On-line beam diagnostics based on single-shot beam splitting phase retrieval
Download: 714次
In a high-power laser system, the quality of the laser beam performs an important role[1]. However, due to the complexity of high-power laser systems containing hundreds of optical elements, the quality of the laser beam may be influenced by the material defects, manufacturing error, gas density variations, thermal distortion, and dust in the environment, which will lead to the deformation of the wave-front and failure of the experiment. The traditional beam diagnostics method includes the interferometers[2] and Shack–Hartmann[3,4] sensors, where the interferometers have high accuracy, but their complex structure and high demands of the environment make them difficult to measure the on-line beam quality. The Shack–Hartmann sensors measure the wave-front by dividing it into subbeams with arrays of microlenses and analyzing focal spot displacement[5], and it has been applied successfully in the OMEGA extended performance (EP) laser system to measure the focal spot at full energy[6]. However, the resolution of the Shack–Hartmann sensor is limited by its finite number of microlenses[7]. Phase retrieval is an effective technique for estimating the wave-front based on a series of intensity measurements[8,9]. It has been applied to the high-energy laser system focal spot characterization[10]. The phase retrieval method contains a variety of algorithms like the Gerchberg–Saxton (G-S) algorithm[11], the Yang–Gu algorithm[12], the Eerror-reduction (ER) algorithm[13], and the hybrid input–output (HIO) algorithm[14]. In 2010, Zhang proposed the coherent modulation imaging (CMI) algorithm[15,16], which uses only one diffraction pattern to recover the phase of the incident beam and is able to achieve fast converge due to the random phase modulation. The CMI technique has been successfully applied in wave-front diagnostics in high-power laser systems[17]. All of these algorithms can realize phase reconstruction with one single measurement. However, since only one frame of the diffraction pattern is recorded, the information coded is quite limited, and they always suffer disadvantages of a small field of view, low converging speed, and low reliability. The ptychography algorithm[18] proposed by Rodenburg uses a single probe to laterally scan the target sample in overlapping positions and record the diffraction patterns; by applying the ptycholographic iterative engine (PIE) algorithm[19] to the intensity of recorded diffraction patterns, the amplitude and phase of the target sample can be reconstructed rapidly with high resolution. However, the data acquisition of the PIE experiment generally takes 10 min or more[20]; in case of an X-ray, the acquisition time will even be hours[21]. The long data acquisition time requires high stability of the experimental environment, and all of these disadvantages make the PIE algorithm unavailable for dynamic imaging. To speed up the data acquisition, our research group proposed a grating-based single-shot PIE method[22]. This method uses a two-dimensional grating to split the illuminating probe into overlapping beam clusters illuminating on the sample. In 2016, Cohen and Sidorenko[23] proposed using a pinhole array to generate an array of overlapping illuminating probes. Since these two methods replace the probe scanning process with overlapping multi-beam illumination to realize PIE with a single measurement, the data acquisition time is obviously shortened. However, the beam clusters illuminating on the sample are required to be overlapped, and, at the recording plane, they are required to be separated. These requirements make the experiment setup accordingly complex.
In this Letter, on-line beam diagnostics based on single-shot beam splitting phase retrieval is proposed. The incident beam to be measured is split into ten or more replicas by a Dammann grating; these replicas illuminate on a weakly scattering plate with known structure. Since there is no overlap between the neighboring replicas, the experiment setup can be simplified, and the diffraction patterns recorded on the charge coupled device (CCD) are easy to separate with each other totally by iteratively calculating between three planes: the grating plane, weakly scattering plane, and detector plane. The complex field of the incident beam can be reconstructed rapidly and precisely. Compared with the CMI technique, this method uses a single-shot diffraction pattern, which has more replicas, and thus, the signal to noise ratio obviously improves.
The principle of the single-shot beam splitting phase retrieval can be shown schematically with Fig.
In real experiments, the structure of the Dammann grating cannot be ideally perfect. The diffracted beams from it will not be exactly the same as each other. Then, in the iteration process, the algorithm cannot be applied only on the plane of the weakly scattering plate, the transmitting function of the Dammann grating must also be considered. A standard PIE algorithm can be applied in advance in the experiment to obtain the exact structure of the Dammann grating
The experiment setup is shown in Fig.
To get the accurate transmitting function of the Dammann grating and the weakly scattering plate, we use translation stages (Thorlabs PMZ-8) to scan them, and then we adopt a standard PIE algorithm to reconstruct their complex field. The Dammann grating is scanned in
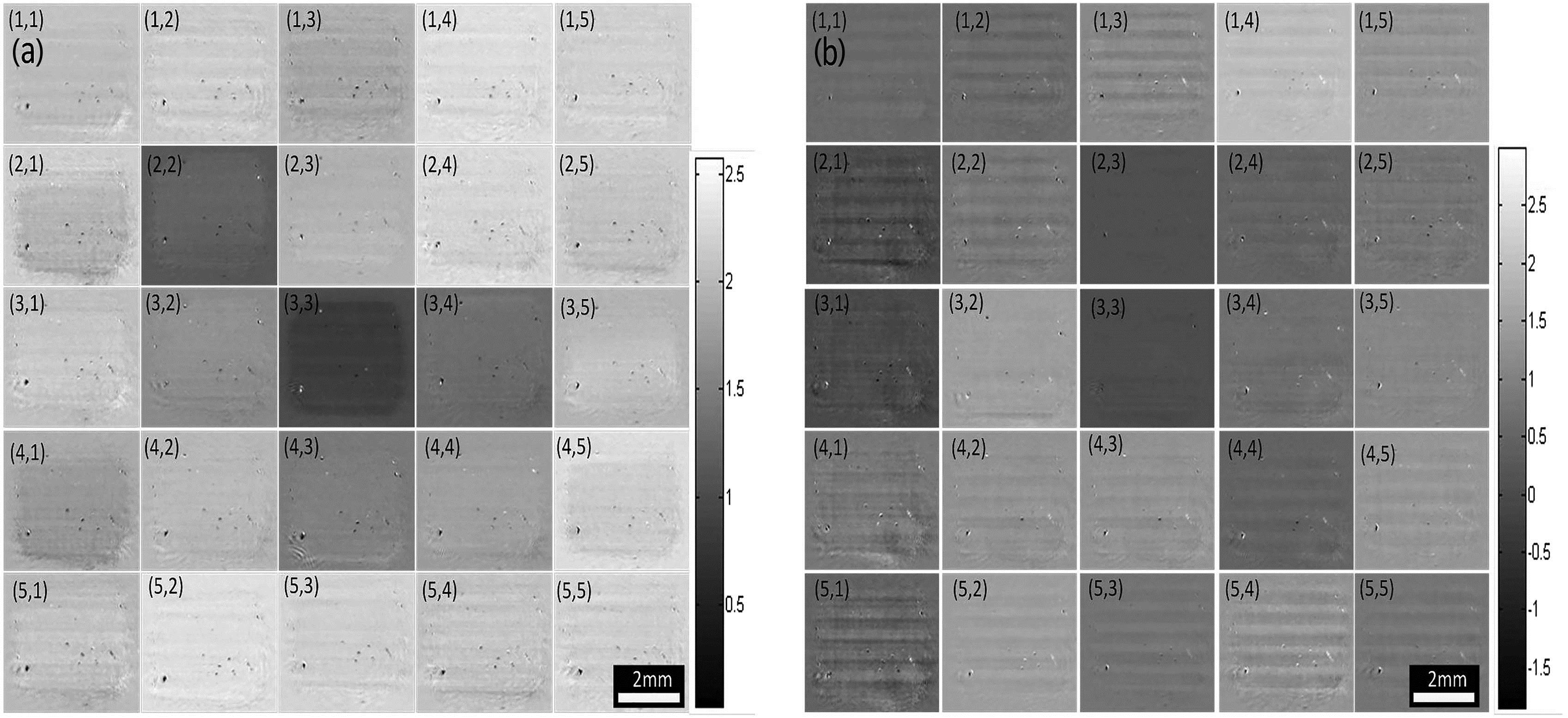
Fig. 3. orders of transmission functions of the Dammann grating: (a) the transmitting modulus and (b) the transmitting phase.
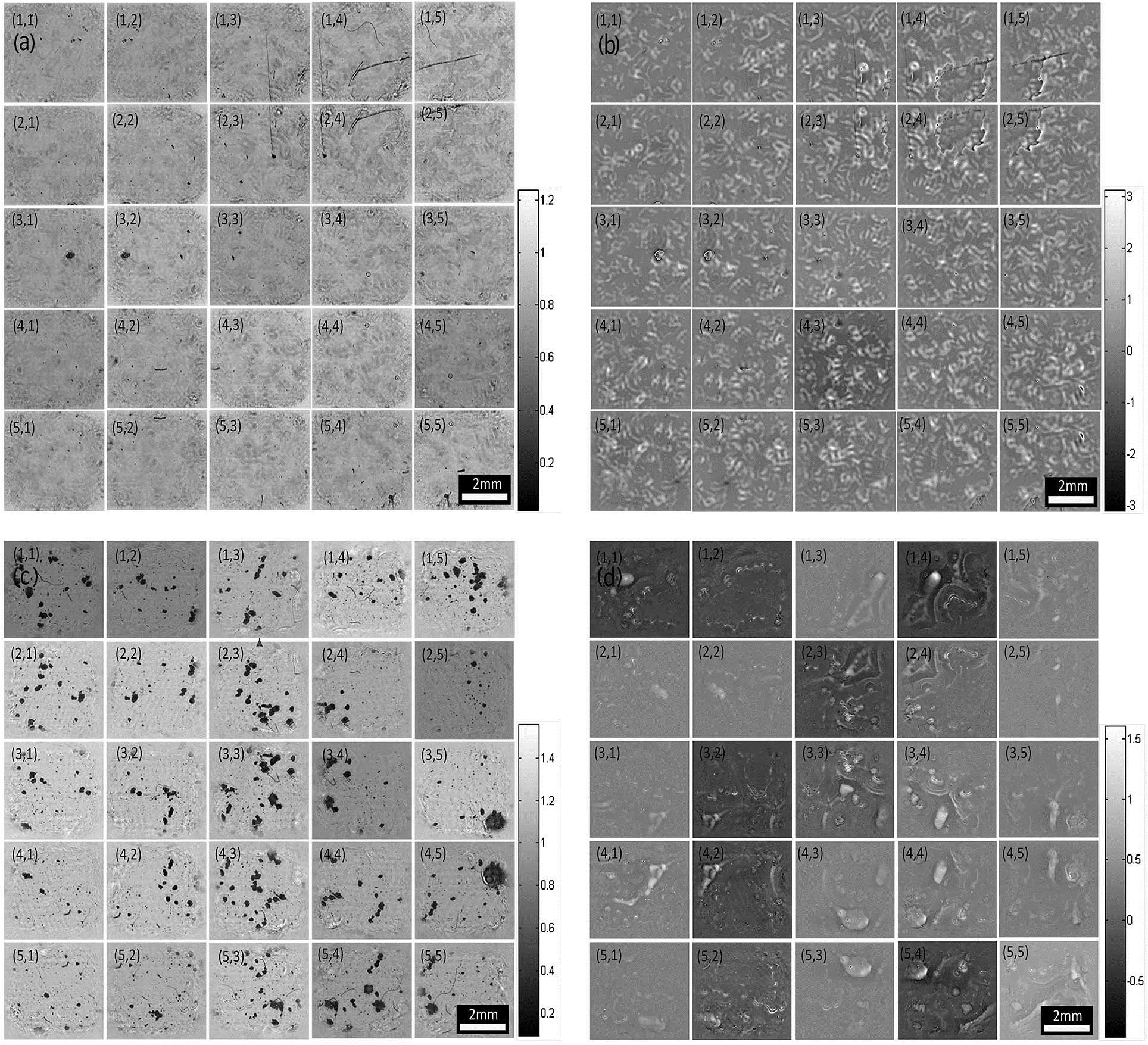
Fig. 4. (a) The transmitting modulus and (b) the transmitting phase of the orders of transmission functions of the weakly scattering plate at a wavelength of 632.8 nm; (c) the transmitting modulus and (d) the transmitting phase of the orders of transmission functions of the weakly scattering plate at a wavelength of 1053 nm.
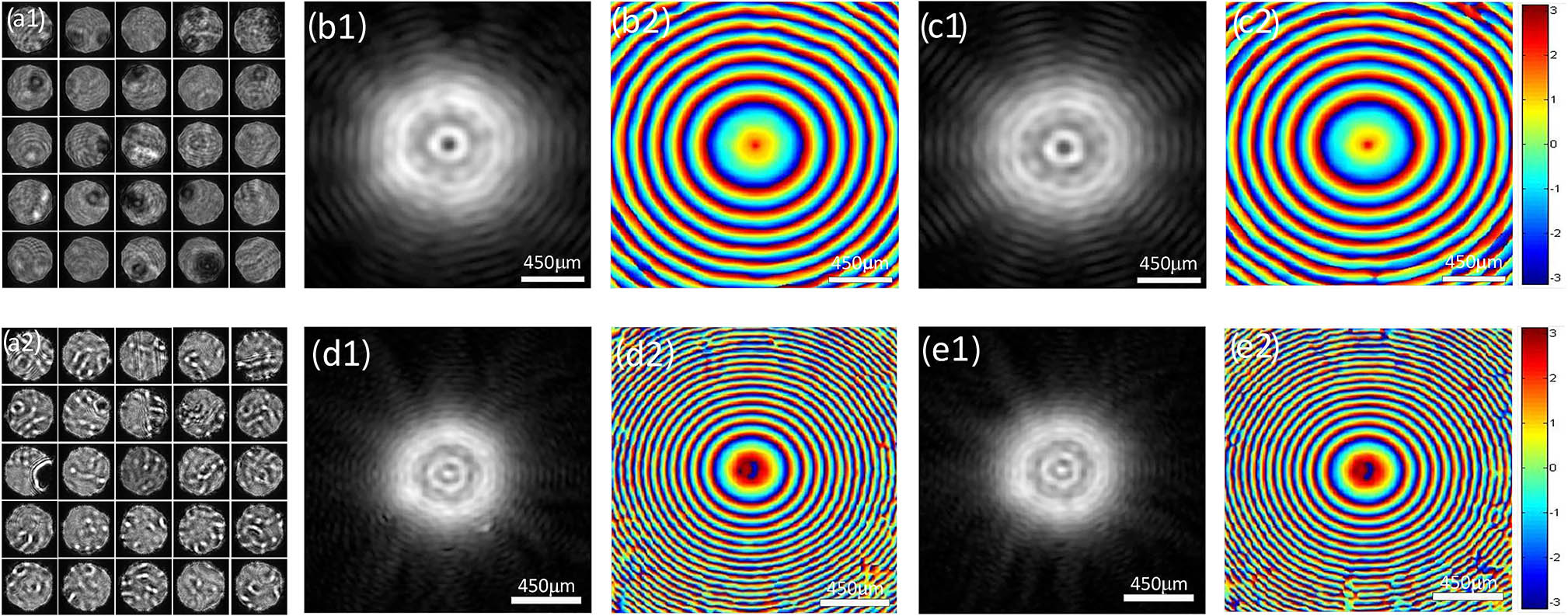
Fig. 5. (a1) The recorded diffraction pattern array at a wavelength 1053 nm; (a2) the recorded diffraction pattern array at a wavelength 632 nm; (b1) the reconstructed amplitude and (b2) phase of the light field incident on the grating at a wavelength of 1053 nm by the beam splitting phase retrieval algorithm; (c1) the reconstructed amplitude and (c2) phase of the light field incident on the grating at a wavelength of 1053 nm by the PIE algorithm; (d1) the reconstructed amplitude and (d2) phase of the light field incident on the grating at a wavelength of 632.8 nm by the beam splitting phase retrieval algorithm; (e1) the reconstructed amplitude and (e2) phase of the light field incident on the grating at a wavelength of 632.8 nm by the PIE algorithm.
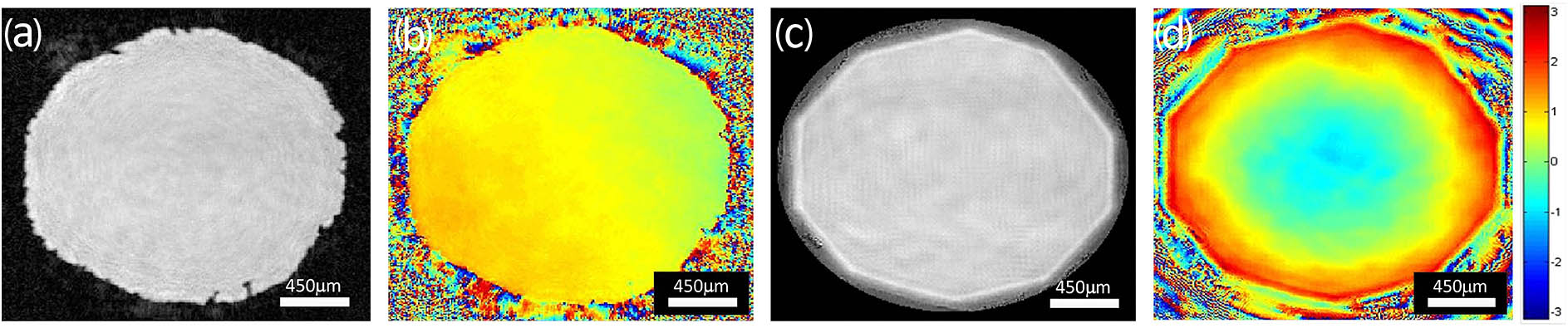
Fig. 6. (a) Reconstructed amplitude and (b) phase of the incident beam at the aperture plane at a wavelength of 632 nm by the beam splitting phase retrieval algorithm; (c) reconstructed amplitude and (d) phase of the incident beam at the aperture plane at a wavelength of 1053 nm by the beam splitting phase retrieval algorithm.
For measuring the weakly scattering plate, it is also scanned in
To verify the feasibility of this method, we use a laser beam with wavelengths 1053 and 632.8 nm as the incident beam. The diffraction patterns are shown in Figs.
To check the resolution of this method, a USAF 1951 resolution target is put at the aperture plane. Its transmitting modulus is reconstructed, and the results are shown in Fig.

Fig. 7. (a), (b) The reconstructed amplitude of USAF 1951 resolution target and its amplification of a dashed red box at a wavelength of 1053 nm in log scale; (c), (d) the reconstructed amplitude of USAF 1951 resolution target and its amplification of a dashed red box at a wavelength of 632.8 nm in log scale; (e) the curve of error changed with iteration times in log scale at wavelengths of 632.8 and 1053 nm.
The curve of error changed with iteration times in the log scale is shown in Fig.
In this Letter, a single-shot beam splitting phase retrieval method for on-line beam detection has been proposed. The incident beam to be measured is diffracted into many replicas by a Dammann grating. These replicas propagate along their own directions and pass through a weakly scattering plate with a known structure. The diffraction patterns can be recorded with a single measurement. By adopting the iterative algorithm between the grating plane, the weakly scattering plate plane, and the record plane, the complex field of the incident beam can be reconstructed; the feasibility of this method has been verified experimentally with wavelengths 632.8 and 1053 nm. Both the incident sphere wave and plane wave can be reconstructed rapidly and precisely. Since there is no overlap between the neighboring illuminating beams on the weakly scattering plate, the experiment setup is simplified and easy to be applied in on-line beam diagnostics.
[1]
[2]
[3]
[4]
[5]
[7]
[8]
[9]
[10]
[11]
[12]
[13]
[14]
[15]
[16]
[17]
[18]
[19]
[20]
[21]
[22]
Xi He, Cheng Liu, Jianqiang Zhu. On-line beam diagnostics based on single-shot beam splitting phase retrieval[J]. Chinese Optics Letters, 2018, 16(9): 091001.