Broadband polarization beam splitter based on a negative refractive lithium niobate photonic crystal slab
Download: 959次
The polarization beam splitter (PBS) can split two different beams of mutually orthogonal polarizations along different directions. The PBS has played an important role in optical fiber communication and integrated optics as an important device in polarization optical correlation systems. The traditional PBS[1,2] uses birefringence in a natural crystal or polarization selectivity in a multiplayer structure to realize polarization splitting based on the Brewster angle. But it has the following drawbacks: (1) the natural crystal requires a high thickness, (2) the multilayer structure has a complex processing technology with a millimeter level size that cannot be applied to the micro-machining technology, (3) it is sensitive to the change of angle, and (4) the transmission light has a low extinction ratio. Therefore, the traditional PBS cannot meet the size needs of modern integrated optics systems.
In recent years, several kinds of PBSs[35" target="_self" style="display: inline;">–
Recently, more research has been focused on lithium niobate (LN)-based PhCs[1618" target="_self" style="display: inline;">–
In this Letter, we propose an LN-based PBS by using a refractive negative PhC slab that features two different PBGs and the propagation conditions of TE polarization and TM polarization at the wavelength of 1.55 μm. This pillar-type wedge PhC slab has a lattice constant of 0.816 μm and a rod radius of 244.8 nm. This PBS can separate the incident light with
Here, we demonstrate a PBS based on negative refraction. The principle of negative refraction is shown in Fig.
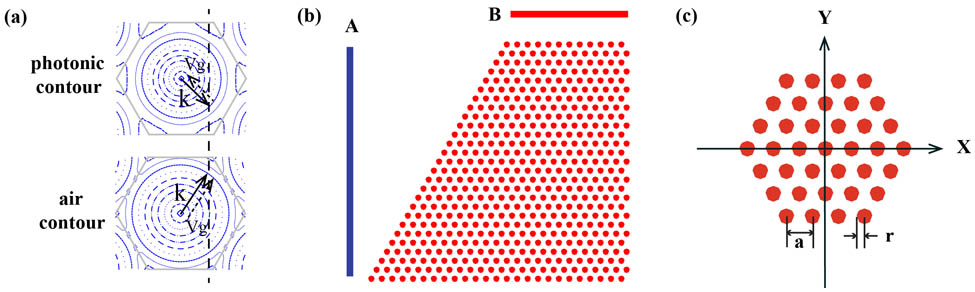
Fig. 1. Configuration of the negative refractive PhC. (a) The principle of negative refraction. The direction of group velocity is along the increasing direction of the contour line. The wave vector is on the same side of the normal at the interface of the air and the PhC. (b) The two-dimensional PhC based on LN. Ports A and B are the two receivers to measure the transmission of the incident light. (c) The enlarged structure of the PhC with a hexagonal array. is the lattice constant. is the radius of the dielectric rod.
The negative refractive PhC we constructed is shown in Figs.
Figure

Fig. 2. Realization of the PBS. The incident light is separated after passing through the PhC. (a) TE polarization refracted in the negative direction. (b) TM polarization refracted in the positive direction. A and B are the two receivers to measure the light transmission.
Here, we give the theoretical analysis of the PBGs of the PhC by using the plane wave expansion method, which needs at least two PBGs to achieve negative refraction. We assume that

Fig. 3. Bandgap as a function of the wave vector for (a) TE polarization and (b) TM polarization.
Then, we study the relationship between the transmission and free-space wavelength based on the normalizing condition. Under this condition, the lattice constant and waveguide width are

Fig. 4. Curves of transmission versus free-space wavelength based on the normalizing condition with the incident angle of 20° of the two polarizations. (a) TE polarization. (b) TM polarization. The optional free-space wavelength range based on the normalizing condition is 1.85–1.92 μm. The normalized frequency range is 0.52–0.54.
We choose the wavelength of 1.55 μm as the light source. According to the normalized frequency formula

Fig. 5. Relationship between the transmission and incident angle. The gray segment represents the region where the PhC acts as a PBS. (a) TE polarization for LN-based PBS. (b) TE polarization for Si-based PBS. (c) TM polarization for LN-based PBS. (d) TM polarization for Si-based PBS. For the LN-based PBS, the angle bandwidth is 20°–28° with a light transmittance of over 80%. For the Si-based PBS, the transmission of TM polarization is less than 80%, due to the large reflection that occurs at the surface.
Then, we choose 22° for the LN-based PBS and 14° for the Si-based PBS as the incident angles. The relationship between the transmission and wavelength is shown in Fig.
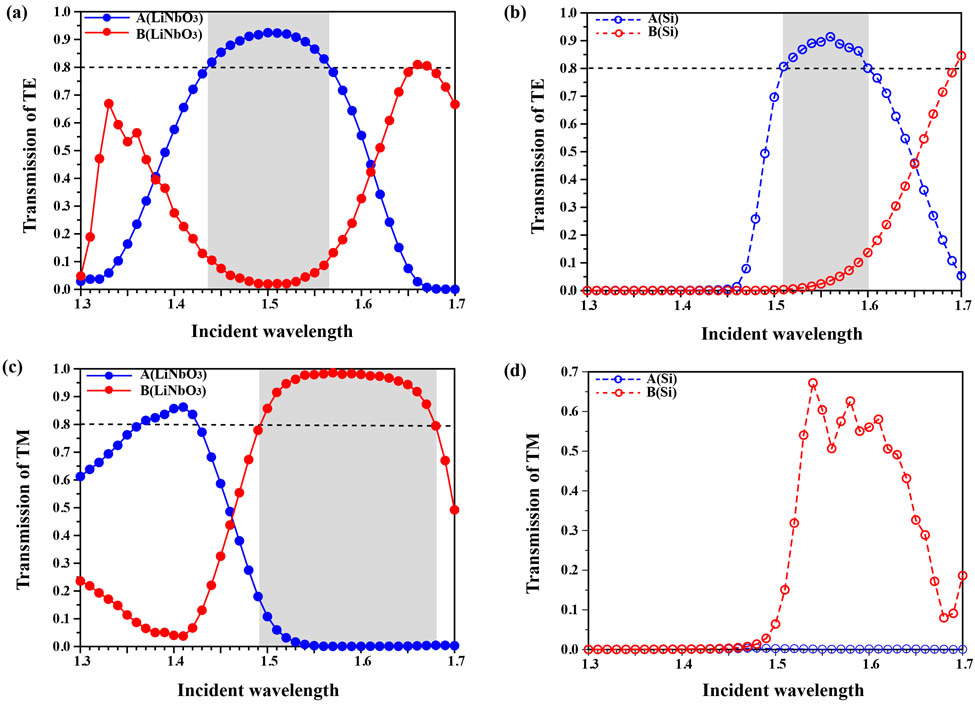
Fig. 6. Relationship between the transmission and wavelength. The gray segment represents the region that the PhC acts as a PBS. (a) TE polarization for LN-based PBS. (b) TE polarization for Si-based PBS. (c) TM polarization for LN-based PBS. (d) TM polarization for Si-based PBS. The wavelength bandwidth range is 1.50–1.57 μm, and the span of the wavelength is 70 nm with a transmission of over 80% for the LN-based PBS. The transmission of TM polarization based on Si cannot be over 70% under the condition of the two polarizations separating completely, because it has much more energy because of the surface reflection.
We compare the difference between the LN-based and Si-based PBSs. The transmission of TM polarization based on Si cannot be over 70% under the condition of the two polarizations separating completely. The LN-based PBS has a larger wavelength bandwidth than the Si-based PBS.
In conclusion, we demonstrate an LN-based PBS with a wedge-shaped negative refractive PhC. It has a good performance, including an efficient polarization splitting function with a high transmission of over 80%, an angle bandwidth of 8°, and a wavelength bandwidth of 70 nm. With a Si-based PhC, besides the large reflection that occurs at the surface and induces energy loss, no good PBS feature can be obtained with high efficiency. Combined with the other nonlinearities of LN, the LN-based PBS may play an important role in on-chip integrated quantum computation and quantum communication.
[1]
[2]
[3]
[4]
[5]
[6]
[7]
[8]
[9]
[10]
[11]
[12]
[13]
[14]
[15]
[16]
[17]
[18]
[19]
[20]
[21]
[22]
[23]
[24]
Siqi Duan, Yuping Chen, Guangzhen Li, Chuanyi Zhu, Xianfeng Chen. Broadband polarization beam splitter based on a negative refractive lithium niobate photonic crystal slab[J]. Chinese Optics Letters, 2016, 14(4): 042301.