BaTiO3-ZnNb2O6陶瓷介电及储能性能研究
钛酸钡(BaTiO3)是一种典型的ABO3型钙钛矿结构的铁电体材料, 因其优良的介电、压电以及铁电性能[1]被广泛应用于多层陶瓷电容器[2], 铁电存储器[3], 传感器及非线性电光器件等[4,5]。BaTiO3 (BT)陶瓷介电常数(εr)非常高, 室温为1500~2000 ℃, 在居里温度(TC)附近高达10000 ℃以上[6], εr与温度呈非线性关系, 导致εr随温度变化率较大, 此外εr对电场、频率和压力等条件变化也非常敏感。BT陶瓷介电损耗(tanδ >0.05)相对较高。BT陶瓷饱和极化强度值(Ps >27 μC/cm2)高[7], 而剩余极化强度值(Pr > 14.7 μC/cm2)也很高[8]。BT陶瓷击穿电场(BDS< 100 kV/cm)较低[9]。
高εr和大Ps使BT陶瓷成为一种非常有潜力的无铅陶瓷储能电容器材料[10,11,12], 可应用于激光脉冲**和混合电动车等领域[13,14,15,16,17,18,19]。但高Pr和低BDS导致BT陶瓷储能性能并不良好[20,21,22]。近年来许多研究人员尝试在BT的A位和B位引入离子合成BT基弛豫铁电体陶瓷从而降低Pr: Huang等[23]用Sol-Gel法合成Ba0.4Sr0.6TiO3陶瓷, 在240 kV/cm电场强度下获得1.23 J/cm3能量存储密度(W), 和94.52%能量存储效率(η)。Puli等[24]用固相法制备0.85Ba(Zr0.2Ti0.8)O3-0.15(Ba0.7Ca0.3)TiO3陶瓷, 在170 kV/cm下获得0.94 J/cm3的W和94.52 %的η。Sun等[25]用固相法合成Ba1-xSm2x/3Zr0.15Ti0.85O3陶瓷, 当x=0.003获得1.15 J/cm3可释放能量密度(Wrec)和92%的η。此外, 在BT中加入Bi基化合物合成BT-Bi基弛豫铁电体陶瓷成为近几年研究热点: Wang等[26]用固相法合成(1-x)BaTiO3-xBi(Mg2/3Nb1/3)O3陶瓷, 当x=0.1在143.5 kV/cm下获得1.13 J/cm3的Wrec和92.4%的η。Hu等[27]用固相法合成0.88BaTiO3- 0.12Bi(Mg1/2Ti1/2)O3陶瓷, 在224 kV/cm下获得1.81 J/cm3的Wrec和88%的η。Yuan等[28]用固相法合成0.9BaTiO3-0.1Bi(Zn0.5Zr0.5)O3陶瓷, 在264 kV/cm下获得2.46 J/cm3的Wrec和86.8%的η。Li等[29]用固相法合成0.88BaTiO3-0.12Bi(Li0.5Nb0.5)O3陶瓷, 在270 kV/cm下获得2.03 J/cm3的Wrec和88%的η。上述BT基和BT-Bi基弛豫铁电体陶瓷Pr均随着离子化合物含量增加逐渐减小, 储能性能得到明显改善, 然而Ps急剧降低成为储能性能提高的瓶颈。
为了提高BT基陶瓷BDS, 许多研究人员在BT基或BT-Bi基陶瓷中添加玻璃来提高致密度, 同时达到降低烧结温度的效果。Wang等[30]在BT陶瓷中添加BaO-SrO-TiO2-Al2O3-SiO2-BaF2玻璃, 当玻璃添加量为7wt%, BDS提高到94.6 kV/cm, 获得0.32 J/cm3的Wrec。Wang等[31]在Ba0.4Sr0.6TiO3陶瓷中添加BaO-B2O3-SiO2-Na2CO3-K2CO3玻璃, BDS提高到280.5 kV/cm, 获得0.72 J/cm3的Wrec。Yang等[32]在Ba0.4Sr0.6TiO3 陶瓷中添加Bi2O3-B2O3-SiO2玻璃, 当玻璃添加量为9wt%, BDS提高到279 kV/cm, 获得1.98 J/cm3的Wrec和90.57%的η。Yang等[33]在Ba0.85Ca0.15Zr0.1Ti0.9O3陶瓷中添加B2O3-Al2O3-SiO2玻璃, BDS提高到250 kV/cm, 当玻璃添加量为5wt%, 获得1.15 J/cm3的Wrec。Wu等[34]在Ba0.4Sr0.6Zr0.15Ti0.85O3陶瓷中添加SrO-B2O3-SiO2玻璃, BDS提高到220 kV/cm, 当玻璃添加量为5wt%, 获得0.45 J/cm3的Wrec和88.2%的η。Wang等[35]在0.85BaTiO3-0.15Bi(Mg2/3Nb1/3)O3陶瓷中添加B2O3-Na2B4O7-Na2SiO3玻璃, BDS提高到240 kV/cm, 当玻璃添加量为3wt%, 获得1.26 J/cm3的Wrec和80.9%的η。上述BT基和BT-Bi基玻璃陶瓷BDS均随着玻璃含量增加而增大, 但同样存在玻璃含量高时Ps大幅降低现象。此外在BT陶瓷中添加低温晶相助烧剂也可改善BT基陶瓷BDS。铌酸锌(ZnNb2O6)[36]是一种典型低温烧结微波介质陶瓷。Wang等[37]用固相法合成BaTiO3-ZnNb2O6 (BT-ZN)陶瓷, 结果表明添加ZN显著降低BT陶瓷烧结温度。Yan等[38]和Yang等[39]分别用固相法和微波烧结法制备BT-ZN陶瓷, 结果表明添加ZN并未明显改变BT陶瓷TC。上述研究发现添加ZN使BT陶瓷BDS和储能性能都得到改善, 但均未系统性研究ZnNb2O6含量对BT陶瓷介电常数频率稳定性和温度稳定性, 以及对极化强度值和储能性能的影响。
本工作用固相法制备了BaTiO3-ZnNb2O6 (BT-ZN)陶瓷, 系统地研究了ZnNb2O6含量对BT-ZN陶瓷烧结温度、相结构、显微结构、介电常数的频率稳定性和温度稳定性、击穿电场、极化强度值和储能性能的影响。
1 实验方法
1.1 样品制备
以分析纯BaCO3、TiO2、ZnO和Nb2O5为原料, 固相法制备(1-x)BaTiO3-xZnNb2O6(x=0.5mol%, 1mol%, 1.5mol%, 2mol%, 3mol%, 4mol%) (BTZN1-6)陶瓷。先分别称料BaCO3、TiO2、ZnO和Nb2O5, 球磨6 h, 然后分别在1150和900 ℃煅烧2 h合成BT和ZN预烧粉, 并将预烧粉二次球磨。将BT和ZN预烧粉按配比称料球磨6 h, 干燥后加入PVA (5wt%)造粒, 在150 MPa下压制成直径12 mm, 厚度1~ 2 mm圆片生坯。600 ℃排胶4 h后在1100~ 1350 ℃下烧结2 h成瓷。用被银法在850 ℃保温20 min制备厚度1 mm的介电性能测试样品。用喷金法制备厚度0.3 mm的铁电性能测试样品, 电极直径为6 mm。
1.2 性能测试
采用阿基米德排水法测试样品密度。采用X射线衍射仪(XRD, D8 Advance, Bruker, Germany)测试样品相结构。采用扫描电子显微镜(SEM, Verios 460, FEI, USA)观察抛光热腐蚀样品表面显微结构。采用阻抗分析仪(E4990A, Aglient, USA)测试样品介电性能频率稳定性, 频率范围为100 Hz~10 MHz。采用LCR电桥(E4980A, Aglient, USA)测试样品介电性能温度稳定性, 温度范围为-100~500 ℃, 升温速率3 ℃/min, 测试频率为100 Hz、1 kHz、10 kHz、100 kHz和1 MHz。采用铁电测试仪(Premier II, Radiant, USA)测试样品室温下电滞回线, 测试频率为10 Hz。
2 结果和讨论
2.1 BTZN陶瓷的结构分析
图1是BTZN陶瓷不同烧结温度的密度, 插图为不同ZN含量BTZN陶瓷最佳烧结温度和密度, BTZN陶瓷最佳烧结温度随ZN含量增加从1275 ℃ (BTZN1)降低到1200 ℃(BTZN6)。纯BT陶瓷烧结温度高达1375 ℃[3], 而ZN陶瓷烧结温度仅有1150 ℃[36], 说明添加ZN有效降低了BTZN陶瓷烧结温度。而最佳烧结温度下BTZN陶瓷密度随ZN含量增加从5.808 g/cm3(BTZN1)降低到5.701 g/cm3 (BTZN6), 主要由于ZN陶瓷的理论密度(5.645 g/cm3)比BT陶瓷(6.018 g/cm3)低[37]。
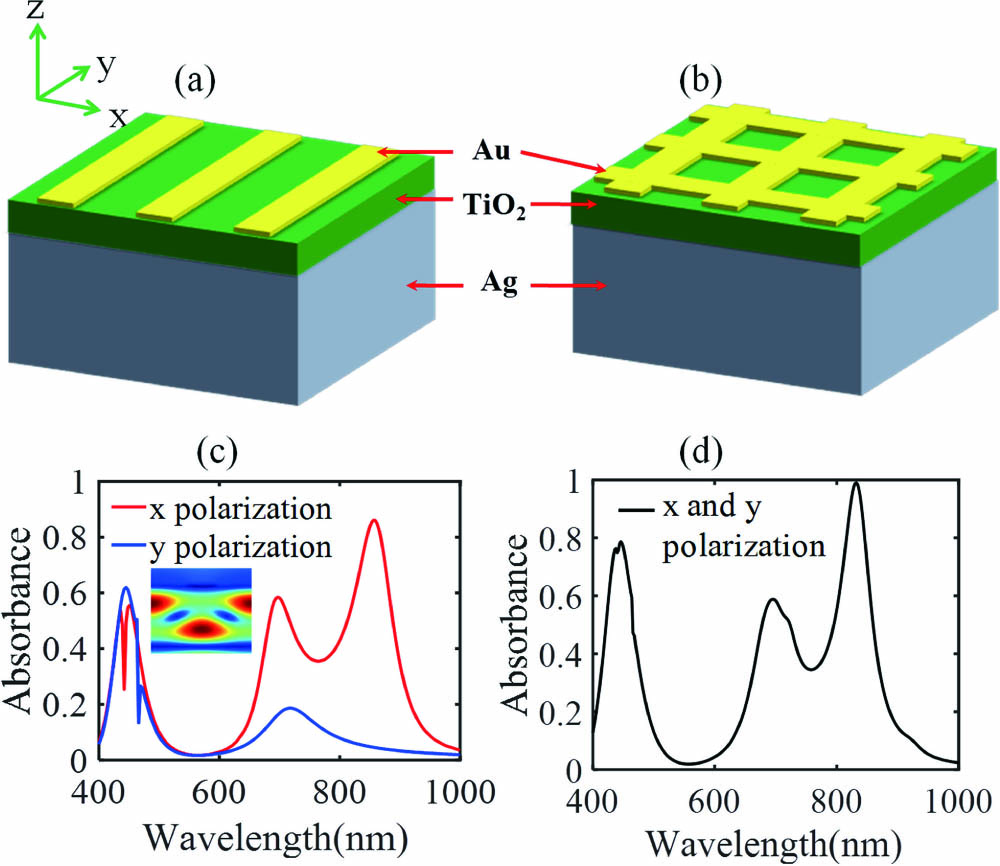
图 1. BTZN陶瓷不同烧结温度的密度, 插图为不同ZN含量BTZN陶瓷最佳烧结温度和密度
Fig. 1. Density as a function of sintering temperature for BTZN ceramics with inset showing the optimum sintering temperature and density of BTZN ceramics with different ZN content
图2(a)是BTZN陶瓷XRD图谱, 结果表明所有样品主晶相衍射峰为BaTiO3(No. 050626)。当ZN含量达到3mol%时出现ZnNb2O6(No. 371371)和第二相Ba2Ti5O12(No. 170661)的衍射峰[37]。
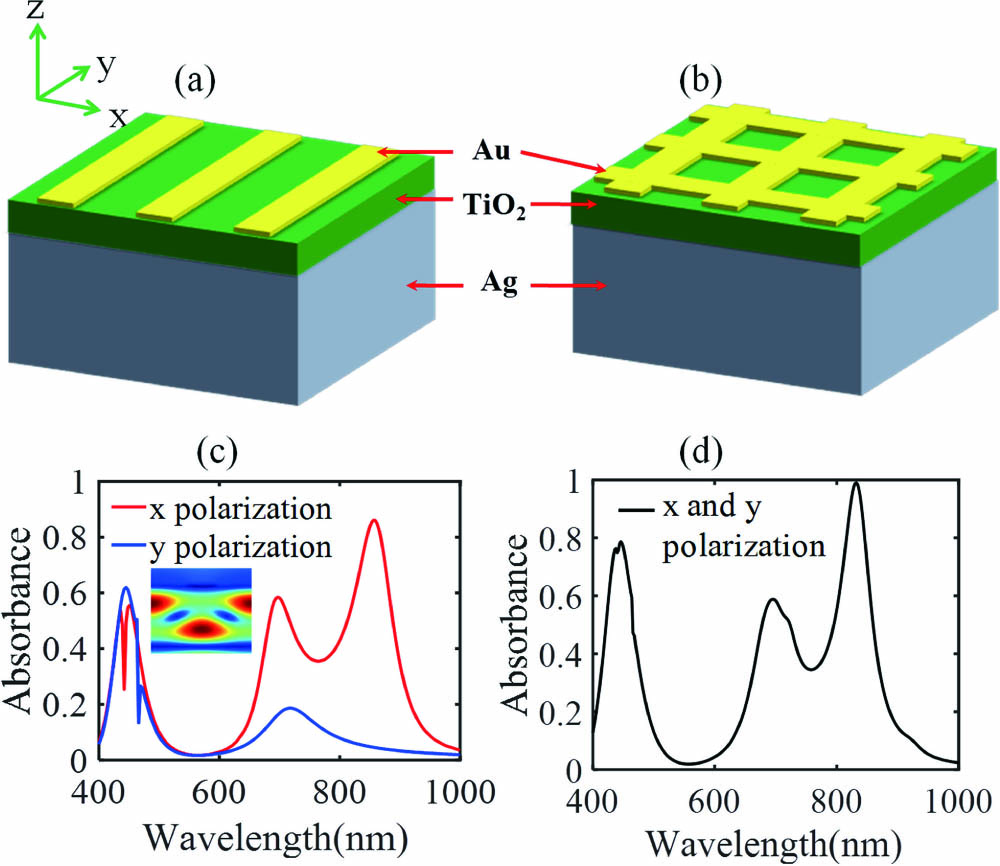
图 2. (a)BTZN陶瓷XRD图谱和(b)31°~32°放大图谱
Fig. 2. (a) XRD patterns of BTZN ceramics and (b) enlarged XRD patterns from 31° to 32° of BTZN ceramics
图2(b)是BTZN陶瓷31°到32°放大图谱, 衍射峰随ZN含量增加向低角度方向移动, 说明晶面间距变大。通常容差因子t在0.79~1.1可形成稳定钙钛矿结构, 容差因子计算公式[40]:
式(1)中: rA——A位阳离子半径; rB——B位阳离子半径; rO——氧离子半径。Zn2+取代A位和B位容差因子分别为0.728和0.909, 而Nb5+分别为0.718和0.926。Zn2+(0.074 nm)和Nb5+(0.070 nm)远小于A位Ba2+(0.135 nm), 比B位Ti4+(0.068 nm)略大。所以Zn2+和Nb5有可能取代B位Ti4+, 增大B-O八面体体积, 导致晶面间距增大。
图3是BTZN陶瓷的抛光热腐蚀SEM照片, 从图中可见所有样品均很致密, 晶粒尺寸随ZN含量增加显著减小, 说明ZN抑制BTZN陶瓷晶粒生长, 晶粒尺寸减小非常有利于提高陶瓷BDS[35]。
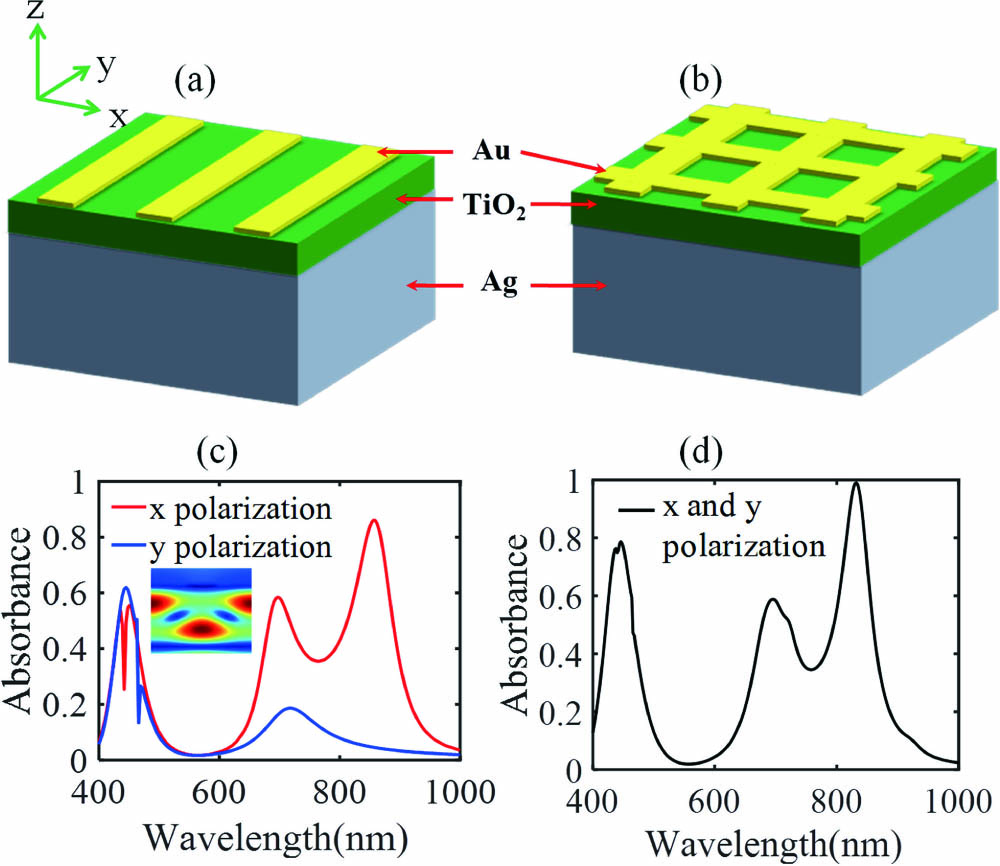
图 3. BTZN陶瓷热腐蚀的SEM照片(a) BTZN1; (b) BTZN2; (c) BTZN3; (d) BTZN4; (e) BTZN5; (f) BTZN6
Fig. 3. SEM images of polished and thermally etched BTZN ceramics
2.2 BTZN陶瓷的介电性能
图4(a, b)是BTZN陶瓷介电常数(εr)和介电损耗(tanδ)随频率变化的曲线, BTZN陶瓷εr和tanδ都随ZN含量增加逐渐降低。100 Hz下εr从3923 (BTZN1)降低到1604(BTZN6), tanδ从0.013(BTZN1)减小到0.006(BTZN6), 10 MHz下所有样品tanδ <0.05, 这是由于ZN的εr和tanδ都远远低于BT[36]。所有BTZN陶瓷εr随频率升高逐渐降低, 是由于不同极化机制响应频率不同造成的。低频下电子位移极化、离子位移极化和偶极子取向极化等各种机制都对εr有贡献, 高频时只有电子位移极化和离子位移极化机制[35]。BTZN1陶瓷εr随频率升高降低较为明显, 随ZN含量增加, BTZN陶瓷εr表现出良好的频率稳定性, 说明ZN显著减弱了BTZN陶瓷介电极化。
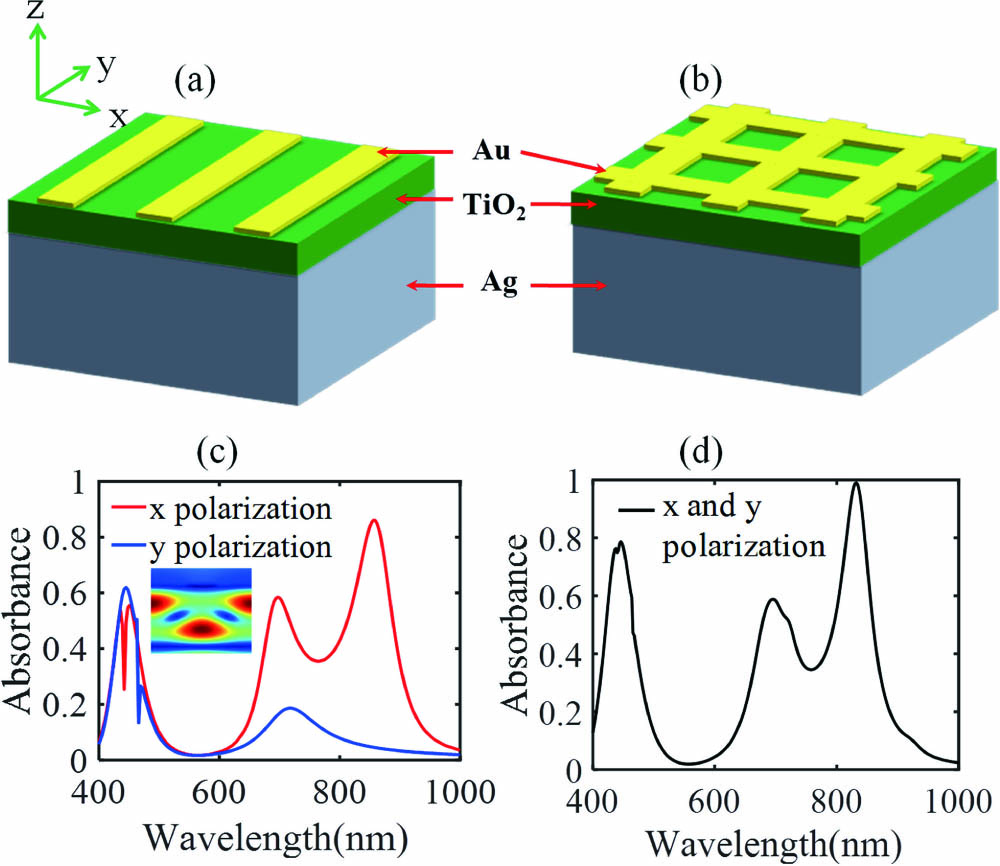
图 4. BTZN陶瓷的介电性能频率稳定性
Fig. 4. Frequency stability of dielectric properties for BTZN ceramic
为了进一步研究ZN含量对BTZN陶瓷εr随频率变化的影响, 我们定义了一个公式:
式(2)中: εr(f)——频率为f时的εr; f——测试频率。图4(a)中插图是线性拟合斜率a和截距b的拟合值与ZN含量关系。随ZN含量增加, 斜率a从114.7(BTZN1)减小到26.6(BTZN6), 说明εr随频率变化率越来越小。截距b和εr减小规律一致, 从4195(BTZN1)减小到1670(BTZN6)。
我们通过定义频率电容系数(Frequency coefficient of capacitance, FCC)来描述BTZN陶瓷εr频率稳定性:
式(3)中: C100 Hz——频率为100 Hz时C; Cf——频率为f时C。图4(c)是FCC随频率变化, 所有BTZN陶瓷FCC均随频率升高逐渐减小, 是由于εr随频率升高逐渐减小。为了进一步描述ZN含量对FCC影响, 图4(d)是FCC随ZN含量变化曲线, 在相同测试频率下FCC均随ZN含量增加逐渐增大, 10 MHz下FCC从-14.85%(BTZN1)提高到-8.45%(BTZN6), 说明ZN添加有利于提高BTZN陶瓷εr频率稳定性。
图5是BTZN陶瓷在-100~500 ℃范围内的介电常数和介电损耗与温度的关系。随着ZN含量增加, BTZN陶瓷居里温度(TC)并没有明显变化, 而介电常数居里峰展宽较为明显。Yang等[39]采用TEM在BTZN陶瓷晶粒和晶界处都发现了Zn和Nb元素, 说明ZnNb2O6和BaTiO3形成了固溶体。但晶界处Zn和Nb元素含量明显高于晶粒内部, 且观察到BTZN陶瓷存在壳核结构。由于壳核结构的形成和Zn2+和Nb5+取代B位Ti4+造成BTZN陶瓷的化学成分不均匀, 引起弥散相变导致居里峰展宽[38]。TC处最大介电常数(εm)随ZN含量增加逐渐减小, 1 MHz下εm从3956(BTZN1)降低到1694(BTZN6), 主要是由于ZN的εr低于BT[36]。TC和εm的变化和其他在BT中添加ZN的文献报道结果一致[38,39]。用电容温度系数(Temperature Coefficient of Capacitance, TCC)来进一步研究BTZN陶瓷εr的温度稳定性[35, 41-42]:
式(4)中: C25 ℃——25 ℃时的电容; CT——温度T时的电容。
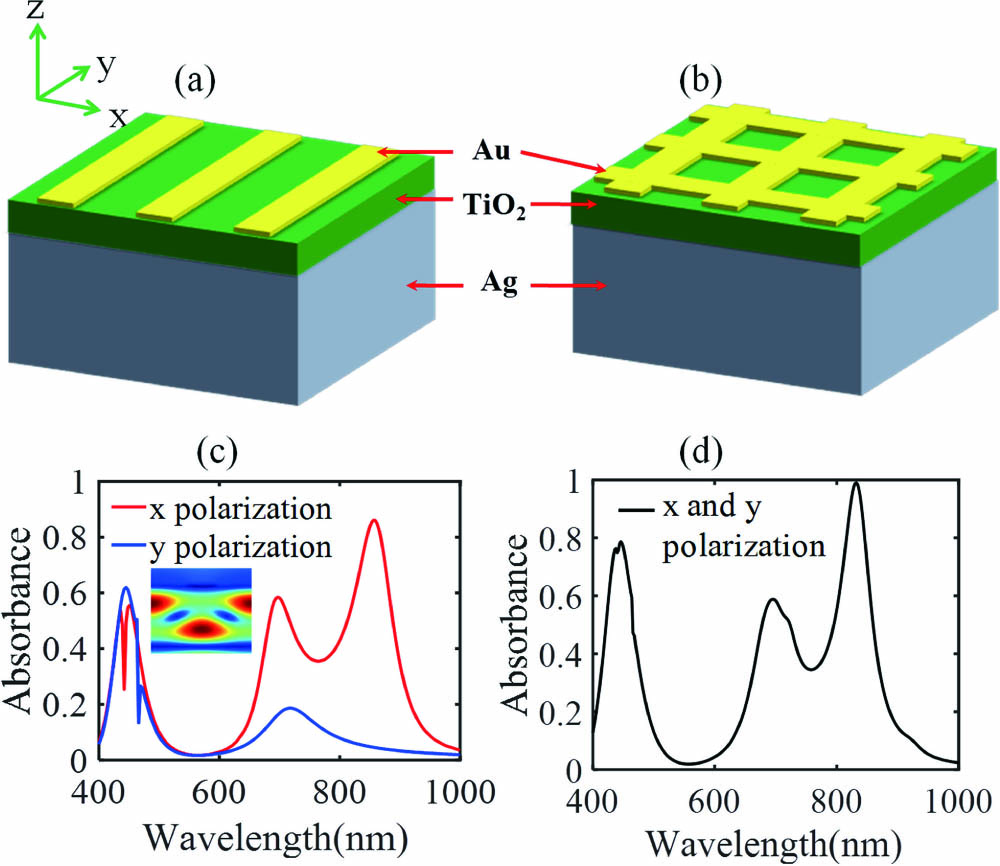
图 5. BTZN陶瓷-100~500 ℃的介电常数和介电损耗
Fig. 5. Temperature dependence of dielectric constant and loss of BTZN ceramics from -100 ℃ to 500 ℃
图6是BTZN陶瓷基于25 ℃在1 MHz下的TCC, 虚线表示TCC范围是±15%, 25 ℃的TCC为0, 位于两条虚线中间。从图中可以看出, 所有BTZN陶瓷均满足X8R(-55~150 ℃, ΔC/C25 ℃≤±15%)[43]电容器标准。
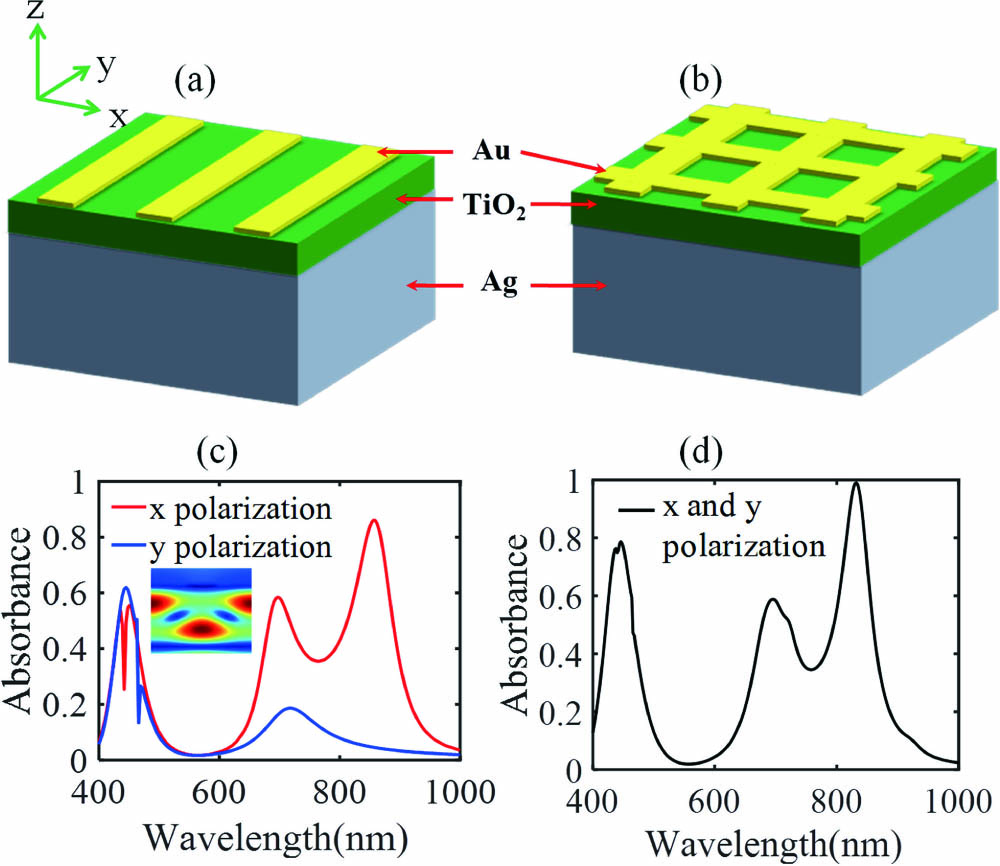
图 6. BTZN陶瓷1 MHz下基于25 ℃的TCC
Fig. 6. TCC of the BTZN ceramics at the frequency of 1 MHz and a base temperature of 25 ℃
2.3 BTZN陶瓷的储能性能
图7是BTZN陶瓷击穿电场下的室温电滞回线(10 Hz)。所有样品表现出瘦电滞回线, 高BDS, 大Ps和低Pr, 适合应用于能量存储领域[26]。插图是不同ZN含量的BTZN陶瓷的BDS, BDS随ZN含量增加而增大。从80 kV/cm(BTZN1)增大到240 kV/cm(BTZN6), BDS的提高主要是由于晶粒尺寸减小(图3)。
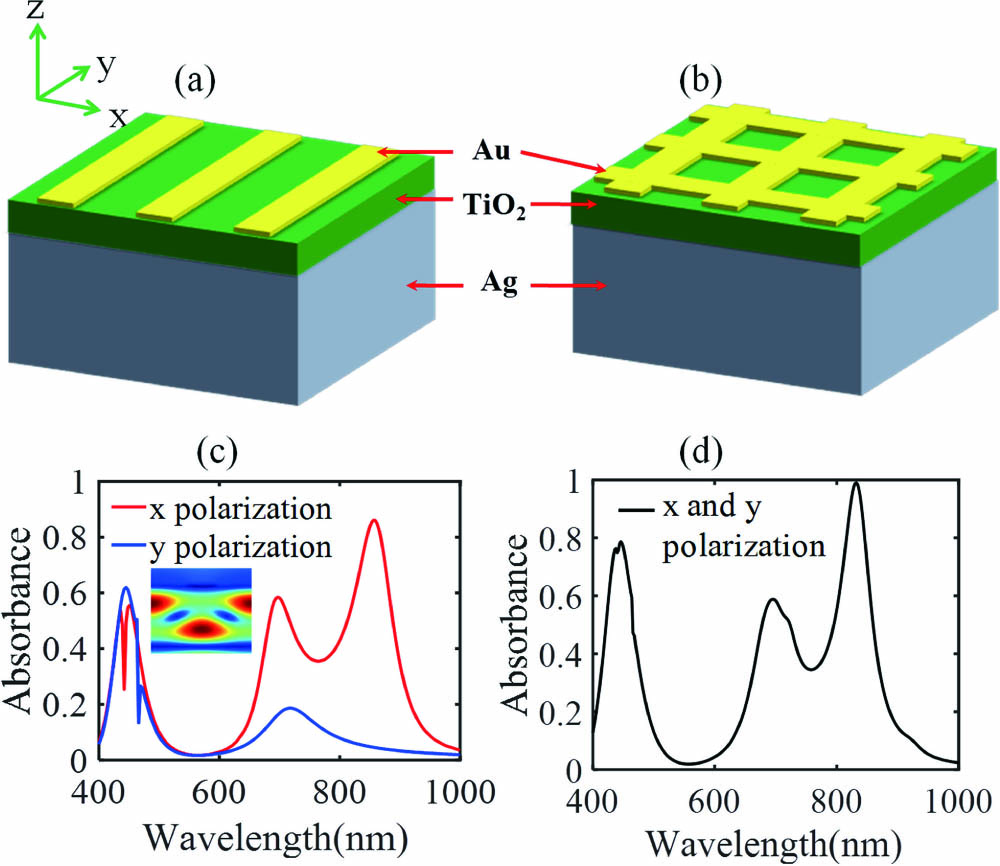
图 7. BTZN陶瓷击穿电场下室温电滞回线(10 Hz), 箭头方向为ZN含量增大方向, 插图为不同ZN含量BTZN陶瓷BDS
Fig. 7. P -E loops of BTZN ceramics at critical electric field, room temperature and 10 Hz with direction of the arrow indicating the direction in which the ZN content increases with inset showing the BDS of BTZN ceramics with different ZN contents
图8(a)是不同ZN含量BTZN陶瓷在100 kV/cm下(最大极化强度值)的Pmax、Pr和Pmax-Pr (BTZN1陶瓷的BDS为80 kV/cm, 所以并未出现)。Pmax、Pr和Pmax-Pr 均随着ZN含量增加逐渐减小。Pmax和Pr分别从20.26和4.48 μC/cm2(BTZN2)减小到11.67和1.37 μC/cm2(BTZN6), 这是由于添加ZN稀释了BTZN陶瓷的铁电性, 和其他BT-ZN陶瓷的结果一致[37,38,39]。用P-E曲线研究BTZN陶瓷储能性能, 曲线由于存在滞回, 充电曲线和放电曲线并不重合。能量存储密度(W)是充电电流曲线和极化强度轴包围的面积积分, 可释放能量密度(Wrec)是放电电流曲线和极化强度轴包围的面积积分, 损失掉能量密度(Wloss)是充放电电流曲线包围的面积积分, 能量存储效率(η)是Wrec/W。因此W, Wrec, Wloss和η都是衡量能量存储性能的重要指标[35]:
式(5~8)中: E——电场强度; P——极化强度值。
图8(b)~(e)是BTZN陶瓷不同电场强度下W, Wrec, Wloss和η。随ZN含量增加W, Wrec和Wloss都逐渐增加, 而η逐渐减小, 主要由于Wloss随电场强度增加显著增大[26, 35]。实际应用不仅需要高Wrec, 同时高η也是必须的, 低η材料在充放电过程中会将大量电能转换成热能, 导致材料性能恶化[35]。当ZN的含量为4mol%时, 不同电场下η均高于77.6%。图8(f)是不同ZN含量BTZN陶瓷在各自BDS下的W, Wrec, Wloss和η, 随ZN含量增加W, Wrec, Wloss和η大体呈增大趋势, 当ZN含量4mol%, BTZN6陶瓷在240 kV/cm电场下获得1.22 J/cm3的Wrec和77.6%的η。
3 结论
本研究采用固相法制备(1-x)BaTiO3-xZnNb2O6 (x=0.5mol%, 1mol%, 1.5mol%, 2mol%, 3mol%, 4mol%)陶瓷。添加ZN有效降低了BTZN陶瓷的最佳烧结温度。XRD结果表明所有样品主晶相为BaTiO3, 当ZN含量达到3mol%时出现ZnNb2O6和第二相Ba2Ti5O12。BTZN陶瓷晶粒尺寸随ZN含量增加显著减小。εr和tanδ随ZN含量增加逐渐降低, 100 Hz下εr从3923降低到1604, tanδ从0.013减小到0.006, 10 MHz下所有样品tanδ <0.05。εr频率稳定性随ZN含量增加逐渐增强, 10 MHz下FCC从-14.85%提高到-8.45%。介电温谱结果表明所有BTZN陶瓷均符合X8R电容器标准。BDS随ZN含量增加而增大, 从80 kV/cm增大到240 kV/cm。极化强度值随着ZN含量的增加逐渐降低, 100 kV/cm下Pmax和Pr分别从20.26和4.48 μC/cm2减小到11.67和1.37 μC/cm2。随ZN含量增加W, Wrec, Wloss和η大体上呈增大趋势, 当ZN含量为4mol%时, BTZN6陶瓷在240 kV/cm电场下获得1.22 J/cm3的Wrec和77.6%的η。结果表明BTZN陶瓷有望应用于高温电容器和储能领域。
王通, 王渊浩, 杨海波, 高淑雅, 王芬, 鲁雅文. BaTiO3-ZnNb2O6陶瓷介电及储能性能研究[J]. 无机材料学报, 2020, 35(4): 431. Tong WANG, Yuanhao WANG, Haibo YANG, Shuya GAO, Fen WANG, Yawen LU.