Anisotropic edge enhancement in optical scanning holography with spiral phase filtering
Download: 1047次
A common device to generate helical wavefronts or vortex beams is the spiral phase plate (SPP), which has an azimuthal structure
Edge enhancement has found utility in a variety of image processing applications where detecting the edge or shape is of interest, such as in industrial inspections[5,6], and fingerprint identification[7,8]. Generally, the enhancement effect is isotropic or symmetrical such that regardless of orientation, each edge of the input pattern is enhanced uniformly. However, in some cases, the feature information around certain orientations and edges is of greater interest and therefore requires anisotropic edge enhancement to emphasize these edges. The methods reported for selective edge enhancement include changing the integer power and offset angle of the sine function in the vortex distribution[9], fractional[10] or shifted vortex filters[11,12], a superposed vortex amplitude filter[13], and the introduction of astigmatism in the vortex filter[14].
The use of SPPs for enhancement effects in digital incoherent holography was first attempted using the Fresnel incoherent correlation holography technique[15], and then by OSH[4]. The former approach is based on self-interference and requires the capture of three phase-shifted recordings sequentially, which could introduce bias build-up when using complex objects[16]. The latter overcomes this by heterodyne scanning the object such that the holographic information is carried by the heterodyne frequency, which can be extracted using electronic filtering. With the scanning property, OSH can be applied in a variety of fields, ranging from microscopes to remote sensing[17,18].
In this Letter, we demonstrate the use of OSH to record selective edge-only information of an object holographically. We introduce three methods to do this: (1) shifting the position of the SPP to position
OSH is based on a two-pupil optical heterodyne image processor[19,20] that captures the entire holographic information of an object with a two-dimensional (2D) scan while preserving the phase information in the recording process. The two pupils in the standard OSH system are such that one pupil is a uniform function or a plane wave and the other is a delta function or point source. The interference of these two pupils is used to 2D scan an object to extract its holographic information.
The two pupil functions,
An electronic bandpass filter tuned to the heterodyne frequency
Note that in the above equations, we have performed the integration over the depth, i.e., over
Equations (
A complex hologram recorded by the system is achieved by adding Eqs. (
An SPP was first demonstrated in the OSH imaging system in Ref. [4] where the SPP was used as one of the pupil functions. Thus, for the OSH system, the pupil functions are
In Eq. (
Method 1: Anisotropic Filtering by Shifting the SPP
One approach to break the symmetry of the SPP is to shift the singularity away from the origin to a user-specified position in polar coordinates
Method 2: Anisotropic Filtering by Shifting Two Symmetrically Offset SPPs With the Same Charge
In this case, the pupil becomes
Method 3: Anisotropic Filtering by Shifting Two Symmetrically Offset SPPs With Opposite Charges
In this case, the pupil is
The holographic recordings and subsequent reconstructions are simulated in MATLAB with the implementation parameters
a. Results of Method 1:
The original object used in the simulation is shown in Fig.
![(a) |OTF| [fx and fy are spatial frequencies in unit of 1/mm] and (b) reconstructed image of single-offset SPP with 5 mm shift in the horizontal direction. (c) and (d) Output images of the single-offset SPP with different shift directions.](/richHtml/col/2016/14/1/010006/img_004.jpg)
Fig. 4. (a) [fx and fy are spatial frequencies in unit of 1/mm] and (b) reconstructed image of single-offset SPP with 5 mm shift in the horizontal direction. (c) and (d) Output images of the single-offset SPP with different shift directions.
b. Results of Method 2:
When two SPPs with the same charge are used as the pupil, the OTF seems to exhibit properties of high-pass filtering regardless of the shift distance. The simulated

Fig. 5. (a) and (b) and the reconstructed image of the two-offset SPP pupil with same charge and 5 mm shift. (c) and (d) and the reconstructed image of the two-offset SPP pupil with same charge and 2.5 mm shift.
Another difference between the single-offset SPP and two-offset SPPs can be illustrated using a white square object on a dark background. The results from using a two-offset SPPs pupil have double lines at the extracted edge, while those from using a single-offset SPP produces one line at the edge, as seen in Figs.

Fig. 6. Comparison of the edge extracted images of a square object using (a) single-offset SPP and (b) two-offset SPPs.
c. Results of Method 3:
For two offset SPPs of opposite charges, the
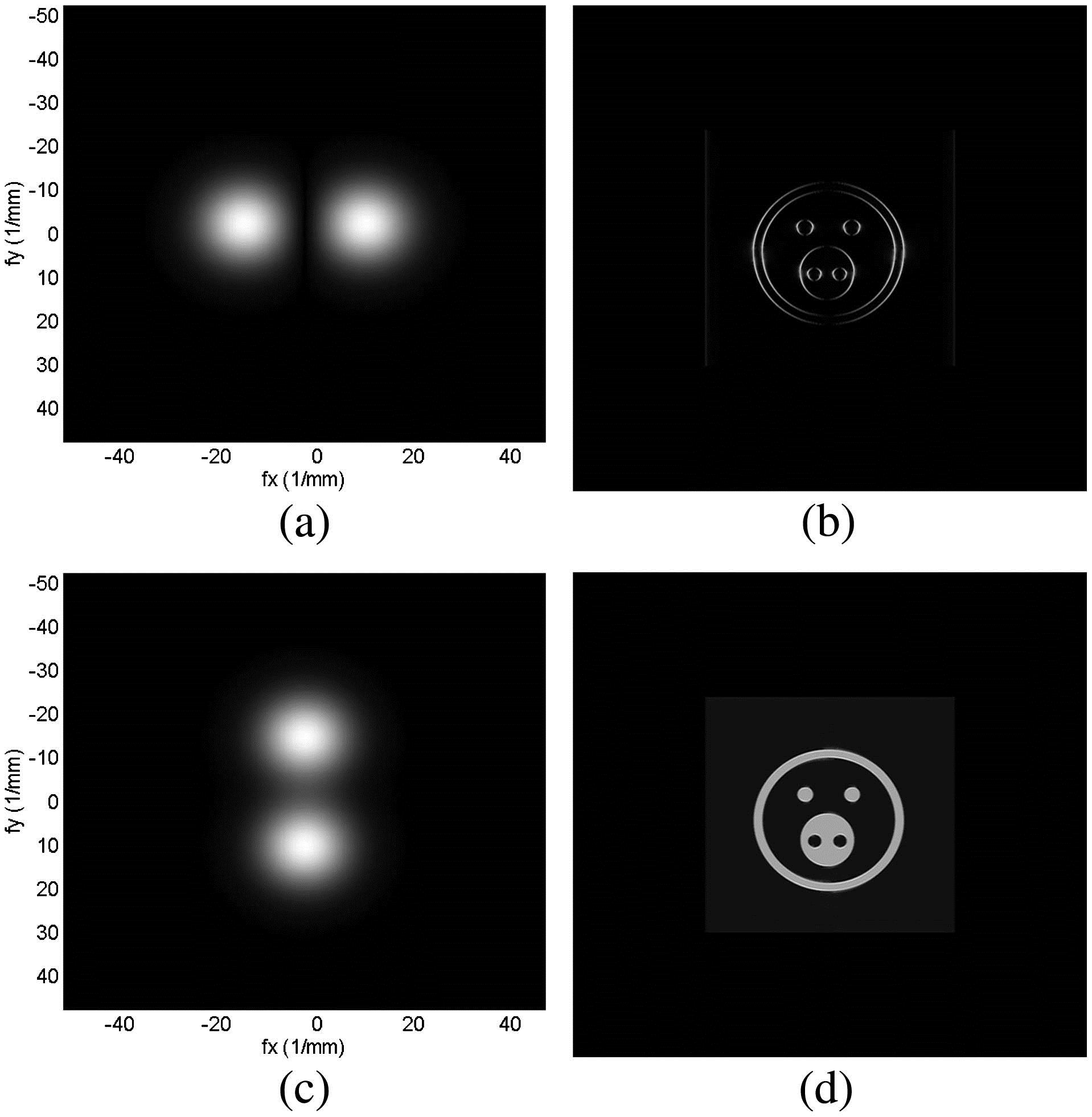
Fig. 7. (a) and (b) reconstructed image of the two-offset SPPs as a pupil with opposite charges and 2.5 mm shift, (c) and (d) reconstructed image of the two-offset SPPs as a pupil with opposite charges in the vertical direction.
If the two SPPs are shifted in the vertical direction,

Fig. 8. Improved and the reconstructed images of the two-offset SPPs as a pupil with opposite charges in the form of Eq. (11 ), and is (a) and (b) and (c) and (d) .
In conclusion, we propose three methods to realize anisotropic filtering in an OSH system using SPPs as one of the pupil functions. The first method shifts the vortex filter in the pupil plane, the second method employs two symmetrically offset vortices of the same charge, and the third method uses two symmetrically offset vortices of opposite charges. Anisotropic edge enhancement effects are demonstrated for all three methods in the computerimulations. The single-shifted SPP produces selective edge enhancement results for large shift distances but cannot remove the low-frequency information when shift distances are small. Anisotropic edge enhancement results for small shift distances are obtained by adding a same charge or opposite charge SPP in the radially opposite direction, as illustrated in the second and third methods, respectively. Further, method 3 demonstrated anisotropic edge enhancement more distinctively than method 2, as more information in the direction perpendicular to the SPP orientation is filtered out. Additional areas of investigation include comparing results from the computer simulations to those from experimental investigations and implementing other anisotropic filtering approaches.
[1]
[2]
[3]
[4]
[5]
[6]
[7]
[8]
[9]
[10]
[11]
[12]
[13]
[14]
[15]
[16]
[17]
[18]
[19]
[20]
Kelly K. Dobson, Wei Jia, Ting-Chung Poon. Anisotropic edge enhancement in optical scanning holography with spiral phase filtering[J]. Chinese Optics Letters, 2016, 14(1): 010006.