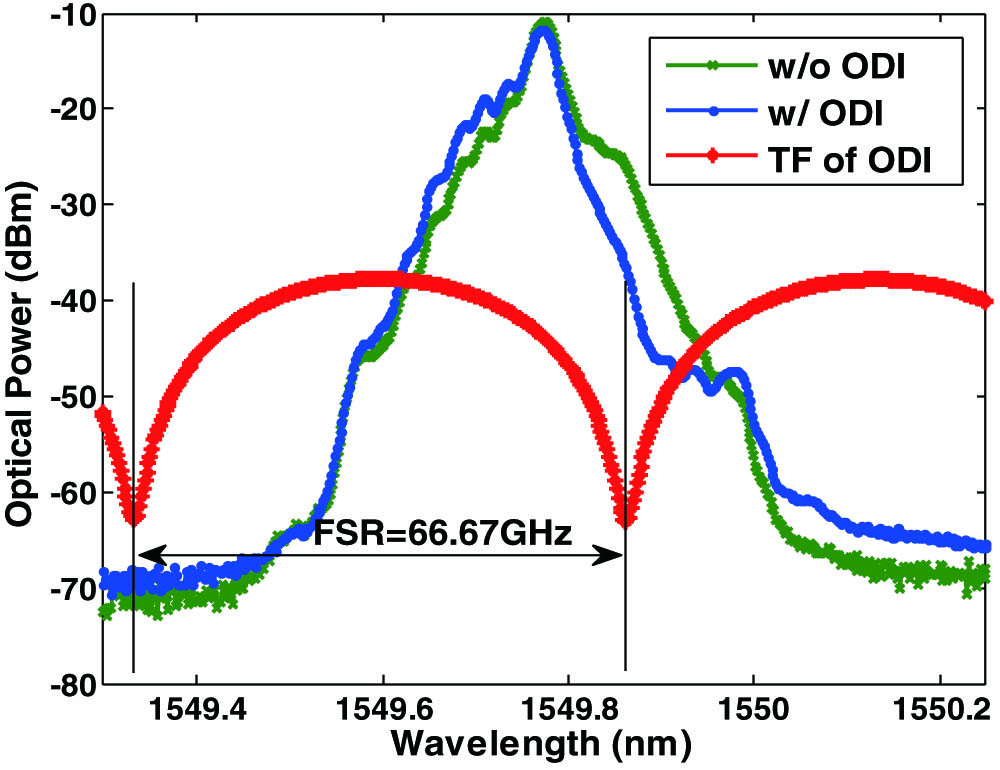
Fig. 2. (Color online) Spectrum of 25 Gb/s OOK signal generated by DML1 with and without ODI, the transmission function of the ODI is also depicted. (w/o, without; w/, with; TF, transmission function.)
To further demonstrate the frequency equalization effect of the ODI, we also measured the OB2B frequency response of the transceivers with and without ODI using a vector network analyzer (VNA). Figure

Fig. 3. (Color online) Frequency response of the transceivers with and without ODI. (BW, bandwidth.)
Subsequently, we turned on all the four DMLs with the channel spacing setting to 200 GHz and then modulated them with independent 25 Gb/s OOK signals under the condition of a proper bias current and driving voltage. Optical spectra of the four wavelength multiplexed 100 Gb/s OOK signals after ODI spectral shaping are shown in Fig.

Fig. 4. Optical spectra of the WDM multiplexed 100 Gb/s OOK signals after ODI filtering.
In order to evaluate the transmission performance of the whole system, we first checked the role of ODI on the eye diagram of OB2B and MCF transmission. As shown in Fig.
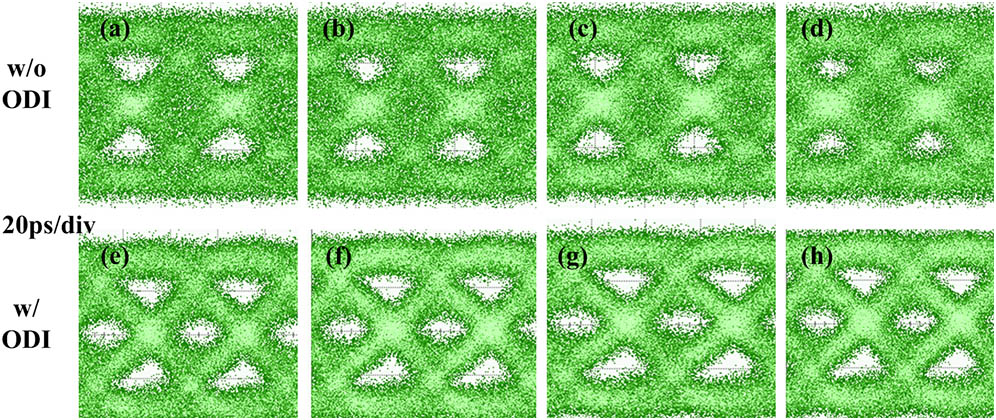
Fig. 5. Measured eye diagrams for the OB2B case with and without ODI. (a) (d) are for without ODI, and (e) (h) are for with ODI, respectively.

Fig. 6. Measured eye diagrams of 25G OOK signals after 20 km MCF transmission. (a) in core 1 for the DS direction without ODI, (b) in core 5 for the US direction without ODI, (c) in core 1 for the DS direction with ODI, (d) in core 5 for the US direction with ODI.
Lastly, we investigated the BER performance versus different ROPs for the bidirectional transmission system when ODI is used in the TX. Cores 1 to 4 are used for DS, while cores 5 to 7 are for US transmission. As a result, the aggregate data rate per fiber is 700 Gb/s, in which 400 Gb/s (

Fig. 7. (Color online) BER performances of 100G OOK signals for different cores in bidirectional MCF transmission. (a)–(d) are for DS (core 1 and 2) and US (core 5 and 6), respectively. ( : .)

Fig. 8. Receiver sensitivity variation among different fiber cores and wavelengths. ( : .)
In conclusion, taking advantage of spatial channel multiplexing over wavelength stacking, we experimentally demonstrate a real-time quasi-full-duplex 400G/300G optical interconnection over 20 km MCFs, using only 10G-class transponders operated in the C-band. To address the bandwidth limited problem, which can be further aggravated by chirp and dispersion, we utilize a single ODI as an optical frequency equalizer for all four wavelength channels simultaneously. With this technique, the 3 dB bandwidth of transponders can be greatly extended, and the quality of the eye diagrams can be significantly improved. Thanks to the ODI-based chirp management and frequency equalization, 100 Gb/s per fiber core transmission can be realized with a receiver sensitivity of about
With the emerging of heterogeneous and bandwidth-intensive applications driven by the upcoming era of 5G and internet of things (IOT), large-capacity short-reach optical interconnection operating at 100 Gb/s and beyond is highly desired. To support such a scenario, inherently high-density and potentially cost-efficient SDM technologies are indispensable. Considering the space congestion problem, which may happen among the massive connections between servers and racks in data centers, the MCF will play as an important and promising transmission media to support high-density bidirectional optical interconnection. With the development of low-crosstalk MCF and compact low-loss fan-in and fan-out devices, more spatial channels can be feasible in one pipeline, thus, it emerges as an ideal platform for future data-driven multi-service featured high-density optical interconnections. As an alternative, FMF is also a promising physical medium for big-data services transmission with technical progress on low-crosstalk FMF fabrication and low-loss mode multiplexing and demultiplexing techniques. Ultimately, dense SDM technologies using multicore FMFs are expected to further enhance the capacity by orders of magnitude, supporting even larger bandwidth transmissions with a lower system cost. For the short-range transmission scenario, the use of 10G-class transceivers is also important to control the system cost while upgrading the capacity. It is envisaged that the extensively studied PAM4 transmission techniques can be easily applied to our MCF system to further improve the DS/US capacity to 800 Gb/s with the help of some essential DSP algorithms during PAM4 signal modulation and demodulation.
[1]
[2]
[4]
[5]
[6]
[8]
[9]
[10]
[11]
[12]
[13]
[14]
[15]
[16]
[17]
[18]
[19]
Zhenhua Feng, Honglin Ji, Ming Tang, Lilin Yi, Lin Gan, Lei Xue, Qiong Wu, Borui Li, Jiajia Zhao, Weijun Tong, Songnian Fu, Deming Liu, Weisheng Hu. C-band real-time 400/300 Gb/s OOK bidirectional interconnection over 20 km multicore fibers[J]. Chinese Optics Letters, 2017, 15(8): 080602.