Multi-band imaging and focusing of photonic crystal flat lens with scatterer-size gradient
Download: 911次
A photonic crystal (PC)[13" target="_self" style="display: inline;">–
A PC has special band structures, which can realize negative refraction in some specific bands[19,20]. Previous works have shown that a common PC (CPC) flat lens can achieve imaging of the point source[20,21], but it cannot achieve focusing of the plane wave. The PC flat lens with a scatterer-size gradient can achieve imaging of the point source[23] and focusing of the plane wave[24,25], but the band is single in previous works. The PC flat lens with a scatterer-refractive-index gradient can achieve multi-band super-resolution imaging of the point source[28], but the plane wave can only be focused in a single and low band. If the imaging of the point source and the focusing of the plane wave are simultaneously achieved in the multi-band, not only are the advantages of each band fully utilized, but also it is of great significance to design new optical focusing and imaging devices, reduce the size of devices, reduce the complexity of the system, introduce new optical functions, and so on.
In this Letter, a PC flat lens with a scatterer-size gradient is proposed, which can simultaneously achieve imaging of the point source and focusing of the plane wave in the multi-band.
The model of the two-dimensional (2D) triangular lattice PC flat lens with a scatterer-size gradient is shown in Fig.
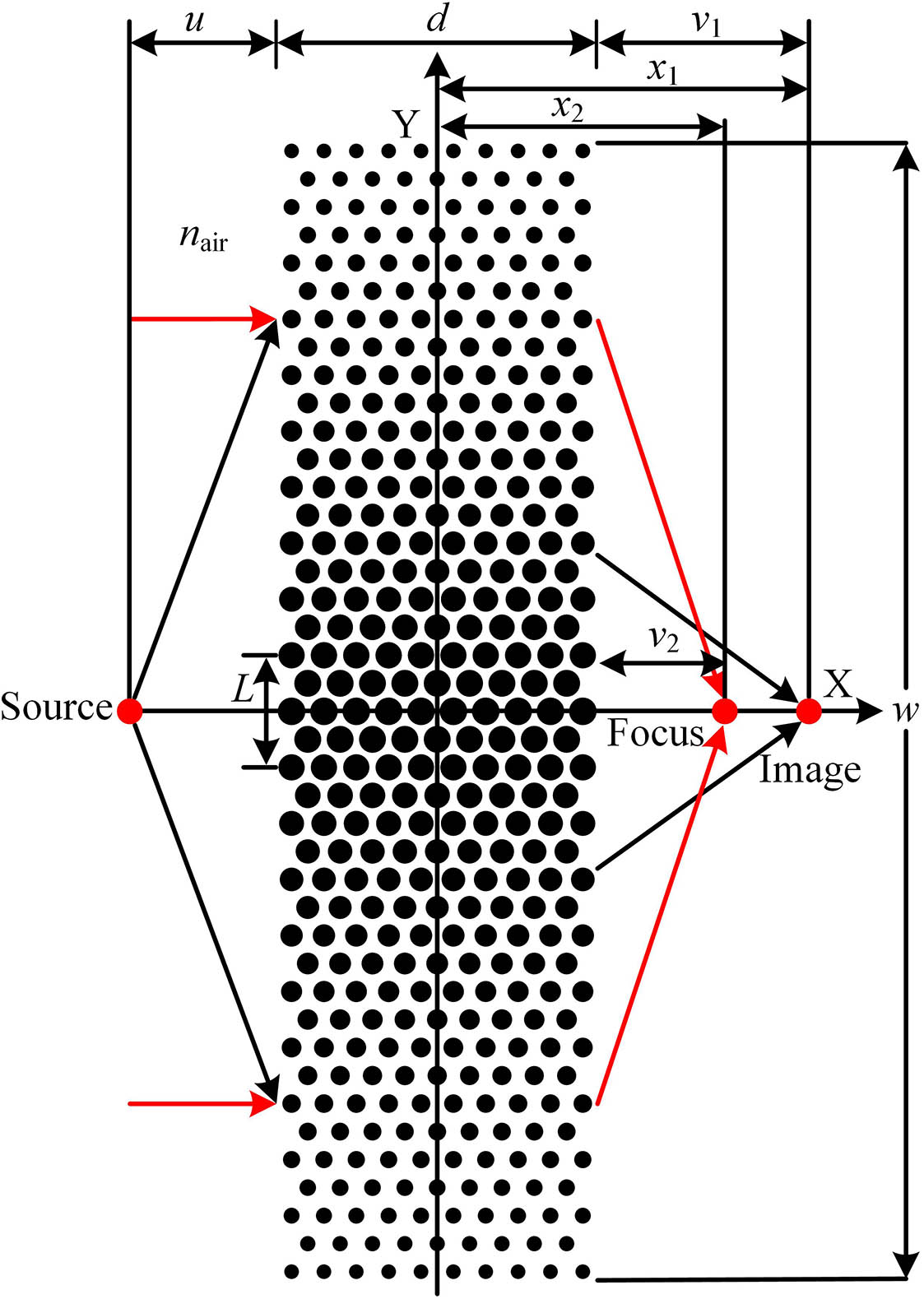
Fig. 1. Model of the PC flat lens with a scatterer-size gradient; the red arrow represents the light path of plane wave focusing, and the black arrow represents the light path of point source imaging.
When the photonic band gap (PBG) exists, the PC, whose equi-frequency line (EFL) is a circle or approximately a circle, can be equivalent to a uniform medium[29]. In addition, the PBG is generated easier by larger refractive index differences in the PC. Therefore, the scatterer (dielectric column) material is set as germanium (
The theory of negative refraction of the PC is to produce a “negative group refractive index” by using a special dispersion relation of PCs at the edge of the band gap[30]. When the lightwave is incident on the PC from air, the refractive direction is determined by the group velocity, and the vector relationship between the group velocity and wave vector
Because the frequency variation trend in the EFL of the band is correlated with its band structure, the dispersion curve can be used to judge whether the PC has a negative refraction effect. If the EFL shape of the band is circular, the PC is approximately a homogeneous isotropic medium, and the group velocity is collinear with the wave vector. The refractive direction of the beam in the PC can be analyzed using the EFL method[29], as shown in Fig.
![Direction of beam propagation in the PC is analyzed by the EFL method[29]. The left picture shows the EFL of air corresponding to the frequency of the incident beam, indicating that the beam is incident on the PC from the air. The right picture shows the EFL of the band corresponding to the frequency of the refractive beam, indicating the direction of propagation of the beam in the PC[28]. This is just a schematic diagram.](/richHtml/col/2019/17/8/080501/img_002.jpg)
Fig. 2. Direction of beam propagation in the PC is analyzed by the EFL method[29]. The left picture shows the EFL of air corresponding to the frequency of the incident beam, indicating that the beam is incident on the PC from the air. The right picture shows the EFL of the band corresponding to the frequency of the refractive beam, indicating the direction of propagation of the beam in the PC[28]. This is just a schematic diagram.
As shown in Fig.
All simulation calculations in TM polarization mode are performed with COMSOL Multiphysics software based on the finite element method (FEM). Since the scatterer’s radius of the PC flat lens with a scatterer-size gradient does not change periodically, the band structure of the PC flat lens with the scatterer-size gradient cannot be directly calculated. Therefore, the band structures of two CPCs with the scatterer’s radius
![(a) Band structures of two CPCs with the scatterer radius r =0.40 μm and r =0.39 μm (the two radii correspond to the band structures that are, respectively, distinguished by solid and dotted lines). EM propagates along the Γ−K direction of the irreducible Brillouin zone. (b) EFLs of the first band of three CPCs with different scatterer radii, the scatterer radii of the CPC are 0.4 μm (black), 0.3 μm (red), and 0.2 μm (blue), respectively; (c) ERI of the first band of CPC with different scatterer radii; (d) influence of different scatterer radii on group velocity[31]. This is just a schematic diagram.](/richHtml/col/2019/17/8/080501/img_003.jpg)
Fig. 3. (a) Band structures of two CPCs with the scatterer radius and (the two radii correspond to the band structures that are, respectively, distinguished by solid and dotted lines). EM propagates along the direction of the irreducible Brillouin zone. (b) EFLs of the first band of three CPCs with different scatterer radii, the scatterer radii of the CPC are 0.4 μm (black), 0.3 μm (red), and 0.2 μm (blue), respectively; (c) ERI of the first band of CPC with different scatterer radii; (d) influence of different scatterer radii on group velocity[31]. This is just a schematic diagram.
From Fig.
Theoretical analysis of Fig.

Fig. 4. Take as an example: (a) the imaging field of the gradient PC flat lens when the incident beam is the point source (PS) lightwave; (b) the focusing field of the gradient PC flat lens when the incident beam is a plane wave (PW); (c) field power (normalized) of imaging of PS and focusing of PW in the axial plane; (d) field power (normalized) of imaging of PS in the imaging plane and focusing of PW in the focusing plane.
As can be seen from Figs.
It can be known from previous work[32] that the CPC flat lens can realize imaging of the point source in the second band, and the imaging mechanism is that the CPC flat lens has negative ERI in the second band. As shown in Fig.
![Take λ2=4.127 μm as an example: (a) the imaging field of the CPC flat lens with r=0.40 μm when the incident beam is the PS lightwave; (b) the imaging field of the gradient PC flat lens when the incident beam is the PS lightwave; (c) the focusing field of the gradient PC flat lens when the incident beam is the PW; (d) field power (normalized) of imaging of the PS and focusing of the PW in the axial plane; (e) field power (normalized) of imaging of the PS in the imaging plane and focusing of the PW in the focusing plane; (f) the second band EFLs of two CPCs with scatterer radius r=0.40 μm and r=0.39 μm (the EFLs corresponding to the two radii and the light cone are distinguished by black, purple, and blue). The blue arrow is the incident beam, which is incident from the air to the PC. The red arrow is the direction of propagation of the beam in the PC, that is, the refractive beam. This is just a schematic diagram[34].](/richHtml/col/2019/17/8/080501/img_005.jpg)
Fig. 5. Take as an example: (a) the imaging field of the CPC flat lens with when the incident beam is the PS lightwave; (b) the imaging field of the gradient PC flat lens when the incident beam is the PS lightwave; (c) the focusing field of the gradient PC flat lens when the incident beam is the PW; (d) field power (normalized) of imaging of the PS and focusing of the PW in the axial plane; (e) field power (normalized) of imaging of the PS in the imaging plane and focusing of the PW in the focusing plane; (f) the second band EFLs of two CPCs with scatterer radius and (the EFLs corresponding to the two radii and the light cone are distinguished by black, purple, and blue). The blue arrow is the incident beam, which is incident from the air to the PC. The red arrow is the direction of propagation of the beam in the PC, that is, the refractive beam. This is just a schematic diagram[34].
As shown in Figs.
From the anisotropic left-hand negative refraction theory[33], it can be found that the fifth band has a negative refraction effect, and its focusing frequency
![Take λ5=2.582 μm as an example: (a) the imaging field of the CPC flat lens with r=0.40 μm when the incident beam is the PS lightwave; (b) the imaging field of the gradient PC flat lens when the incident beam is the PS lightwave; (c) the focusing field of the gradient PC flat lens when the incident beam is the PW; (d) field power (normalized) of imaging of the PS and focusing of the PW in the axial plane; (e) field power (normalized) of imaging of the PS in the imaging plane and focusing of the PW in the focusing plane; (f) the fifth band EFLs of two CPCs with scatterer radii r=0.40 μm and r=0.39 μm (the EFLs corresponding to the two radii and the light cone are distinguished by black, purple, and blue). The blue arrow is the incident beam, which is incident from the air to the PC. The red arrow is the direction of propagation of the beam in the PC, that is, the refractive beam. This is just a schematic diagram[34].](/richHtml/col/2019/17/8/080501/img_006.jpg)
Fig. 6. Take as an example: (a) the imaging field of the CPC flat lens with when the incident beam is the PS lightwave; (b) the imaging field of the gradient PC flat lens when the incident beam is the PS lightwave; (c) the focusing field of the gradient PC flat lens when the incident beam is the PW; (d) field power (normalized) of imaging of the PS and focusing of the PW in the axial plane; (e) field power (normalized) of imaging of the PS in the imaging plane and focusing of the PW in the focusing plane; (f) the fifth band EFLs of two CPCs with scatterer radii and (the EFLs corresponding to the two radii and the light cone are distinguished by black, purple, and blue). The blue arrow is the incident beam, which is incident from the air to the PC. The red arrow is the direction of propagation of the beam in the PC, that is, the refractive beam. This is just a schematic diagram[34].
From Figs.
As can be seen from Fig.
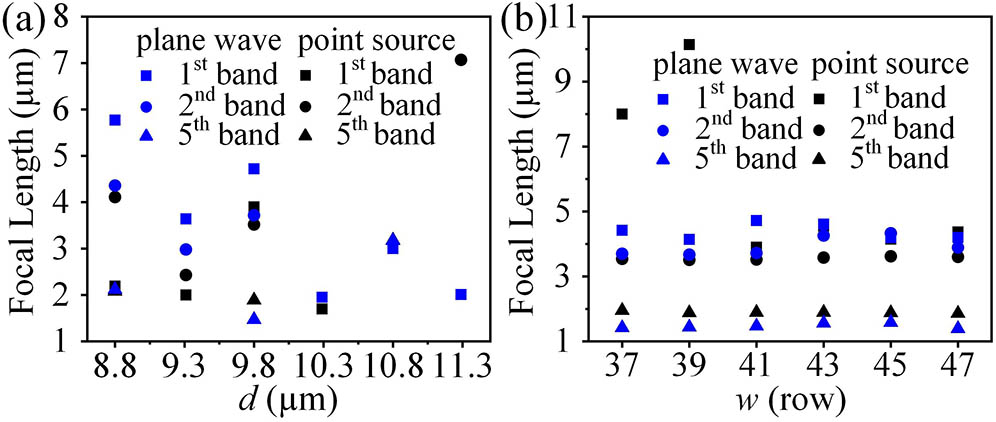
Fig. 7. Effect of the thickness and width on the focal length: (a) thickness; (b) width represented by the rows of the scatterers.
In conclusion, the PC flat lens with a scatterer-size gradient can simultaneously achieve imaging of the point source and focusing of the plane wave in the multi-band with only one structure, which is expected to reduce the preparation cost of the optical device and provide a reference for the design of new optical focusing and imaging devices in the future.
[1]
[2]
[3]
[4]
[5]
[6]
[7]
[8]
[9]
[10]
[11]
[12]
[13]
[14]
[15]
[16]
[17]
[18]
[19]
[20]
[21]
[22]
[23]
[24]
[25]
[26]
[27]
[28]
[29]
[30]
[31]
[32]
[33]
Yuan Cen, Jianlan Xie, Jianjun Liu. Multi-band imaging and focusing of photonic crystal flat lens with scatterer-size gradient[J]. Chinese Optics Letters, 2019, 17(8): 080501.