21 MHz~100 kHz重复频率亚皮秒NALM锁模光纤激光器
下载: 1465次特邀研究论文
Objective The repetition rate of a mode-locked fiber laser pulse is one of the most important defining parameters. On-demand repetition rates vary, serving a wide range of applications. For example, lasers with high pulse repetition rates (between tens of MHz and a few GHz) are not only used to generate optical frequency combs or coherent stacked pulses, but also offer high-precision wavelength calibration for astronomical spectrographs. In contrast, lasers with low pulse repetition rates (below 1 MHz) are highly valued in industrial laser materials processing to keep the characteristics of cooling machining. The mode-locked lasers with high repetition rates can easily be generated by shortening the laser cavity length. However, when it comes to the lasers with low repetition rates, the things get more complex and costly because these lasers require an additional acousto-optic modulator (AOM) or electro-optic modulator (EOM) to pick pulses from a laser source with high repetition rates. Compared with the traditional SESAM (semiconductor saturable absorption mirror) mode-locked fiber lasers, the mode-locked fiber lasers with nonlinear amplifying loop mirrors (NALM) as artificial saturable absorbers demonstrate the advantages of fast relaxing time, high damage threshold, life expectancy, all-fiber structure, and ultralow repetition rates over a relative broadband. These pulses can be generated by elongating the cavity length. After compression, these pulses have a sub-picosecond duration. In this study, we report a sub-picosecond NALM mode-locked fiber laser at different repetition rates varying from 21 MHz to 100 kHz by adjusting the length of the passive fiber at the proper position in the oscillator. All pulses with different repetition rates can be compressed to a sub-picosecond level.
Methods We constructed the NALM mode-locked fiber laser based on an all-fiber structure and an all-polarization-maintaining design. To realize different repetition rates in the mode-locked laser pulse output, we gradually elongated the oscillator cavity length via inserting two additional passive fiber segments into two different locations in the cavity (Fig. 1). In case of pulse repletion rates below 1 MHz, the length and position of the two passive fiber segments were carefully designed to avoid accumulating excessive nonlinear effects, such as stimulated Raman scattering, which undermined the stability of mode-locked pulses and decreased the output pulse energy. In particular, the first passive fiber segment was spliced after the gain fiber, whereas the second one was spliced after the output coupler. Finally, we demonstrated a 20 μm/130 μm Yb-doped double cladding fiber amplifier with an ultralow repetition rate NALM mode-locked fiber laser as seed instead of any pulse-picking device.
Results and Discussions First, we obtained a self-starting mode-locked pulse train with a repetition rate of 21.16 MHz at the main and NALM pump powers of 90 mW and 126 mW. The output pulse was centered at 1030 nm with a 3-dB bandwidth at 9.1 nm, and the pulse duration was compressed from 5.3 ps to 352 fs by a pair of 1379 lp/mm gratings (Fig. 2). Second, we obtained mode-locked pulse trains with repetition rates of 5.92 MHz, 1.28 MHz, 457 kHz, 280 kHz, 181 kHz, and 100 kHz by adjusting the cavity length through the addition of two passive fiber segments. The cavity lengths were 33.8, 156, 438, 714, 1106, and 2000 m, respectively (Table 1 and Fig. 3). While the pulse repetition rate decreased from 21 MHz to 100 kHz, the pulse energy and duration increased by two orders of magnitude, namely, from 1 nJ to 104 nJ and 5.3 ps to 300 ps, respectively. The broadest 3-dB spectrum at 30 nm was demonstrated at a repetition rate of 5.92 MHz, which corresponded to the shortest compressed pulse duration of 177 fs. Importantly, all the pulses were compressed to a sub-picosecond level. Especially for the ultralow repetition rate pulses, it was a good choice for ultralow repetition rate laser system to be front-end seeded without any pulse-picking devices. Finally, a 388-kHz, 62.7-ps, 20.8-nJ NALM mode-locked seed was amplified to 3 μJ after a single 20/130-μm Yb-doped double cladding fiber amplifier stage (Fig. 5), which was further compressed to 537 fs.
Conclusions We report on a mode-locked ytterbium-doped fiber laser with a nonlinear amplifying loop mirror in an all-polarization-maintaining designed cavity. The repetition rates of the laser vary between 21 MHz and 100 kHz by adjusting the length of passive fiber at the proper position of the oscillator. The 5.3-ps mode-locked pulse with a 3-dB bandwidth at 9.1 nm is first obtained at a repetition rate of 21.16 MHz, which is then compressed to 352 fs. The broadest 3-dB bandwidth at 30 nm and the shortest compressed pulse duration of 177 fs are demonstrated at a repetition rate of 5.92 MHz. Limited by the length of available passive fiber, we obtain a pulse with a maximum energy up to 104 nJ and duration of 300 ps directly from the oscillator at the lowest repetition rate of 100 kHz. The pulse is subsequently compressed to 1.053 ps. All the output mode-locked pulses at different repetition rates with a broad spectral bandwidth are characterized and compared with the traditional low repetition rate mode-locked fiber lasers. They are compressible to sub-picosecond, which is different from the dissipative soliton resonance and noise-like pulses. A 388-kHz, 62.7-ps, 20.8-nJ NALM mode-locked laser seed is amplified to 3 μJ after a single-fiber amplifier stage, which could be further compressed to 537 fs, and this whole laser system is very compact without any pulse-picking components or multistage fiber amplifiers.
1 引言
近年来,锁模光纤激光器因为其光束质量好、峰值功率高、结构紧凑稳定等特点,一直备受关注,被广泛应用于光学频率梳[1-3]、激光手术[4]、激光微纳制造[5]等方面。不同的应用场景所需要的脉冲重复频率是不一样的。例如,光频梳[1-3]、相干脉冲堆积[6]所需的重复频率为几十MHz或者几百MHz甚至GHz;而在工业加工领域,为了保证冷加工的特性,脉冲的重复频率通常低于1 MHz,需要使用额外的脉冲选择装置如声光调制器(AOM)或者电光调制器(EOM)对锁模脉冲进行降频[7-9],这增加了系统的复杂性和经济成本。通过减小腔长可实现高重复频率锁模脉冲[3],但是通过增加腔长获得的低重复频率锁模脉冲的重复频率范围却是有限的,其重复频率一般在1 MHz以上[10-12]。随着振荡器腔长从百米增加至千米级,对应的重复频率则降低至1 MHz以下,输出脉冲更多以类噪声(NLP,noise-like pulse)或者耗散孤子共振(DSR,dissipative soliton resonance)的形式出现[13-17],不能被压缩至亚皮秒,关于耗散孤子脉冲的研究鲜有报道[18],这种低重复频率的脉冲激光器作为工业加工飞秒光纤激光器系统前端种子源的优势不够明显。
在锁模方式上,半导体可饱和吸收体反射镜(SESAM)等实体可饱和吸收体虽然发展了很多年,但是仍然受工作寿命的限制,表现出逐渐退化甚至失锁。在某些特殊精密应用上,如光频梳,激光器的噪声是一个不得不考虑的参数,SESAM本身较长的恢复时间会明显助长激光器的噪声水平,是各类技术噪声的主要诱导因素[19-20]。近年来,Mamyshev振荡器的发展也很迅速,其输出能量可达到几十nJ甚至μJ量级[21-23],但是Mamyshev振荡器需要利用外部注入信号来启动锁模。非线性放大环形镜(NALM)这类虚拟可饱和吸收体具有较短的恢复时间、较低的输出噪声及较高的损伤阈值和输出能量,不受锁模退化和工作寿命的限制,依靠光纤环路中的非线性相移差便可以实现锁模,不需要额外的实体可饱和吸收器件 [24-30],且全光纤结构和全保偏设计保证了结构的紧凑性和工作的稳定性。更重要的是,通过增加腔长,在低重复频率下也能实现可压缩的宽带锁模[25,27],支持压缩后亚皮秒脉冲的输出。
在锁模脉冲形成机制上,传统孤子的形成依赖于腔内的负色散和非线性,受孤子面积定理的限制,脉冲能量一般小于0.1 nJ;色散管理孤子在腔内经历正负色散的交替变化,可实现脉冲周期性的展宽和压缩,将脉冲能量提升至nJ量级。在全正色散腔中,正色散和非线性无法相互抵消以形成孤子,脉冲始终处于展宽过程中,需要在腔内引入滤波耗散机制,实现脉冲周期性的时域窄化和光谱滤波,从而维持脉冲的自洽,即产生耗散孤子,输出脉冲能量可以达到几十nJ。在巨啁啾振荡器(GCO, giant-chirp oscillator)中,通过增加腔长,赋予耗散孤子脉冲更大的线性啁啾,同时保证必要的光谱滤波机制,能够将耗散孤子的脉冲能量进一步提升,输出脉冲具有高度的啁啾、较大的脉宽以及陡峭的光谱边沿。
本文报道了基于全保偏NALM的锁模光纤激光器,通过调整腔内无源光纤的长度和位置,将锁模脉冲的重复频率从最高21 MHz逐步降至最低100 kHz,获得了单脉冲能量为nJ至几百nJ量级的宽带锁模脉冲输出,并且所有重复频率下的脉冲都可以被压缩至亚皮秒量级。当重复频率为21.16 MHz时,获得了光谱带宽为9.1 nm、压缩后脉宽为352 fs的锁模脉冲;当重复频率为5.92 MHz时,获得了最宽光谱带宽为30 nm、最窄脉宽为177 fs的压缩脉宽;在2 km的腔长下,当最低重复频率为100 kHz时,输出了脉宽为300 ps、单脉冲能量为104 nJ的脉冲,并且脉宽可以被压缩至1.053 ps。当重复频率为388 kHz时,利用单脉冲能量为20.8 nJ的NALM锁模,通过单个光纤放大级,实现了3 μJ的能量输出,压缩后脉宽为537 fs,对应的峰值功率为4.47 MW。从振荡器能够直接获得重复频率为几十MHz到几百kHz、能量在nJ量级以上的可压缩锁模脉冲,满足光频梳等高重复频率场景和工业加工等低重复频率场景中前端种子源的需要。
2 实验装置
实验装置结构如
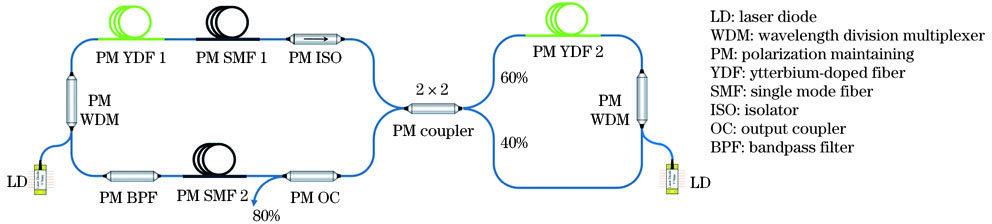
图 1. 全保偏NALM锁模掺镱光纤激光器装置的结构示意图
Fig. 1. Structural diagram of all-PM Yb-doped NALM mode-locked fiber laser setup
分别使用分辨率为0.02 nm的光谱分析仪和带宽为25 GHz的实时高速示波器对脉冲的光谱和时域进行测量,并使用自相关仪对压缩前后的脉宽进行测量记录。
3 分析与讨论
首先,按照
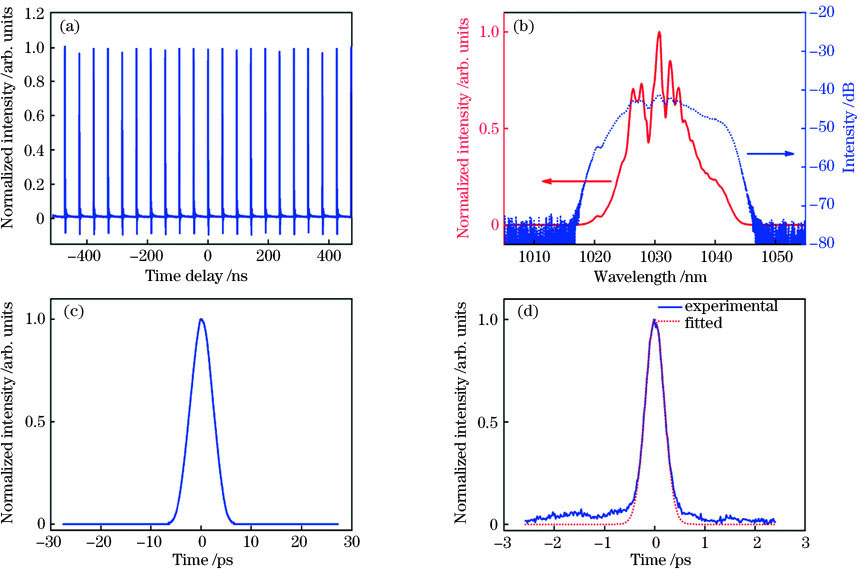
图 2. 21.16 MHz锁模激光器的输出参数。(a)脉冲序列;(b) 输出光谱;(c)输出脉冲的自相关轨迹;(d)压缩后的脉冲自相关轨迹
Fig. 2. Output parameters of mode-locked laser at repetition rate of 21.16 MHz. (a) Pulse sequence; (b) output spectrum; (c) autocorrelation trace of output pulse; (d) autocorrelation trace of compressed pulse
随后在腔内熔接额外的保偏无源单模光纤以增加腔长,振荡器直接输出了具有超低重复频率的锁模脉冲。当腔长分别增加到33.8,156,438,714,1106,2000 m时,均获得了稳定的锁模脉冲序列,对应的重复频率分别为5.92 MHz、1.28 MHz、457 kHz、280 kHz、181 kHz以及100 kHz,各重复频率下的谐振腔参数和脉冲输出参数如
表 1. 不同重复频率下的谐振腔参数和脉冲输出参数
Table 1. Resonant cavity parameters and output pulse parameters at different repetition rates
|
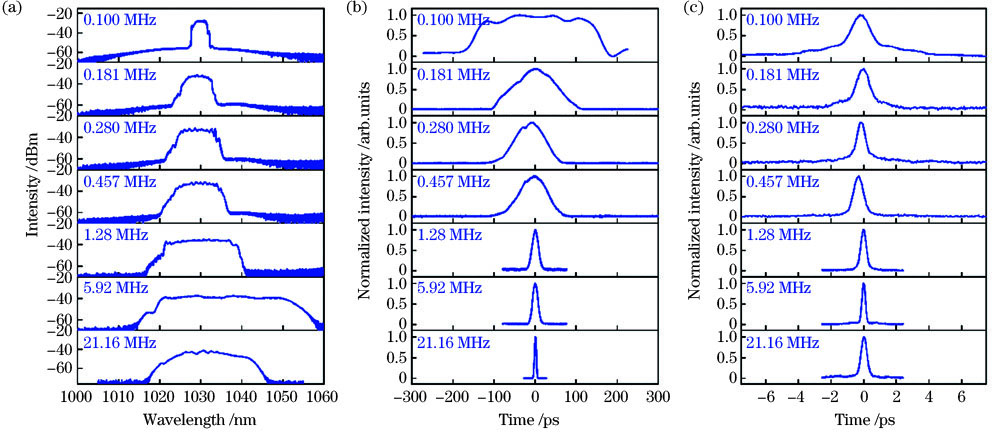
图 3. 不同重复频率下的锁模激光器输出参数。(a)输出光谱;(b)输出脉宽; (c)压缩后的脉宽自相关轨迹
Fig. 3. Output parameters of mode-locked laser at different repetition rates. (a) Output spectra; (b) output pulse durations; (c) autocorrelation traces of compressed pulses
当振荡器脉冲的重复频率从21.16 MHz降低至100 kHz时,单脉冲能量提升了近两个数量级,振荡器直接输出了104 nJ的单脉冲能量。随着腔长的不断增加,锁模脉宽逐渐增加,当重复频率为180 kHz时,其脉宽接近自相关仪的最大量程,自相关轨迹有明显的失真迹象,因而只能采用25 GHz带宽的实时高速示波器和15 GHz带宽的高速光电探头直接测量更宽的脉宽。当重复频率为100 kHz时,测量得到的脉宽为300 ps,脉冲形状为平顶陡沿形,但是该锁模脉冲并不是类噪声脉冲或者耗散孤子共振。因为其自相关轨迹并不存在一个包含突出尖峰的大基座,脉宽也没有随泵浦功率的增加而明显增加,而这两点分别被认为是NLP和DSR存在的明显证据。
对于其他重复频率下(21 MHz~181 kHz)的锁模脉冲,其色散量较小,脉冲宽度均能被一对尺寸为14 mm ×10 mm、光栅刻线密度为1379 lp/mm的透射式光栅压缩至177~663 fs量级,对于重复频率为100 kHz的脉冲的压缩,需要使用一对更大尺寸的光栅刻线密度为1740 lp/mm的反射式光栅,300 ps的脉宽被该光栅压缩后变为1.053 ps。当重复频率较高时,压缩后脉冲的自相关轨迹比较平滑,整体呈现高斯型。然而,随着重复频率的不断降低,虽然非线性的过量积累已得到极大抑制,但是越来越长的无源光纤引入的三阶色散和压缩光栅对引入的三阶色散却无法避免。当重复频率为280,181,100 kHz时,可以在压缩后的锁模脉冲自相关轨迹两翼位置观察到由三阶色散引起的逐渐隆起的基底。
在腔内脉冲循环过程中,不同位置处增加同样长度的无源光纤,所积累的非线性效应是不一样的。相较输出耦合器后面的位置,在腔内脉冲能量较高的位置如主环路增益纤后面,所积累的非线性更强。然而,当重复频率在MHz量级以下时,腔内的非线性积累是一个很重要的因素。一方面,为了实现宽光谱的NALM锁模输出,需要积累一定的非线性相移以获得宽光谱特性;但是另一方面,较长的腔长很容易积累过高的非线性效应,引起受激拉曼散射(SRS)等,不利于锁模脉冲的稳定性和高能量脉冲的输出。因此,低重复频率NALM锁模需要调控腔内的非线性效应,即调控无源光纤的长度和位置。在低重复频率(低于1 MHz)下,我们尝试改变两段保偏无源单模光纤的位置,将第二段无源光纤SMF 2熔接在第一段无源光纤SMF 1后面,尽管腔长没有变化,但是却只能得到调Q脉冲和类噪声输出,无论如何调节泵浦功率都很难实现稳定的锁模。相较于两段式的光纤分布,当将同样总长度的无源光纤完全放置在主环路增益光纤后面时,获得了极大的非线性相移积累,这不利于锁模脉冲的建立。此外,得益于腔内80%的能量经耦合器输出到腔外,腔内非线性积累大大减弱,因而在腔长不断增加、对应的重复频率不断降低的同时,尽可能地将额外的无源光纤增加到第二段无源光纤上,即输出耦合器之后,可避免非线性积累过高。
如

图 4. 输出光谱带宽、脉冲能量、脉宽以及压缩脉宽随重复频率的变化
Fig. 4. Output spectral bandwidth, pulse energy, pulse duration and compressed pulse duration versus repetition rate
相比于传统的SESAM锁模方式,NALM锁模能够在很大的重复频率范围内实现稳定的锁模,特别是在超低重复频率条件下,利用单模光纤振荡器,即可实现能量为几十nJ甚至几百nJ、脉宽可压缩至亚皮秒的脉冲输出,作为放大级前端时,NALM锁模的优势不言而喻。一方面振荡器直接输出了具有超低重复频率的脉冲序列,避免使用脉冲选择器件;另一方面,超低重复频率振荡器提高了单脉冲能量,省去了后端的多级光纤预放大,并将脉宽提高至几十ps甚至几百ps量级,不需要额外的展宽器,便可以直接进入主放大级以放大能量。
为了验证放大的可行性,采用
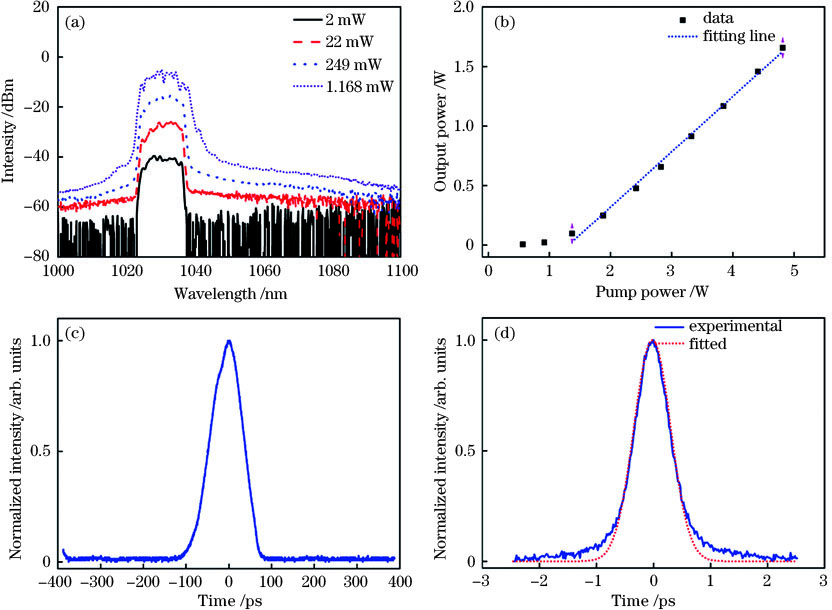
图 5. 20 μm /125 μm双包层放大器的脉冲参数。 (a)不同输出功率下的光谱变化;(b)输出功率随泵浦功率的变化;(c)输入脉宽;(d)放大后的压缩脉宽
Fig. 5. Output parameters of 20 μm /125 μm double-cladding amplifier. (a) Spectra at different output powers; (b) output power versus pump power; (c) input pulse duration; (d) compressed pulse duration after amplification
4 结论
通过优化设计腔内保偏无源光纤的长度和位置并调控腔内的非线性积累,实现了重复频率为100 kHz~21 MHz 的全保偏非线性放大环形镜锁模。输出的脉冲具有高能量、宽带宽和可压缩等特性。当重复频率为21.16 MHz时,直接实现了脉宽为5.3 ps、光谱带宽为9.1 nm的锁模,并且脉宽被压缩至352 fs; 当重复频率为5.92 MHz时,获得的最宽光谱带宽为30 nm,脉宽可以被压缩至177 fs。在低重复频率下获得的单脉冲能量为nJ至几百nJ,其中100 kHz锁模是目前报道的具有最低重复频率的非线性放大环形镜锁模,其300 ps的输出脉宽具有可被压缩至亚皮秒量级的能力。最后基于重复频率为388 kHz的NALM锁模种子源,利用单个光纤放大级就实现了3 μJ的能量输出,压缩后的脉宽为537 fs,峰值功率为4.47 MW,整个激光器系统没有使用任何脉冲选择器件、展宽器和多级光纤预放大级,结构十分紧凑。
[2] 鄢朋朋, 贡航, 叶飞, 等. 全保偏掺铒光纤光梳[J]. 中国激光, 2020, 47(1): 0115001.
[3] Liu G Y, Jiang X H, Wang A M, et al. Robust 700 MHz mode-locked Yb:fiber laser with a biased nonlinear amplifying loop mirror[J]. Optics Express, 2018, 26(20): 26003-26008.
[4] Qiu J. Teichman J M H, Wang T, et al. Femtosecond laser lithotripsy: feasibility and ablation mechanism[J]. Journal of Biomedical Optics, 2010, 15(2): 028001.
[6] Zhou T, Ruppe J, Zhu C, et al. Coherent pulse stacking amplification using low-finesse Gires-Tournois interferometers[J]. Optics Express, 2015, 23(6): 7442-7462.
[7] Sun R, Jin D, Tan F, et al. High-power all-fiber femtosecond chirped pulse amplification based on dispersive wave and chirped-volume Bragg grating[J]. Optics Express, 2016, 24(20): 22806-22812.
[8] Yang P L, Hao T, Hu Z Q, et al. Highly stable Yb-fiber laser amplifier of delivering 32-μJ, 153-fs pulses at 1-MHz repetition rate[J]. Applied Physics B, 2018, 124(8): 1-6.
[9] 孙若愚, 谭方舟, 金东臣, 等. 基于色散波的1 μm飞秒光纤啁啾脉冲放大系统[J]. 中国激光, 2018, 45(1): 0101001.
[10] Renninger W H, Chong A, Wise F W. Giant-chirp oscillators for short-pulse fiber amplifiers[J]. Optics Letters, 2008, 33(24): 3025-3027.
[13] Tian X L, Tang M, Shum P P, et al. High-energy laser pulse with a submegahertz repetition rate from a passively mode-locked fiber laser[J]. Optics Letters, 2009, 34(9): 1432-1434.
[14] Li X H, Liu X M, Hu X H, et al. Long-cavity passively mode-locked fiber ring laser with high-energy rectangular-shape pulses in anomalous dispersion regime[J]. Optics Letters, 2010, 35(19): 3249-3251.
[16] Xiao X S. Low-repetition-rate, all-polarization-maintaining Yb-doped fiber laser mode-locked by a semiconductor saturable absorber[J]. Chinese Physics B, 2017, 26(11): 114204.
[18] Kelleher E J, Travers J C, Ippen E P, et al. Generation and direct measurement of giant chirp in a passively mode-locked laser[J]. Optics Letters, 2009, 34(22): 3526-3528.
[20] RaabeN, MeroM, Song YJ, et al. Detecting determinism in laser noise: a novel diagnostic approach for ultrafast lasers[C]//2016 Conference on Lasers and Electro-Optics (CLEO), June 5-10, 2016, San Jose, CA, USA. New York: IEEE, 2016: 728- 749.
[21] Liu Z W, Ziegler Z M, Wright L G, et al. Megawatt peak power from a Mamyshev oscillator[J]. Optica, 2017, 4(6): 649-654.
[22] Sidorenko P, Fu W, Wright L G, et al. Self-seeded, multi-megawatt, Mamyshev oscillator[J]. Optics Letters, 2018, 43(11): 2672-2675.
[23] Ma CY, KhanolkarA, Zang YM, et al. Ultrabroadband, few-cycle pulses directly from a Mamyshev fiber oscillator[J]. Photonics Research, 2020( 1): 65- 69.
[26] 杨松, 郝强, 曾和平. 非线性放大环形镜被动锁模光纤激光器重复频率精确锁定研究[J]. 中国激光, 2018, 45(8): 0801007.
[27] Bowen P, Erkintalo M, Provo R, et al. Mode-locked Yb-doped fiber laser emitting broadband pulses at ultralow repetition rates[J]. Optics Letters, 2016, 41(22): 5270-5273.
[29] 周佳琦, 潘伟巍, 张磊, 等. 非线性环路反射镜锁模光纤激光器的研究进展[J]. 中国激光, 2019, 46(5): 0508013.
[30] Hänsel W, Hoogland H, Giunta M, et al. All polarization-maintaining fiber laser architecture for robust femtosecond pulse generation[J]. Applied Physics B, 2017, 123(41): 1-6.
石宇航, 程昭晨, 彭志刚, 游雨, 夏童, 王璞. 21 MHz~100 kHz重复频率亚皮秒NALM锁模光纤激光器[J]. 中国激光, 2021, 48(5): 0501013. Yuhang Shi, Zhaochen Cheng, Zhigang Peng, Yu You, Tong Xia, Pu Wang. Sub-Picosecond NALM Mode-Locked Fiber Laser with 21 MHz-100 kHz Repetition Rate[J]. Chinese Journal of Lasers, 2021, 48(5): 0501013.