Compact phase-lock loop for external cavity diode lasers
Download: 998次
Phase-coherent lasers play an important role in many atomic physics experiments, such as high-resolution atomic spectroscopy[1,2], quantum communication[3], Raman velocity selection[4], and atom interferometry[5]. Especially in atom interferometry, Raman pulses are generated with phase-coherent lasers. If the required frequency difference is relatively small, the phase coherence between laser beams can easily be produced by directly diffracting a single laser with an acousto-optic or an electro-optic modulator to shift its frequency while conserving the phase. However, when the required frequency difference increases past the gigahertz level, it is often preferable to use two separate sources. In this case, an optical phase-lock loop (OPLL) is introduced to synchronize the phase between two individual lasers[6,7]. Based on this method, the frequency difference of the two lasers is tunable up to several gigahertz[8,9].
In the last two decades, a number of OPLL designs have been proposed and implemented[10
In this Letter, we present a compact, low-noise, and digital OPLL with two ECDLs. The OPLL presented here is made from inexpensive off-the-shelf components. Compared with the method of frequency mixing, we chose a divider to down-convert the beat note signal and design an ultra-low phase noise radio-frequency (RF) generator as the reference signal. In this way, we avoided the use of an extra commercial microwave and RF generator. The whole cost of the OPLL board and reference signal board is less than 700 dollars. In order to test the performance of our OPLL, we lock a slave laser (SL) to a master laser (ML) with a frequency difference of approximately 6.834 GHz. A mean-square phase error of approximately
The structure of our digital OPLL is shown in Fig.
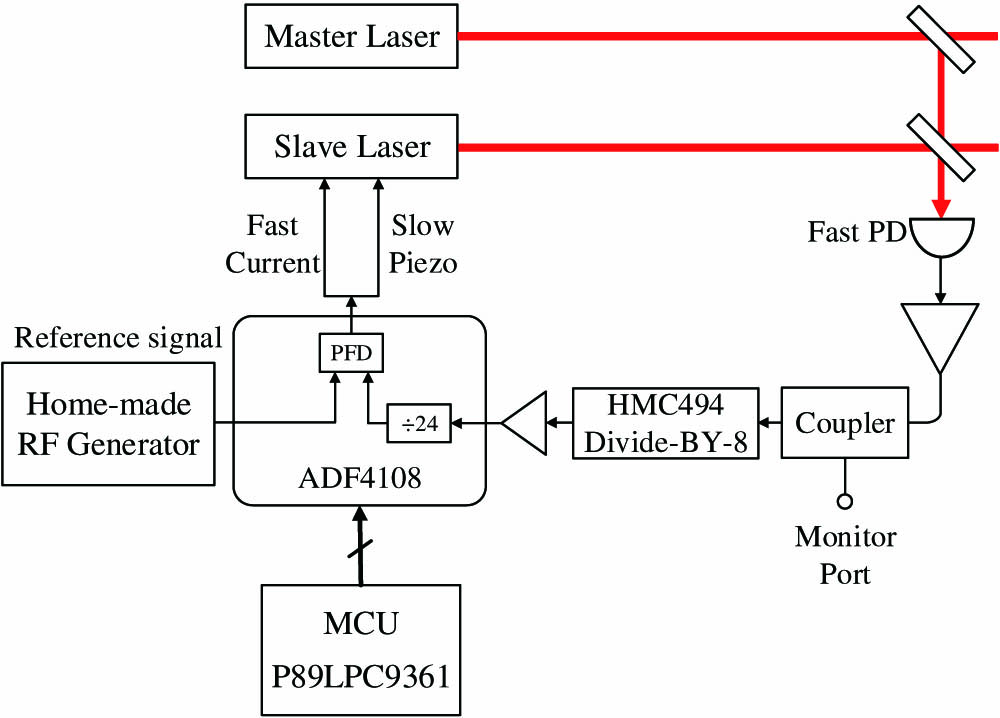
Fig. 1. Structure of OPLL. PFD, phase frequency detector; MCU, microcontroller unit; Fast PD, fast photodiode.
The output optical beams from the ML and SL are overlapped by a beam splitter and sent to an ultrafast photodiode (G4176, Hamamatsu) with a sensitivity of 0.3 A/W @780 nm for generating the beat note signal. The total optical power of the two lasers that reaches the photodiode is about 2 mW. After a wideband (0.2 MHz to 12 GHz) and high-speed response bias tee (ZX85-12G+, Mini-Circuits), the beat note signal at about 6.834 GHz is amplified by a low-noise, ultra-wideband (0.01 to 12 GHz), and excellent gain flatness (
After the pre-amplifier, we use a prescaler (HMC494, ADI) to realize down-conversion. HMC494 is a low-noise divide-by-8 static divider. It has ultra-low single sideband (SSB) phase noise (
The divided and amplified beat note signal is sent into a low-noise digital phase and frequency detector chip (ADF4108, ADI) and then it is compared with a reference signal. ADF4108 has a very high bandwidth (up to 8 GHz). This chip could divide the beat signal by a factor
The digital phase and frequency detector produces a phase error signal by comparing the phase of the down-converted beat note signal with that of the reference signal from the homemade RF generator. The phase error signal is divided into two paths for the slow and fast feedback loops of the OPLL. The fast loop acts directly on the injection current of the slave ECDL to suppress high-frequency fluctuations in synchronization. An resistance capacitance (RC) phase-advanced filter is used here to balance the roll-off in the diode laser response and therefore to extend the loop bandwidth. Long-term corrections are performed by changing the cavity length of the slave ECDL through the slow loop. As the ECDLs and optical elements can be regarded as an equivalent voltage-controlled oscillator (VCO), a complete OPLL has been implemented: it consists of a digital phase frequency detector (DPFD), an external loop filter, and a VCO. Detailed circuit diagrams circuits have been uploaded to the internet[18]. The size of our OPLL board is
There are many commercially available RF generators, but generally they are large and expensive. In addition, much of their phase noise generally does not meet the requirements of a high-performance OPLL. A direct digital synthesizer (DDS) can be well-integrated on-chip with an excellent performance and is capable of providing a trigonometric output with a high frequency resolution, low phase noise, and continuous phase and frequency modulation. We have designed a programmable reference signal source based on the DDS technique. In order to reduce the phase noise of the reference signal, an oven-controlled crystal oscillator (OCXO) is selected as the reference clock of the DDS. The OCXO (O23B, DAPU) has an ultra-low phase noise, a temperature stability of
The schematic configuration of the homemade RF generator is shown in Fig.
The structure of this system is simple and clear. Aside from the ECDLs and the optical elements for the beat measurement, the loop is implemented digitally through two boards. The homemade RF generator board provides a low-phase-noise reference signal to the OPLL board. The OPLL board outputs fast and slow feedback signals to the SL to close the phase loop.
The advantages of our structure are obvious: the use of a divider instead of a microwave mixer to down-convert the beat note saves the use of a microwave source. We locked an SL to an ML by using just two boards without any additional electronic equipment (excluding the control computer). In our system, we can realize a low-noise phase-lock loop with a large frequency differences without the need for an extra commercial microwave or an RF signal source.
With the monitor port on the OPLL board, the beat note signal can be checked when the loop is closed. First, the spectrum of the closed-loop beat note signal is obtained through a spectrum analyzer. A typical 6.834 GHz closed-loop beat note signal is shown in Fig.
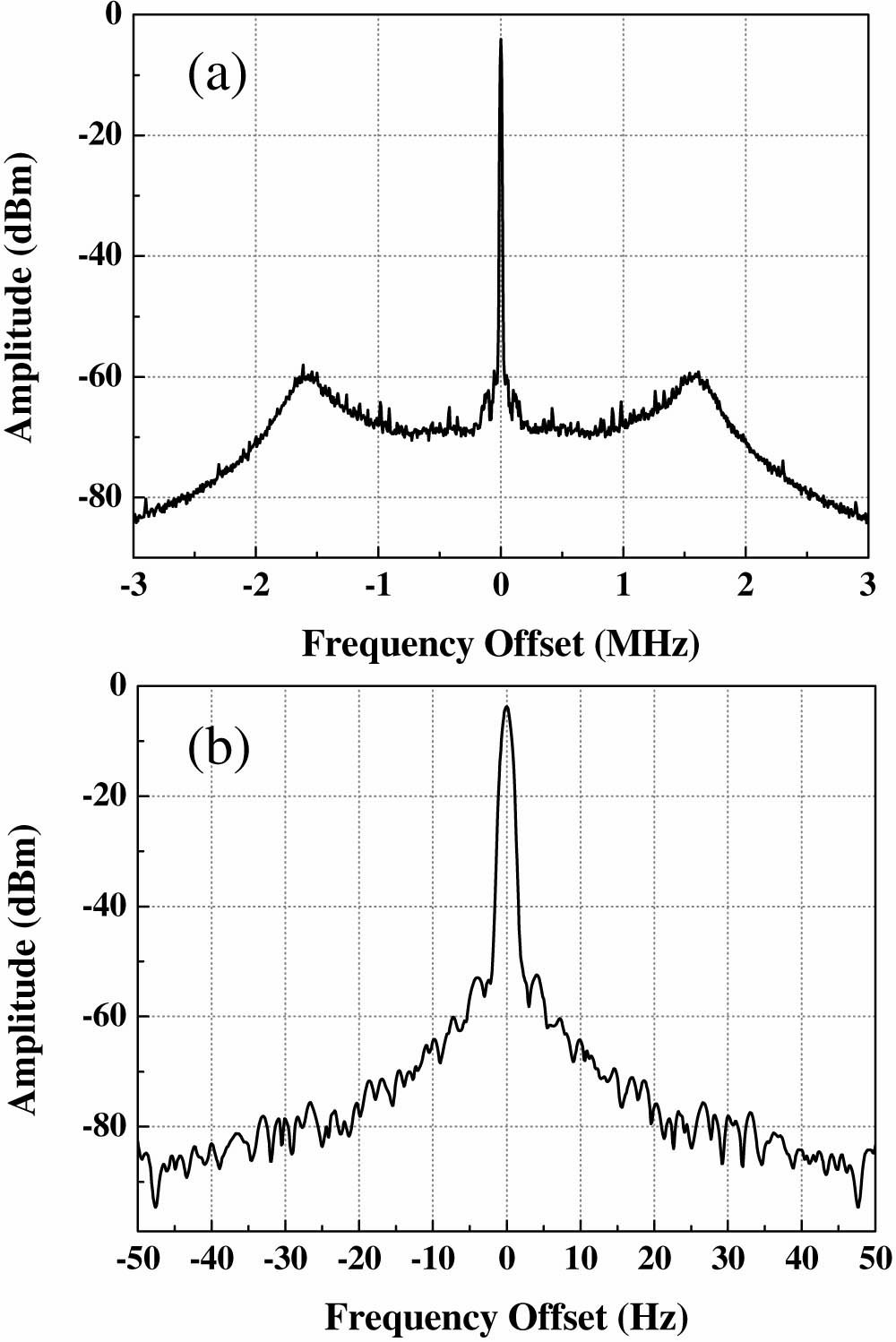
Fig. 3. (a) A typical spectrum of the closed-loop beat note signal (resolution bandwidth is 10 kHz). (b) Expanded view of the beat note signal (resolution bandwidth is 1 Hz)
The beat note signal at a span of 100 Hz is shown in Fig.
Phase noise is an important index to evaluate the performance of a phase-locked loop. In our OPLL system, the phase noise of the beat note signal depends on the phase noise of the homemade DDS signal source, the noise introduced by the feedback circuit, and the feedback bandwidth. By choosing components with good performance, the noise of the circuit can be reduced. We select an ultra-low phase noise OCXO as the reference clock of the RF generator to reduce the phase noise of the reference signal source.
The phase noise of the homemade RF generator at the operating frequency is shown in Fig.
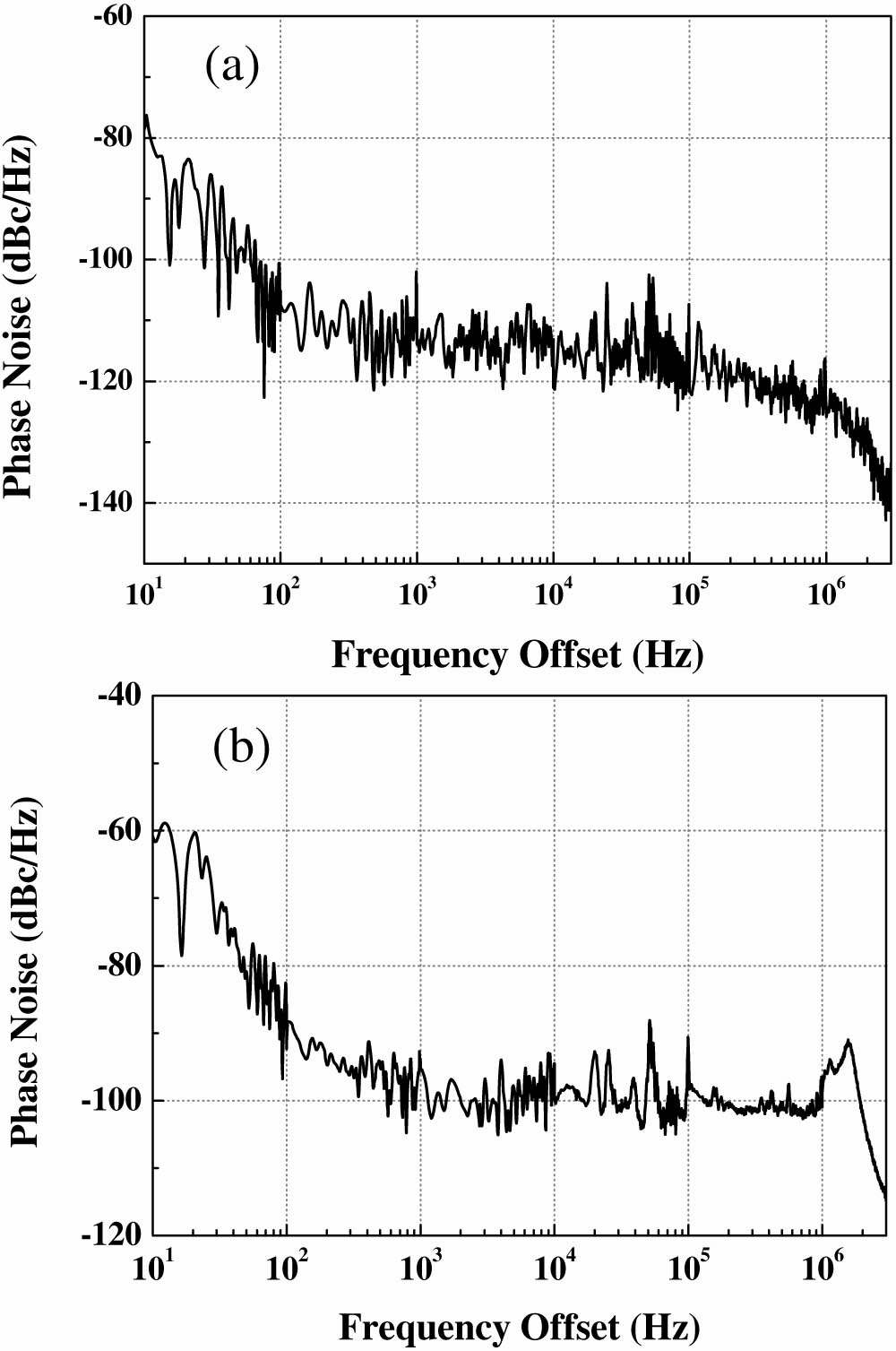
Fig. 4. (a) Phase noise spectrum of the reference signal. (b) Phase noise spectrum of the OPLL.
We also calculate the mean-square phase error
The dynamic performance of the OPLL can be tested through monitoring the amount of time the OPLL requires to recover phase locking after a sudden changes in the reference. It is an indication of the frequency turning agility. When the OPLL was stably locked, we applied frequency hopping on the reference signal to create a frequency difference of about 20 MHz. Then the output of the OPLL was recorded; it is drawn in Fig.
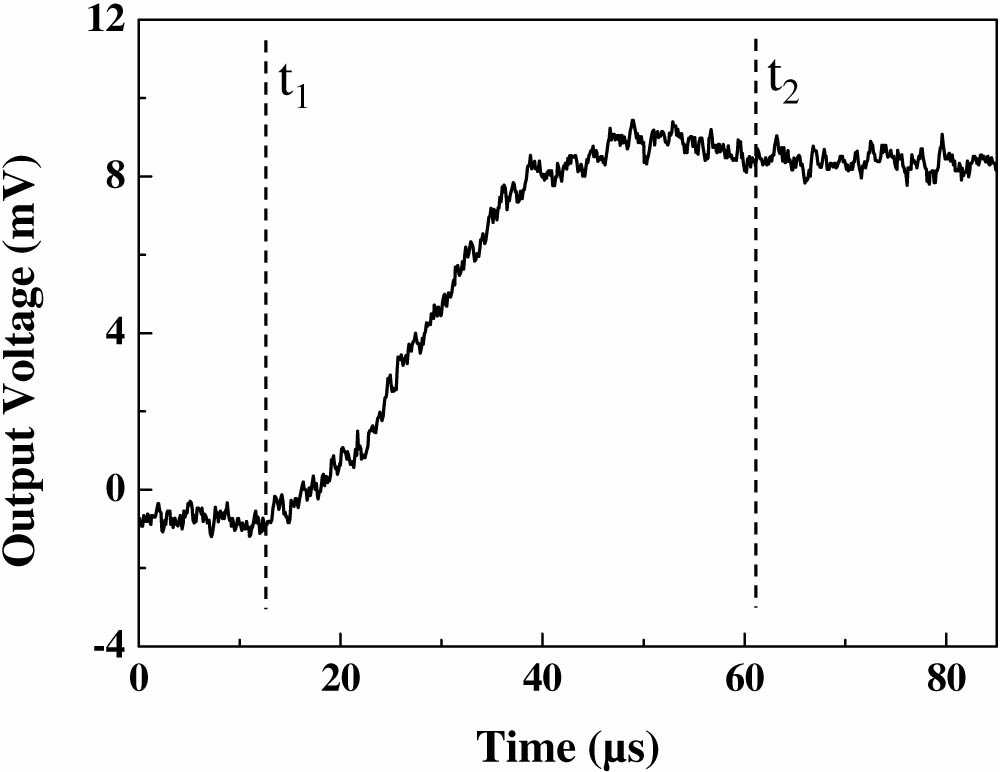
Fig. 5. Dynamic performance of the OPLL. Transition time between and is approximately 50 μs.
In conclusion, we present a compact, low-noise, and inexpensive OPLL. Compared with a conventional OPLL, the OPLL uses a divider instead of a microwave mixer to down-convert the beat note signal. In this way, the microwave reference signal is omitted. We also design an ultra-low phase noise RF generator as the reference signal. The whole cost of the two boards is less than 700 dollars. The mean-square phase error is found to be
[1]
[2]
[3]
[4]
[5]
[6]
[7]
[8]
[9]
[10]
[11]
[12]
[13]
[14]
[15]
[16]
[17]
Chunhua Wei, Shuhua Yan, Aiai Jia, Yukun Luo, Qingqing Hu, Zehuan Li. Compact phase-lock loop for external cavity diode lasers[J]. Chinese Optics Letters, 2016, 14(5): 051403.