Experimental research on impulse coupling effect of a multi-pulse laser on an aluminum target
Download: 548次
Pollution of the space environment is getting more and more serious due to space debris, especially those of centimeters in size. Active clearance of centimeter space debris is the inevitable choice and the only way out[1
In this Letter, we designed a non-contact, multiple-degrees-of-freedom experimental system that can measure recoil impulses to overcome those drawbacks. The experimental devices in this Letter mainly are comprised of a vacuum test chamber, a superconducting magnetic levitation system, a displacement measurement system, and a high-energy laser. As the core parts of our experimental system, the chamber and the maglev system can create the low temperature, low pressure, and weightless space environment for the aluminum target, and the structural design is shown in Fig.
The vacuum test chamber is a vertical container with an inner diameter of 1000 mm and a straight section length of 1250 mm. Three circular observation windows are equipped outside the container. The middle observation window is a fully transparent lens, and the high-energy laser passes through it. This window can be opened for easy pick-and-place of the target. The observation windows on both sides are high-pressure lenses for the cameras that are capturing and recording images. As shown in Fig.
The magnetic levitation system is mainly used for simulating the microgravity or weightless environments in space. Magnetic levitation is achieved by utilizing the pinning effect of a superconductor in a magnetic field. A racetrack-shaped NbTi electromagnetic coil and a high-temperature superconductor YBCO (yttrium barium copper oxide compound) together constitute the magnetic levitation system. The electromagnetic coil (the magnet) can generate a magnetic field distribution with enough field strength and uniformity in the target region. The pinning effect happens when the temperature of the superconductor falls below the critical temperature (about 90 K), which means that it undergoes a transition from a normal-conducting state to a superconducting state (referred to as field cooling) in the magnetic field. The YBCO superconductor will be locked in the uniform magnetic field as its levitation force counteracts the gravity. The lower the temperature drops, the stronger the suspension stiffness and stability are. Therefore, only the horizontal motion can be performed and there is no damping in the direction that can be considered as “free motion” horizontally. Below the magnet, a “free” movement district with a suspension height of 5–10 cm and a length of 50 cm can be formed.
The displacement measurement system uses the camera measurement method. It is a non-contact measurement method that is intuitive, automated, and highly accurate, and is very suitable for monitoring the target’s parameters of displacement and attitude. Two digital cameras capture the suspending target from two directions and performs real-time or post-processing analysis on the collected sequence image to calculate the position parameters of the suspending target relative to the world coordinate system. The target to be measured is abstracted as a mass point, and the light is used to make the target display more prominent features than the background. For example, the background is set to a dark color and the mass point is set to a bright color. As long as the target has a little obvious feature point, the high-speed cameras can easily identify and track the target and measure its position information. In this experiment, the center of the spherical object can reflect a distinct bright spot, which we use as a feature point. In this way, particle features can be extracted from the image by simple segmentation. The principle of two camera measurements is that the position of the cameras is known and the cameras can obtain the angle information of target. According to the cameras’ position information and the target’s angle information, the absolute position of the target in space can be determined.
The high-energy laser system used in our experiment can generate the required pulse with a wavelength of 1064 nm, a pulse width of 10 ns, and a beam diameter of 1.2 cm. It also has an adjustable energy in the range of 0–5 J for a single pulse and an adjustable pulse repetition in the 1–5 Hz range.
The experimental target irradiated by the high-energy laser consists of two parts, one is a superconducting block of YBCO material, and the other, as a load shell, is a commonly used spacecraft aluminum material. The superconducting block is embedded in the center of the aluminum shell. In order to more easily embed the superconductor, we cut off the upper and lower parts of the sphere with two parallel planes symmetric about the center of the sphere, as shown in Fig.
The impulse coupling effect experiment of multi-pulse laser irradiation of the aluminum target includes three processes, in order: creating a low-temperature and low-pressure vacuum environment by air pumping and system cooling; making the target levitate at a set height to create a weightless environment; performing the experiment of laser irradiation on the target and recording the displacement data. The laser parameters are shown in Table
Table 1. Laser Parameters
|
Figure
Then we obtain the curve of the speed variation with the number of pulses in which the interval time between two pulses is 1 s, as shown in Fig.
Reference [23] presents a theoretical calculation method for the recoil impulse of a 3D-surface target irradiated by a single-pulse laser,
If the recoil impulse of the multi-pulse laser irradiation on the target is a simple linear superposition of the results after every single-pulse action, the displacement over time curve is shown as the red curve in Fig.
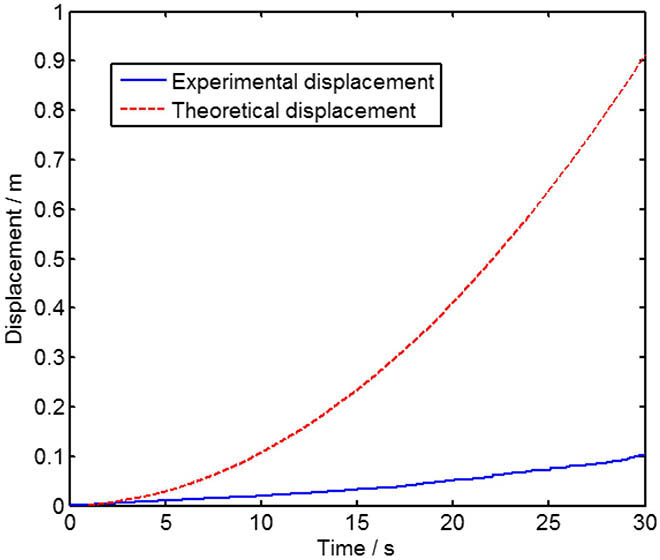
Fig. 7. Comparison between the direct accumulated results of every single-pulse action and the experimental result.
In order to better study the impulse coupling effect of multi-pulse laser irradiation on space debris, analogous to the method of Eq. (
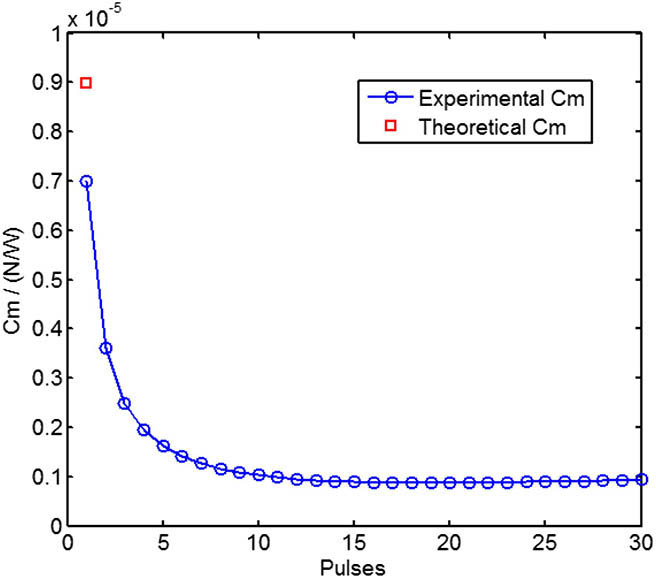
Fig. 8. Trend of the multi-pulse impulse coupling coefficient changing with the number of pulses.
The theoretically calculated values of the velocity increment and the impulse coupling coefficient of the first single-pulse laser irradiation on the target in Fig.
Figure
It is worth noting that in our experiment, during the first 30 pulses of the laser irradiation on the aluminum target, it is ensured that the aluminum target has a good suspension stability in the vertical direction, but after that, with the following pulses, the aluminum target gradually loses suspension stability, and ultimately is unable to continue suspension. It is an increase in temperature that causes the target to lose the condition that satisfies the pinning effect. There are two main reasons for the temperature rise. First, we used a contact temperature sensor (thermistor) to measure the temperature under continuous pulse action. The laser is set to the parameters corresponding to the experimental laser, and after 30 pulses action, the temperature of the target rose from 294.75 K to 297.82 K. Second, external heat radiation is also an important cause of the target temperature rise. In the experimental verification, even if the laser does not work, the suspending target will eventually fall. The stabilization time is maintained between 20 min and 30 min, and the laser action will accelerate its temperature rise effect.
In summary, this Letter shows the principle of the pinning effect to design a set of experimental systems that can realize a recoil impulse measurement after multi-pulse laser irradiation on space debris with a multi-degree of freedom and without contact damping. Using aluminum as the experimental target, the experiment of the impulse coupling effect of the multi-pulse laser irradiation on space debris was performed and relevant theoretical analysis was carried out. The experimental results demonstrate that the multi-pulse impulse coupling effect is not the direct accumulation of the results of every single-pulse laser coupling with the target, and the multi-pulse impulse coupling coefficient decreases with the increase of the number of pulses, but will eventually stabilize at a certain value. More importantly, it can guide us to calculate the recoil impulse of multi-pulse coupling. The experimental conditions are out of the atmospheric influence, so it is adapted to the space-based laser for debris removal and could provide some reference for the research of the removal process.
[4] 4CastronuovoM. M., Acta Astronaut.69, 848 (2011).AASTCF0094-576510.1016/j.actaastro.2011.04.017
[5] 5CovelloF., Adv. Space Res.50, 918 (2012).ASRSDW0273-117710.1016/j.asr.2012.05.026
[6] 6MonroeD. K., Proc. SPIE2121, 276 (1994).
[7] 7ChoM., J. Spacecr. Rockets31, 920 (1994).10.2514/3.26537
[8] 8PhippsC. R.ReillyJ. P., Proc. SPIE3092, 728 (1997).
[9] 9SchallW. O., Proc. SPIE3343, 564 (1998).
[10] 10BohnW. L., Proc. SPIE3612, 79 (1999).
[11] 11PhippsC.LanderM., in AIP Conference Proceedings, American Institute of Physics (2011), p. 339.
[12] 12SchallW. O., Proc. SPIE3574, 426 (1998).
[13] 13CampbellJ. W.TaylorC. R., Proc. SPIE, 3343, 583 (1998).
[14] 14LiuJ.GeL.FengL.JiangH.SuH.ZhouT.WangJ.GaoQ.LiJ., Chin. Opt. Lett.14, 051404 (2016).CJOEE31671-7694
[15] 15SchallW. O., J. Spacecr. Rockets39, 81 (2002).10.2514/2.3785
[16] 16EarlyJ. T.BibeauC.PhippsC. R., AIP Conf. Proc.702, 190 (2004).
[17] 17EsmillerB.JacquelardC., AIP Conf. Proc.1402, 347 (2011).
[18] 18PhippsC. R.BakerK. L.LibbyS. B.LiedahlD. A.OlivierS. S.PleasanceL. D.RubenchikA.TrebesJ. E.GeorgeE. V.MarcoviciB.ReillyJ. P.ValleyM. T., AIP Conf. Proc.1464, 468 (2012).
[19] 19LiuZ.ZhangY.DingJ.SunS.HuB., Chin. Opt. Lett.15, 121401 (2017).CJOEE31671-7694
[20] 20BohnW. L., AIP Conf. Proc.1464, 442 (2012).
[23] 23WangC.ZhangY.WangK., Proc. SPIE10152, 101520E (2016).PSISDG0277-786X10.1117/12.2245326
Qianqian Shi, Yan Zhang, Kunpeng Wang, Chenglin Wang, Peng Zhao. Experimental research on impulse coupling effect of a multi-pulse laser on an aluminum target[J]. Chinese Optics Letters, 2018, 16(12): 121401.