掺磷非晶氧化钛负载铂用于高效催化氧化挥发性有机化合物
下载: 531次
Volatile organic compounds (VOCs), like toluene, benzene, esters and hydrocarbons, are emitted from various industrial sources which can cause serious environmental pollution and health problems[1,2]. Toluene, one kind of toxic and strong carcinogenic chemical, is frequently used in making paints, adhesives, rubbers, and leather tanning processes because of its excellent ability to dissolve organic substances[3,4]. However, toluene is difficult to degrade due to its stable structure[5]. Several techniques, such as physical and chemical adsorption, photocatalytic and catalytic oxidation methods, are widely used for the combustion of VOCs[6,7]. Among them, catalytic oxidation is regarded as a promising approach owing to its high efficiency and convenient operating conditions[8].
Researches on catalysts for toluene oxidation have been conducted, including noble metal and metal oxides catalysts[9,10]. Due to the significant reduction on activation energy during the catalytic oxidation process, noble metal based catalysts, such as Pt, Pd, Au, Rh, and Ir have shown impressive performance in toluene removal[11,12,13]. It was found that supported Pt catalysts showed the best catalytic performance compared with other noble metals[14,15]. It should be pointed out that the supports play an important role in the catalytic reaction processes[16,17,18]. Many works have focused on the metal- support interaction by studying the catalytic properties of TiO2, Al2O3, ZrO2, and ZnO supported Au nanoparticles[19], and the shape effect of Pt/CeO2 catalysts[10]. Nevertheless, most supports suffer from low specific surface area and few active sites, which are crucial for the overall catalytic activity.
Due to high specific surface area and variable valence, amorphous materials have attracted increasing interests in VOCs oxidation. And the numerous defects in amorphous structures can offer large quantities of oxygen vacancies, which are beneficial for the adsorption of oxygen and organic molecules. Lee, et al[20]. reported that carbon black supported amorphous MnOx is highly efficient for oxygen involved reaction. Wang, et al[21] found that amorphous MnOx modified Co3O4 can enhance the catalytic activity for the VOCs oxidation. It was demonstrated that the amorphous structure of bimetallic Pd-Pt/CeO2-Al2O3-TiO2 could provide more vacancies and active sites for catalytic combustion[22]. Therefore, the amorphous catalysts show a tremendous potential in practical catalytic reactions. However, it is still a challenge to develop highly active and robust catalysts based on the amorphous materials for the oxidation of VOCs.
Herein, we demonstrate an efficient Pt/ATO-P catalyst for the catalytic removal of VOCs under high gas hourly space velocity (GHSV) and high substrate concentration. It should be pointed out that incorporating phosphorus into the framework of TiO2 is a widely applied strategy for obtaining amorphous mesoporous feature[23,24]. And the P element can stabilize the TiO2 framework and significantly increase the specific surface area[24].
1 Experimental
1.1 Preparation of sample
1.1.1 Preparation of support
All reagents were of analytical grade and were used without any purification. 3 mL of tetrabutyl titanate was dissolved in 30 mL of ethanol at room temperature, which was marked as solution A. Then 0.125 mL of phosphoric acid (H3PO4) was subsequently dropwisely added into solution A with stirring to form a homogenous mixture, and kept stirring for 24 h. The obtained white solid products were separated by centrifuge, and washed by deionized water and ethanol several times, followed by freeze drying overnight. The as-prepared products were calcined at 400 ℃ in air for 4 h at a heating rate of 5 ℃min-1.
1.1.2 Preparation of catalyst
The ATO-P supported platinum (Pt/ATO-P) sample was prepared via impregnation method. A desired amount of ATO-P was transferred into aqueous solution containing appropriate amount of chloroplatinic acid (H2PtCl4). Subsequently, the samples were impregnated at room temperature for 12 h. After drying out the H2O at 80 ℃, the samples were treated at 350 ℃ for 2 h with a H2/Ar mixture (5/95, V/V).
1.2 Characterization
XRD characterization of the samples was carried out on a German Bruker D8 Advance X-ray diffractometer (XRD) using the Ni-filtered Cu Kα radiation at 40 kV and 40 mA. Nitrogen adsorption-desorption isotherms were measured at -196 ℃ on a Micromeritics ASAP 2460 analyzer. Samples were degassed at 120 ℃ for 24 h prior to the measurement. The specific surface area of the samples was calculated using the Brunauer-Emmett- Teller (BET) method with the adsorption data at the relative pressure (p/p0) range of 0.05-0.2. The total pore volumes were estimated at p/p0=0.99. The pore size distribution (PSD) curves were calculated from the adsorption branch using Barrett-Joyner-Halenda (BJH) model. The prepared materials were pressed into tablets with KBr powder and then detected by FT-IR (Perkin Elmer, USA) in the scanning range from 400 to 4000 cm-1. SEM images were obtained by Hitachi-S4800. A JEOL 2011 microscope operating at 200 kV equipped with an EDX unit (Si(Li) detector) was used for the transmission electron microscope (TEM) and high resolution transmission electron microscope (HRTEM) investigations. The samples for TEM testing were prepared by dispersing the powder in ethanol and applying a drop of highly dilute suspension on carbon-coated grids. XPS data were recorded with a Perkin Elmer PHI 5000 C system equipped with a hemispherical electron energy analyzer. The spectrometer was operated at 15 kV and 20 mA, and a magnesium anode (Mg Kα, hν=1253.6 eV) was used. The C1s line (284.6 eV) was used as the reference to calibrate the binding energies (BE). TG measurements were conducted on a Netzsch STA 449C TG-DSC thermoanalyzer. The flow rate of the carrier gas (air) was 30 mLmin-1. The temperature was raised from room temperature to 800 ℃ at a ramp rate of 10 ℃min-1. Prior to H2-TPR test, the sample (100 mg) was pretreated at 200 ℃ for 2 h and cooled to 50 ℃ in the flowing He. TPR experiment was carried out in 5vol% H2/He flowing at 30 mLmin-1, with a ramping rate of 5 ℃min-1 to a final temperature of ca. 800 ℃. The signal was monitored using a TCD detector.
1.3 Catalytic activity test
The catalytic activity of samples was evaluated in a continued-flow fixed-bed quartz reactor with 50 mg catalyst. Toluene was introduced into the reactor with bubbling toluene solution in ice bath with pure air. The concentration of toluene was about 104 mLm-3, and the flow rate was kept at 30 mLmin-1 by a mass controller, equivalent to a gas hour space velocity (GHSV) of 36000 mLh-1g-1. After steady operation for 100 min, the activity of the catalyst was tested. Toluene concentration was detected by a gas chromatograph equipped with a flame ionization detector. The toluene conversion (Xtoluene) was calculated according to the equation:
where Cin and Cout are the inlet and outlet toluene concentrations, respectively.
2 Results and discussion
2.1 Physicochemical properties of ATO-P support
Fig. 1 displays the schematic diagram of amorphous ATO-P prepared via facile co-precipitation. XRD patterns of ATO-P and TiO2 are shown in Fig. 2. All diffraction peaks of basic TiO2 sample are indexed to anatase phase (JCPDS 21-1276). Interestingly, there is no TiO2 crystal phase observed for ATO-P sample (Fig. 2), suggesting that ATO-P sample is typically amorphous and phosphorus dopant can markedly restrain the crystallization of anatase[25,26].
According to the TGA-DSC thermograms (Fig. 3), a thermal decomposition of ATO-P took place in the temperature range of 20-900 ℃. The first DSC peak at 30-80 ℃ is due to the release of physical adsorbed water. When all the water is released, Ti-OH and HPO42- groups start to condense[27]. These processes occur simultaneously in the temperature range of 100-220 ℃ (1.927% of weight loss) and 220-516 ℃ (0.7% of weight loss), resulting in an overlap of the TG data. There is no further weight loss up to 516 ℃. The DSC curve shows two exothermic peaks at 704 and 781 ℃, corresponding to a two-step exothermic transformation of ATO-P into a crystalline phase.
Fig. 4(a,b) show the SEM images of ATO-P. The ATO-P nanoparticles are homogeneously dispersed with the particle size of ~20 nm, and the sizes are similar to that of TiO2 (Fig. S1(a)). HRTEM was employed to characterize the nanostructure of samples. No porous structure is observed in the HRTEM image of TiO2 (Fig. S1(b)), while various porous structure is shown in ATO-P (Fig. 4(c)). Moreover, the pores of ATO-P are uniform, and the average diameter is around 10 nm. EDS elemental mappings indicate that the P element homogeneously distributes in ATO-P (Fig. 4(d)). It is found that H3PO4 owns unique effects for synthesizing amorphous mesoporous phosphated TiO2[28,29].
As shown in Fig. 5, the obtained ATO-P sample shows a characteristic type-IV isotherm with clear hysteresis loop locates at the p/p0 range of 0.45-1.0, showing the existence of a large amount of mesopore. Notably, the specific surface area of 278.9 m2·g-1 for ATO-P is 21 times higher than that of pristine TiO2. The pore diameters of ATO-P center around 10 nm (Fig. 5 and Table 1), which is consistent with HRTEM result (Fig. 4(c)).
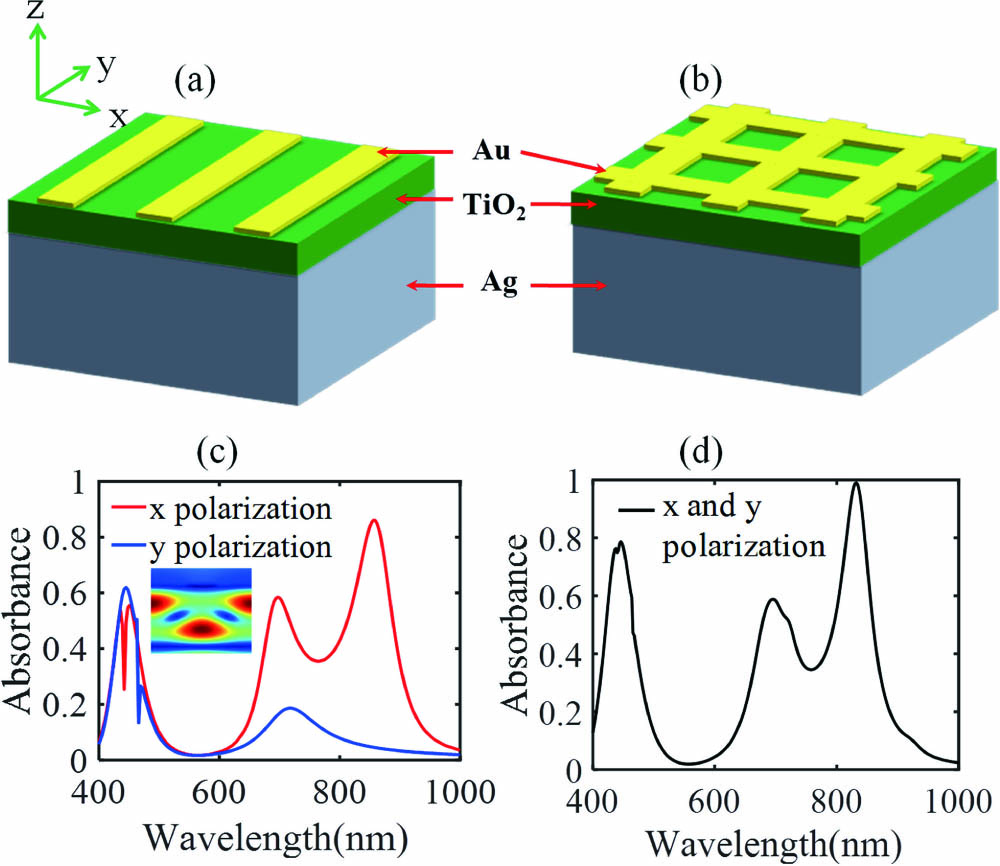
图 5.
Fig. 5. N2 adsorption-desorption isotherms (a) and pore size distributions (b) of ATO-P and TiO2
表 1.
Textural properties and elemental compositions of TiO2 and ATO-P samples
Table 1.
Textural properties and elemental compositions of TiO2 and ATO-P samples
|
The results of EDX are listed in Table 1. The actual P concentration is much less than the initial addition amount of H3PO4, suggesting that partial H3PO4 is leached during the preparation process.
FT-IR spectra of TiO2 and ATO-P samples are depicted in Fig. 6. The wide absorption bands around 3440 and 1620 cm-1 are attributed to the surface adsorbed water and/or hydroxyl groups[30,31]. The bands at 1100 cm-1 are ascribed to the stretching vibration of Ti-O-P species, which are absent in TiO2. The weak bands at 610 cm-1 are due to the vibration of Ti-O-Ti bond[22]. Compared with TiO2, a weak peak appears in series ATO-P, which may result from the incorporating effect of phosphorus dopant. There is no distinct peak over the range of 700-800 cm-1 (Fig. 6), indicating the absence of P-O-P groups in the amorphous mesoporous phosphated TiO2. Therefore, the P element is incorporated into the frameworks of ATO-P by forming Ti-O-P bonds[24].
As shown in Fig. 7(a), the full XPS spectra indicate the existence of P in ATO-P. High-resolution XPS spectra of P 2p, Ti 2p and O 1s are depicted in Fig. 7(b-d). The peak of P 2p of ATO-P is at 134.0 eV, suggesting that phosphorus in ATO-P gives a pentavalent oxidation state of P5+. No peak observed at 128.6 eV, which is the characteristic binding energy of P2p in TiP, indicating the absence of Ti-P bonds in ATO-P samples. As depicted in Fig. 7(c), the peaks of Ti2p3/2 and Ti2p1/2 in ATO-P show remarkable blue-shift owing to the incorporation effect of phosphorus element. Fig. 7(d) shows the XPS spectra of O1s signals of TiO2 and ATO-P. The single peak at 529.5 eV is corresponded to the oxygen in Ti-O bond of TiO2. However, the O1s spectrum of ATO-P contains two peaks at 531.4 and 532.9 eV, which are contributed to Ti-O-P and O-H bond, respectively[32,33].
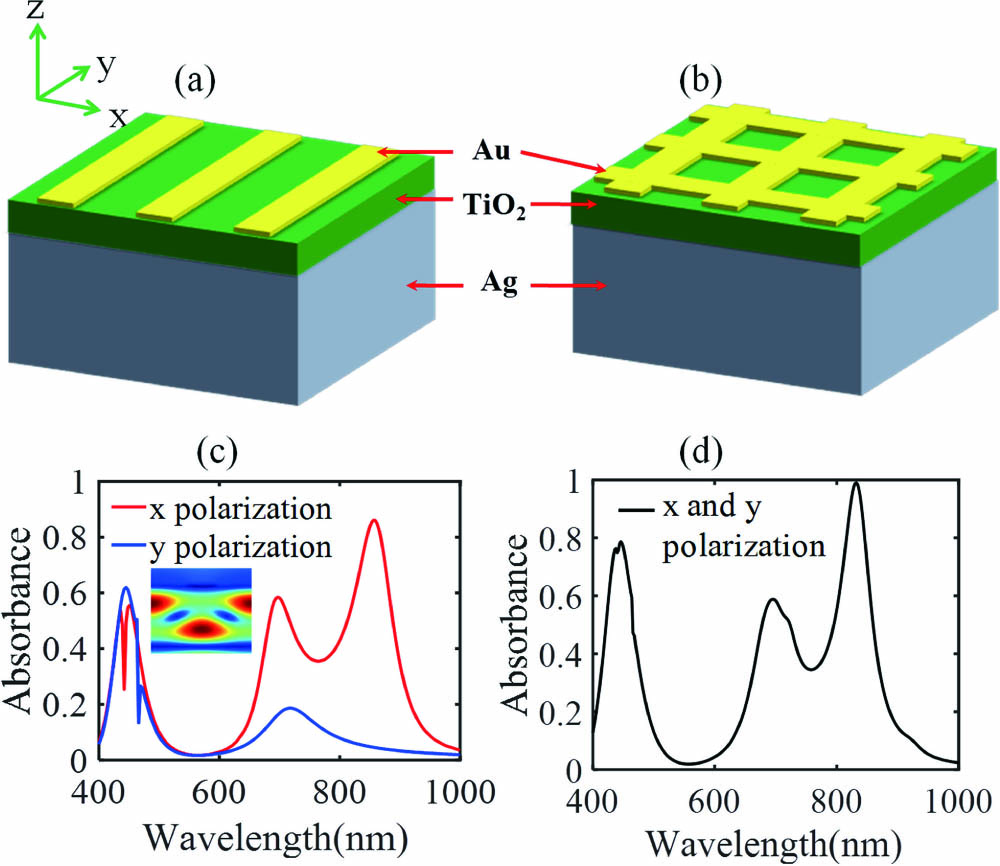
图 7.
Fig. 7. Full XPS spectra (a) of TiO2 and ATO-P; High-resolution XPS P2p (b), Ti2p (c), and O1s (d) of TiO2 and ATO-P
2.2 Physicochemical properties of Pt/ATO-P catalysts
Fig. 8(a) shows that the Pt nanoparticles are well dispersed over the ATO-P support, and the size is relatively uniform with the average parameter of (1.8±0.3) nm (insert in Fig. 8(a)). Fig. 8(b) and S2 demonstrate a d-spacing of 0.23 nm, attributed to the (111) plane of the highly crystalline Pt nanostructure. Furthermore, the actual Pt content was also confirmed by inductively coulped plasma atomic emission spectra (ICP-AES). The mass loadings of Pt in Pt/TiO2 and Pt/ATO-P catalysts are 0.90 and 0.92, respectively, which are close to the nominal composition of 1wt%.
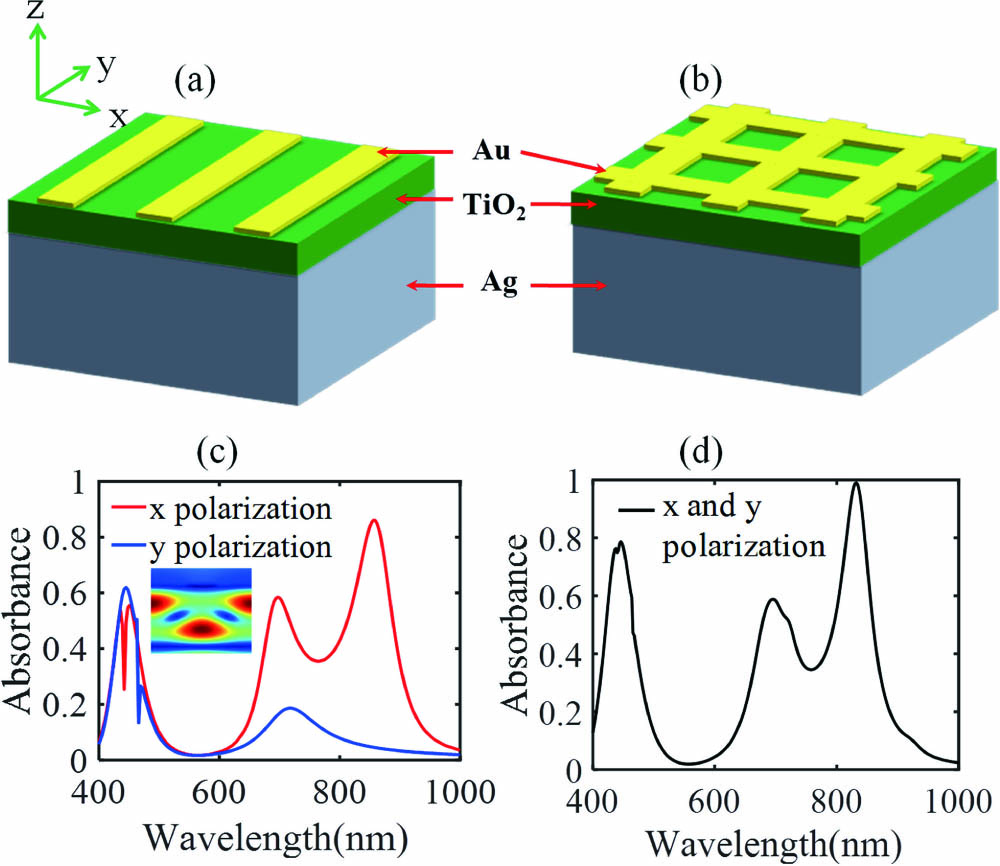
图 8.
Fig. 8. TEM (a) and HRTEM (b) images of Pt/ATO-P with insert in (a) indicating the particle size distribution of Pt nanoparticles, XRD patterns (c) and XPS Pt4f (d) of Pt/ATO-P
Fig. 8(c) shows the XRD patterns of Pt/ATO-P and Pt/TiO2 catalysts. The amorphous structure is still remained for Pt/ATO-P sample. However, no diffraction pattern of Pt nanoparticles is observed, indicating that the Pt nanoparticles are quite small and/or the Pt species are highly dispersed on the ATO-P surface. These results are well consistent with the HRTEM data above mentioned in Fig. 8(a, b).
The results of XPS analysis of Pt/ATO-P and Pt/TiO2 samples are depicted in Fig. 8(d). It is known that the positions of Pt4f7/2 binding energy at 71.1, 72.4, and 74.2 eV are attributed to Pt0, Pt2+, and Pt4+ species, respectively[34]. Similiar XPS profiles are rendered as the indication of a mixture of various valence states for Pt species over the small Pt nanoparticles. The exisence of Ptδ+ species reflects the strong metal-support interaction (Pt-ATO-P), especially the prominent electronic interaction between active Pt and underlying phosphated TiO2 support[35]. This is probably due to the changes of the metal- support interaction by doping phosphorus atoms which can make an obvious effect on Ti-O-P frameworks.
The H2-TPR profiles depicted in Fig. S3 show that there are two H2-consumption peaks at low and high temperature attributed to weak and strong interaction of Pt and supports, respectively[36]. Notably, two reduction peaks of Pt/ATO-P catalyst at 78 and 601 ℃ show stronger intensity than that of Pt/TiO2 at 72 and 433 ℃, indicating strong Pt-support interaction for Pt/ATO-P. These results are consistent with the XPS data.
2.3 Removal of VOCs by Pt/ATO-P catalysts
The catalytic efficiencies are depicted in Fig. 9. It is clearly observed that reaction temperature can enhance the performance of Pt/ATO-P catalyst. The T50 and T90 are widely used to evaluate the catalytic performance[37]. As shown in Fig. 9(a), Pt/ATO-P shows the excellent catalytic activity. T50 and T90 values for toluene combustion are 130 and 140 ℃, which are much lower than those of Pt/TiO2 with T50 and T90 of 160 and 190 ℃, respectively. Combined with the above XPS data (Fig. 8(d)), it can be concluded that the existance of phosphorus component plays an important role in electronic structure of the active Pt species underlying amorphous mesoporous ATO-P support and thus the catalytic oxidation removal of toluene over Pt/ATO-P catalyst.
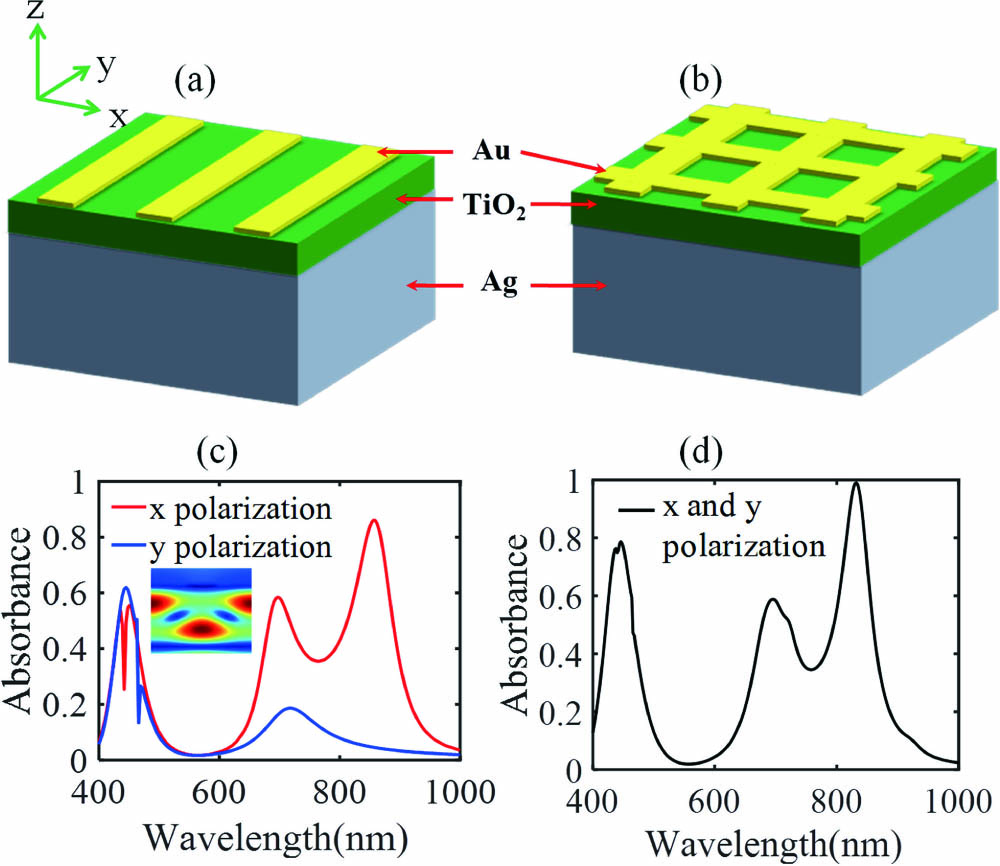
图 9.
Fig. 9. Toluene conversion (a) of 1wt% Pt/ATO-P with respect to reaction temperature, and thermal stability (b) of Pt/ATO-P at 180 ℃
It is well known that noble metal loading significantly affects the catalytic behavior for many reactions. Pt/ATO-P catalysts with different Pt loadings were examined, and the results are depicted in Fig. 10. Compared with 0.5wt% and 2wt%, the Pt loading of 1wt% shows better performance (lower T50 and T90) for toluene oxidation. The low catalytic activity of 0.5 wt% Pt/ATO-P results from low density of active platinum nanoparticles anchoring on the surface of ATO-P support. For the Pt/ATO-P catalyst with Pt loading up to 2wt%, larger size of Pt nanopartices (~5 nm) can be obtained (Fig. S4). Larger Pt particles can not only decrease the dispersion of Pt species[38], but also lead to a weaker metal-support (Pt/ATO-P) interactions, thus resulting in the poor activity.
Stability is critical for the catalysts on the practical application. 1wt% Pt/ATO-P exhibits excellent thermal stability for toluene oxidation over a 50-h period on stream at 180 ℃ without visible loss of activity, as shown in Fig. 9(b). The toluene conversion remains a high level of 95.4% at the end of reaction process and maintains near full selectivity to final products of CO2 and H2O. The excellent stability of Pt/ATO-P catalyst is attributed to the unique geometric structure of crystalline Pt nanoparticles and amorphous mesoporous phosphated TiO2 with prominent electronic interaction. For the used 1wt% Pt/ATO-P, TEM measurement and XPS analysis (Fig. S5 and Fig. S6) demonstrate no significant change on the morphology, average size of Pt nanoparticles, and the chemical oxidation state of active Pt species. These results suggest the robustness of Pt/ATO-P catalyst for toluene oxidation removal under a relatively mild thermal process.
Given the superb thermocatalytic performance for 1wt% Pt/ATO-P catalyst toward toluene oxidation, we were curious to examine whether the engineered material would also catalyze the removal of a class of VOCs, especially the complete oxidation of benzene, n-hexane, ethyl acetate, and mesitylene. As depicted in Fig. 11, the T90 values for the catalytic oxidation of benzene, ethyl acetate, n-hexane, and mesitylene are 216, 331, 271, and 200 ℃, respectively. Notably, high temperature is required for ethyl acetate conversion at 90% due to its strong structural stability[39,40]. These results show a broad scope toward catalytic combustion involving troublesome organic compounds over Pt/ATO-P and indicate that the Pt/ATO-P catalysts can provide a new insight for the oxidation of VOCs.
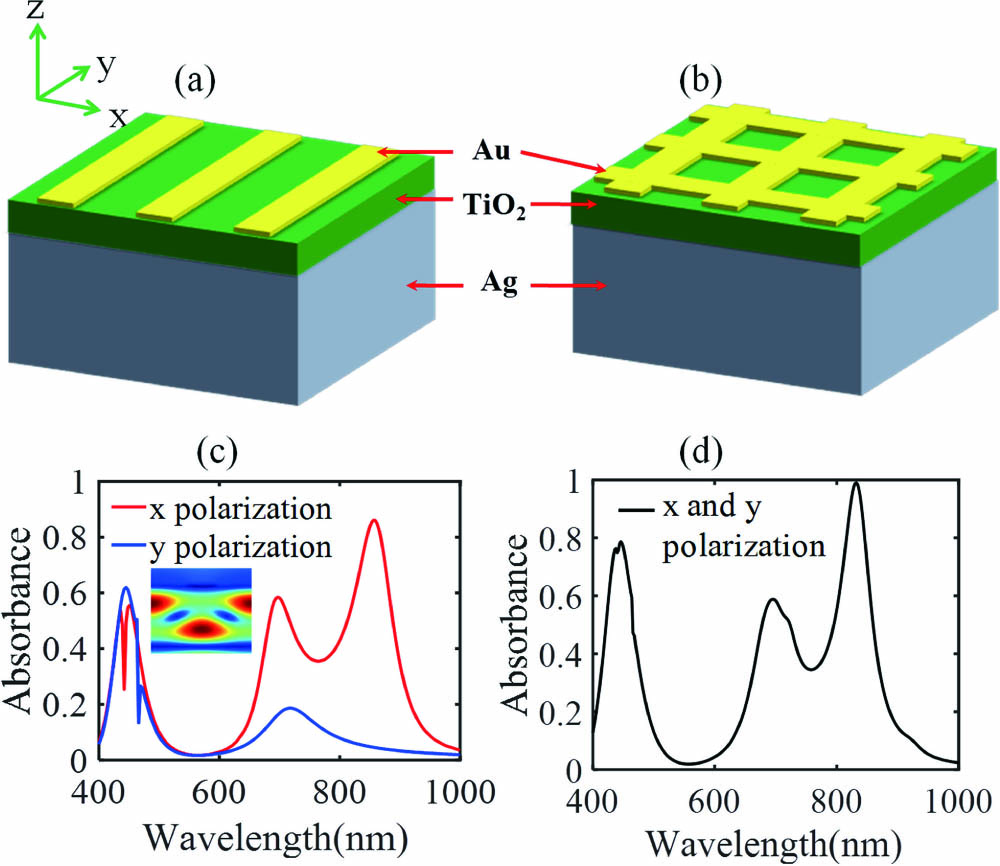
图 11.
Fig. 11. Catalytic activity of Pt/ATO-P for the conversion of benzene (a), ethyl acetate (b), n -hexane (c), and mesitylene (d) with respect to reaction temperature
3 Conclusions
In summary, we successfully fabricated the amorphous mesoporous phosphated TiO2 supported platinum catalysts for efficient removal of volatile organic compounds. The electronic modifications of supported Pt nanoparticles for the underlying amorphous ATO-P material and Pt loading for the whole catalyst were systematically investigated. The phosphorus dopant played an important role for stabilizing the inflated Ti-O-P frameworks as well as the electronic structure of Pt species. Compared with pristine TiO2, ATO-P with high specific surface area showed significant enhancement for Pt/ATO-P samples for catalytic overall oxidation of toluene under practical conditions. The performance of the engineered Pt/ATO-P for toluene combustion was superior to the reference Pt/TiO2 and comparable with the state-of-the-art catalysts. Additionally, Pt/ATO-P catalyst exhibited excellent stability for toluene oxidation removal under a relatively mild thermal process and could be potentially applied in a broad scope of VOCs. The present work is expected to make a significant contribution on the new application of amorphous mesoporous phosphated material in VOCs removal.
8 Supporting Materials:
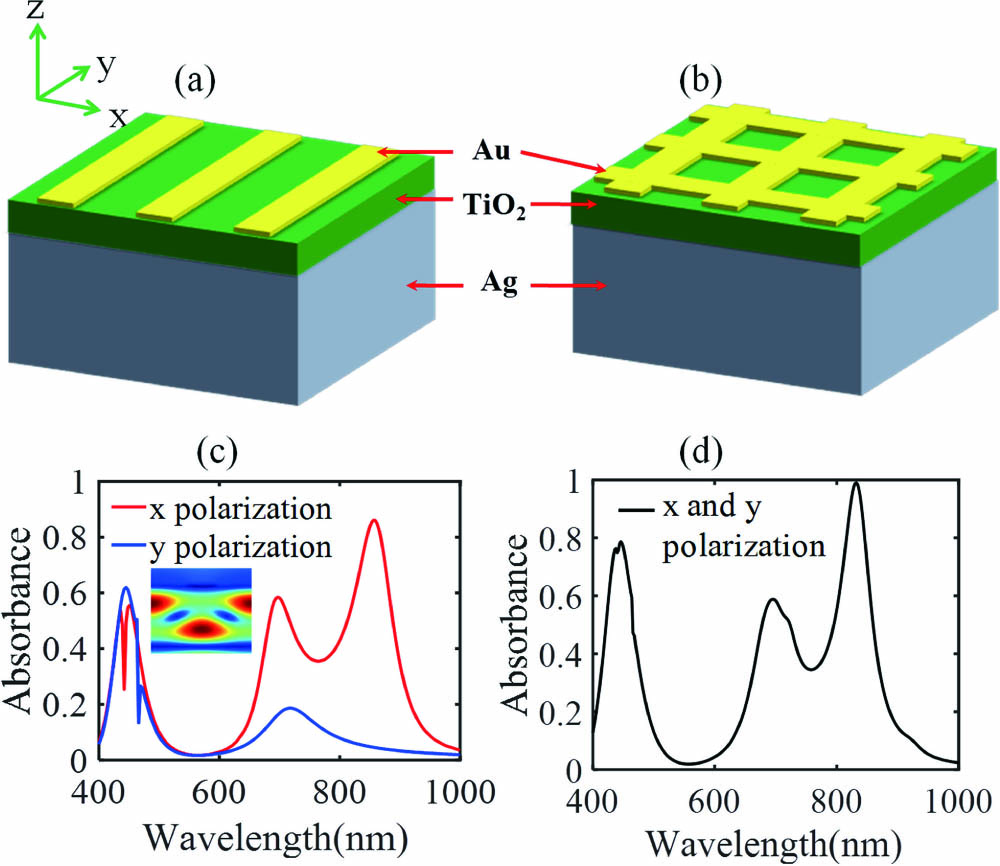
图 15.
Fig. 15. TEM images of 0.5wt% Pt/ATO-P (a) and 2wt% Pt/ATO-P (b) For the catalyst with low loading of 0.5wt%, there are few Pt nanoparticles in the ATO-P supports. By contract, the Pt particles are larger than that with loading of 1wt% when the loading up to 2wt%
Article Outline
黄谢意, 王鹏, 尹国恒, 张绍宁, 赵伟, 王东, 毕庆员, 黄富强. 掺磷非晶氧化钛负载铂用于高效催化氧化挥发性有机化合物[J]. 无机材料学报, 2020, 35(4): 482. Xieyi HUANG, Peng WANG, Guoheng YIN, Shaoning ZHANG, Wei ZHAO, Dong WANG, Qingyuan BI, Fuqiang HUANG.