太赫兹立体相位光栅衍射特性分析
下载: 543次
1 Introduction
Due to the lack of active radiation sources and beam-splitting devices with wide spectrum in terahertz band, there are few kinds of spectral imaging instruments in terahertz band. In particular, as the key component of spectral imaging instrument, the optical splitter directly affects the performance, structural complexity, weight and volume of the instrument[1-3]. The existing optical splitters of visible and infrared spectroscopic instruments include prism, amplitude grating, acousto-optic tuned filter, etc. However, these beam-splitting devices, which are suitable for visible and infrared bands, cannot meet the requirements of spectral detection and imaging in terahertz band. Firstly, as a classical optical splitter, the prism has the advantages of wide free spectrum, simple structure, large amount of light, high energy utilization and easy stray-light suppression. However, with the increase of the wavelength in terahertz band, the penetration of electromagnetic waves will increase and the dispersion of materials will become extremely weak. This means that it is no longer feasible to obtain fine spectra by material dispersion in terahertz spectrum detection[4-5]. Secondly, the beam splitting of amplitude grating depends on the diffraction effect. However, the spectral range of a single grating is limited by the overlap of adjacent secondary diffraction spectra. In order to improve the utilization of light energy, the flare grating is adopted and the limited bandwidth of its wavelength further reduces its free spectral range. The terahertz bandwidth (30 μm~3 mm) is nearly 6 000 times the visible bandwidth (0.38 μm~0.75 μm) and 100 times the infrared bandwidth (0.75 μm~30 μm)[6-8]. This determines that the beam-splitting devices in terahertz band must have a wide free spectral range, so the flared grating and amplitude modulated grating are not applicable to terahertz band. Finally, the acousto-optic tuned filter relies on acousto-optic effect to realize diffraction, and has the advantages of small volume, high crystal diffraction rate and large field of view. However, no acousto-optic crystal suitable for terahertz band has been found in the published literature[9-11].
The weak-signal feature of terahertz detection requires terahertz beam splitter with high energy efficiency. To meet the requirements of terahertz spectral imaging for wide spectral range, high efficiency and real-time detection of spectrometers, a Multi-depth Phase Modulation Grating (MPMG) in terahertz band is proposed in this paper[12]. The phase modulation of incident light is realized by introducing optical path difference resulted from the change of groove depth, so that different regions of reflecting terahertz wave front have different phase information. The typical characteristic of MPMG is that its 0th-order diffraction light carries the phase information due to groove depth modulation and therefore has the ability of splitting light.
2 Diffraction field distribution of MPMG
The MPMG is composed of a series of grating cells whose groove depths vary in an equal gradient. The one-dimensional MPMG and two-dimensional MPMG, whose structures are shown in
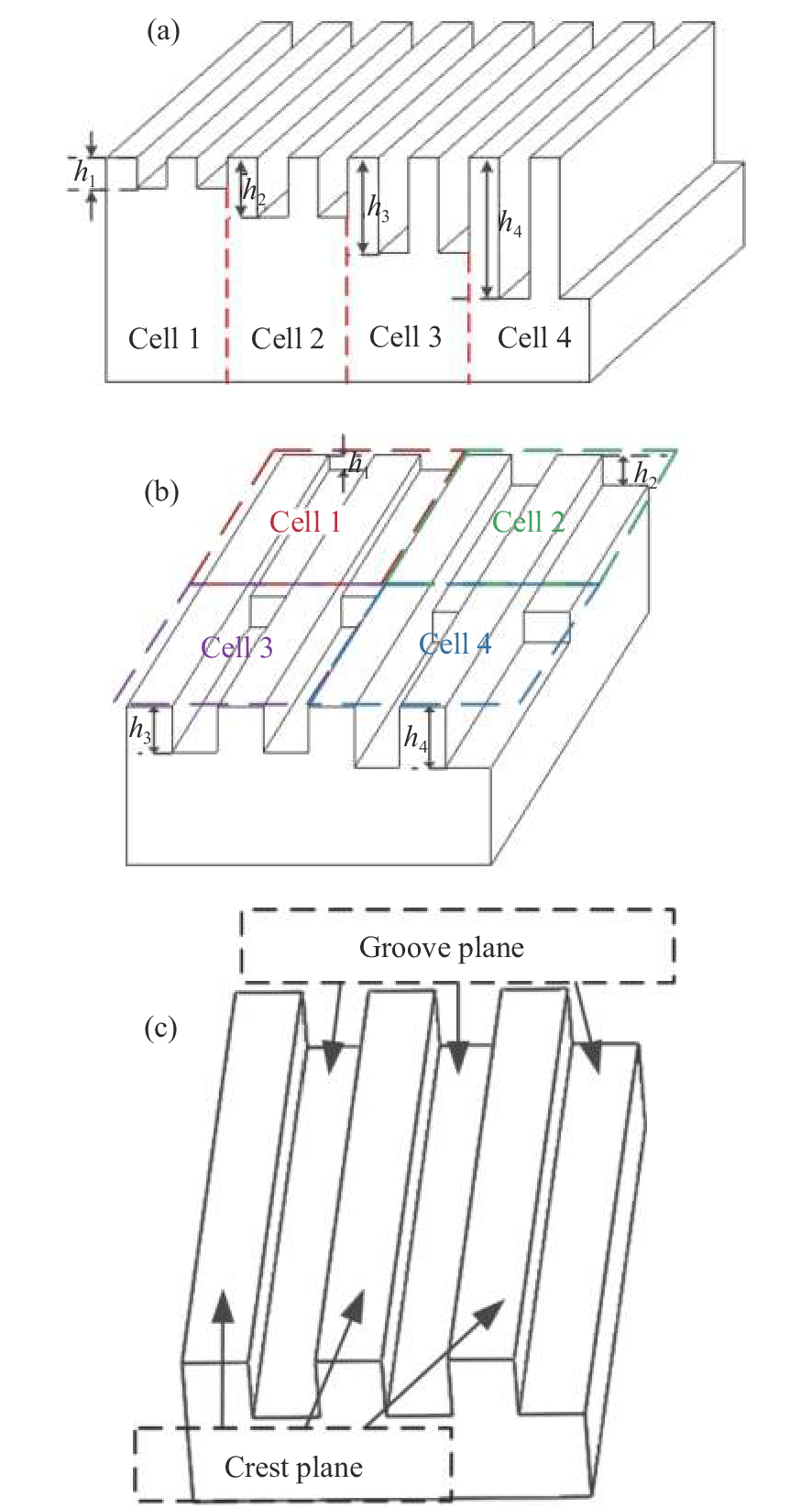
图 1. MPMG示意图(a)一维MPMG,(b)二维MPMG(c)光栅单元
Fig. 1. Schematic diagram of MPMG. (a) 1D MPMG, (b) 2D MPMG, (c) grating cell
The grating cells are equivalent to a series of planes staggered by different phase differences, as shown in

图 2. (a) MPMG等效为平面透射光栅示意图 (b) MPMG衍射示意图
Fig. 2. (a) Reflection grating simulated as a plane transmission grating; (b) MPMG diffraction diagram
When the terahertz wave is incident in the direction parallel to the paper, that is ψ=0, the normalized light intensity distribution after the MPMG diffraction is shown in Eq. (2). The crest reflection planes and groove reflection planes are considered to be with the same width during calculation.
α and β represent the diffraction angle in the x direction and the diffraction angle in the y direction respectively;
That is, the diffraction order distribution of the grating only depends on the grating constant d. When the diffraction light with the same order, the higher d corresponds to a larger diffraction angle.
3 Analysis of MPMG diffraction characteristics
As known from Eq. (3), the intensity distribution of diffraction field along the X-axis is related to the grating constant d (d=2w), the number of crest reflection plane and trough reflection plane pairs (n), and the groove depth (h). The

图 3. 不同相位调制下沿x 轴方向的光强分布
Fig. 3. Light intensity distributions along the x axis under different phase modulation conditions
4 Testing of MPMG diffraction characteristics
We established a set of experimental facilities for evaluating the diffraction characteristics of MPMG in the laboratory, including a high-power THz radiation source system,
表 1. 一维MPMG参数
Table 1. Parameters of 1D MPMG
|
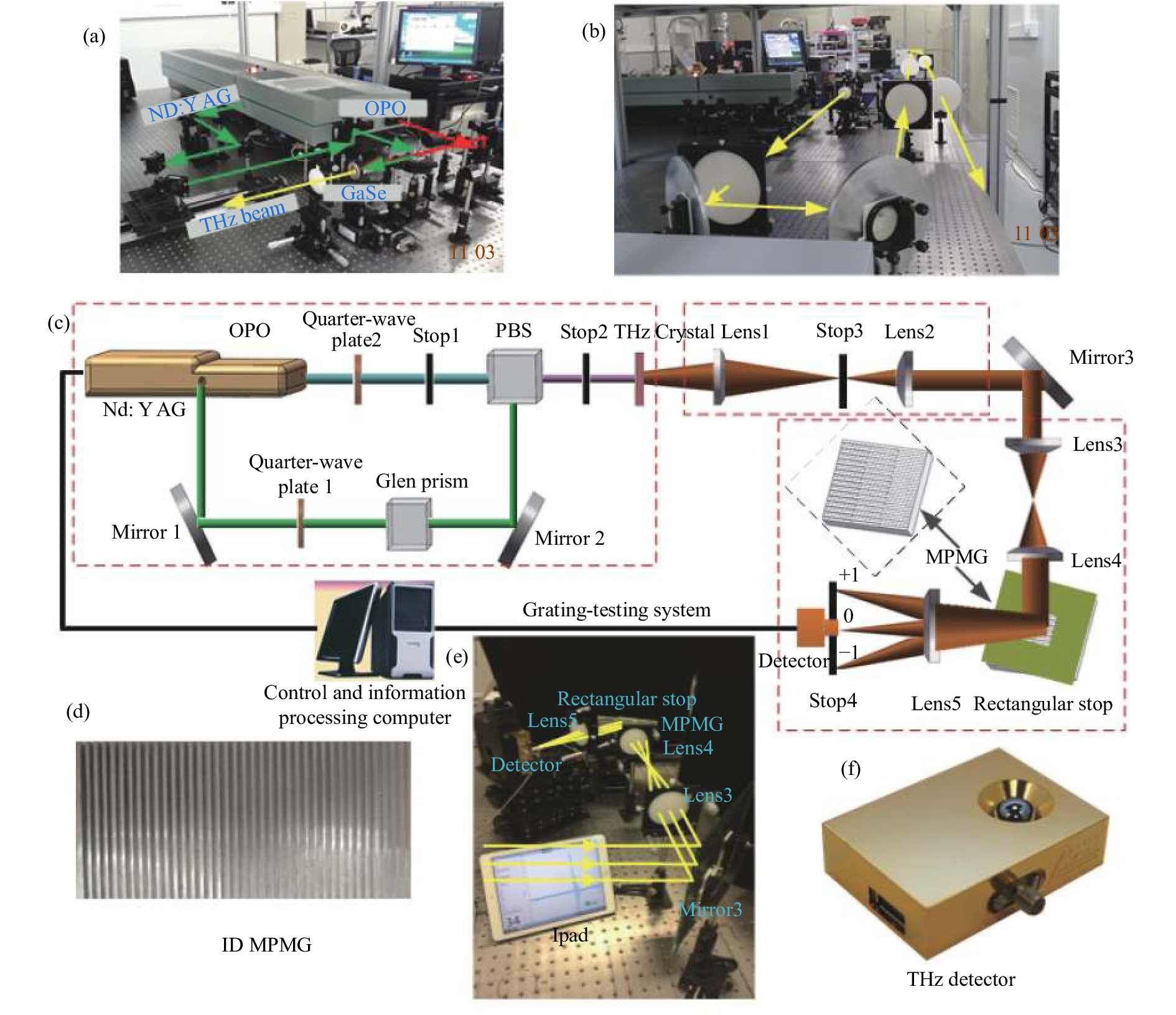
图 4. (a) 高功率辐射源系统;(b) 激光准直与发射系统;(c) 实验方案;(d) 一维MPMG;(e) 光栅测试系统;(f) 探测器照片
Fig. 4. (a) High-power THz radiation source system. (b) Laser collimation and transmission system. (c) Schematic of experiment. (d) 1D MPMG. (e) Grating-testing system. (f) Photograph of the THz detector.
The test method is described below. After being collimated by the lens group, the 0.5 THz radiation is parallelly incident to and diffracted by the MPMG (θ = 60°, ψ = 0°). After being converged by the THz lens, the diffracted waves at the 0th- and ±1st-order are detected by the THz detector in the focal plane of the lens. A thin rectangular stop is placed on the front surface of the MPMG to ensure that only one cell is effectively illuminated by each incident THz wave. By moving the detector in proper order, the diffraction intensities at the 0th- and ±1st-order can be recorded. By repeating this operation for each grating cell, the intensities of all the grating cell at the 0th- and ±1st-order can be obtained. In order to eliminate the influence of laser jitter on the measurement results, the measurement results of multiple tests (20 measurements) are averaged. By normalizing the measured data, the 0th- and ±1st-order diffraction efficiencies of 8 grating cells can be obtained.
By changing the OPO wavelength, the diffraction intensity of the 0.34 THz radiation wave in 8 grating cells is measured. The theoretical simulation curves and measurement results of diffraction efficiencies of 0.5 THz and 0.34 THz radiation in the grating cells are shown in

图 5. 光栅单元0级和1级衍射效率的模拟和测试结果(a) 0.34 THz (b) 0.5 THz
Fig. 5. Simulation and test results of 0th- and 1st-order diffraction efficiency for each grating cell at (a) 0.34 THz and (b) 0.5 THz
5 Conclusion
In this paper, a new MPMG in THz band, which is composed of a series of grating cells, is presented. The groove-depth gradients of these grating cells correspond to different positions of the moving mirrors in the Fourier transform spectrum system. The calculation results of Fraunhofer diffraction field distribution and diffraction efficiency of MPMG show that, the 0th-order diffracted light of MPMG carries the phase information and its diffraction intensity is modulated by the phase introduced by groove depth. We develop the MPMG composed of eight grating cells and test its 0th- and 1st-order diffraction efficiencies at 0.5 THz and 0.34 THz. The test results agree well with the simulation results. Therefore, we believe that the 0th-order diffracted light of MPMG carries the phase information and its diffraction intensity is modulated by the phase introduced by groove depth.
1 引 言
太赫兹波段的光谱成像仪器种类稀少,这是由于太赫兹宽谱主动辐射光源与太赫兹宽谱分光器件缺乏所共同导致的。其中分光器件作为光谱成像仪器的关键部件,直接影响仪器的性能、结构的复杂程度、重量和体积等[1-3]。目前,可见、红外光谱仪器的分光器件有:棱镜、振幅光栅、声光调谐滤波器等。然而,这些适用于可见、红外波段的分光器件并不能满足太赫兹波段物质的谱探测与成像对分光器件的需求。棱镜作为经典的分光器件具有自由光谱范围宽、结构简单、通光量大,能量利用率高,杂散光易于抑制的优点,然而在太赫兹波段,随着波长的增加,电磁波的穿透性能增强,材料的色散现象变的极其微弱,这意味着通过材料色散获取精细光谱的方式在太赫兹谱探测中不在可行[4-5]。振幅光栅是依靠衍射效应分光的,然而受相邻衍射次级光谱重叠的限制,单块光栅的光谱范围有限,为了提高光能利用率而采用的闪耀光栅,其闪耀波长的带宽有限,进一步缩小了光栅的自由光谱范围。太赫兹(30 μm~3 mm)的频段带宽是可见(0.38 μm~0.75 μm)频段带宽的近6 000倍,是红外(0.75 μm~30 μm)频段带宽的100倍[6-8]。这决定了太赫兹波段的分光器件必须具备宽自由光谱范围,因此闪耀光栅、振幅调制光栅不适合用作太赫兹波段的分光器件。声光调谐滤波器依靠声光效应实现衍射分光,其具有体积小,晶体衍射率高、大视场的优点,但目前尚未在公开报道的文献中查阅到适用于太赫兹波段的声光晶体[9-11]。
若要使太赫兹探测到弱信号,则要求太赫兹分光器件必须具备高能量利用效率。针对太赫兹谱成像对宽光谱、高光能利用率、实时探测分光器件的需求,本文提出一种太赫兹立体相光栅(MPMG)分光器件[12],通过刻槽深度的变化引入光程差,实现对入射光的相位调制,使反射太赫兹波前的不同区域具有不同的相位信息。该MPMG的典型特征在于,槽深调制使MPMG的零级衍射光携带相位信息,因此MPMG零级衍射光具备分光能力。
2 MPMG的衍射场分布
MPMG由槽深呈等梯度变化的一系列光栅单元组成。一维MPMG、二维MPMG的结构示意图分别如
将光栅单元等效为一系列不同相位差交错排列的平面,如
当太赫兹波平行于纸面方向入射,即(
即光栅的衍射级次分布仅取决于光栅常数
3 MPMG的衍射特性分析
由式(3)可知,沿x轴的衍射场强度分布与光栅常数
4 MPMG的衍射特性测试
在实验室建立了一套评价MPMG衍射特性的实验装置。验证装置包括高功率THz辐射源系统(
试验方法具体为:0.5 THz辐射经透镜组的准直,平行入射到MPMG(θ = 60°,ψ = 0°)上,并被MPMG衍射,衍射波经THz透镜汇聚后,在透镜焦平面上用THz探测器对0级和±1级衍射波进行检测。在MPMG的前表面放置一个很薄的矩形挡板,以确保每次只有一个单元被入射的THz波有效地照亮。通过依次移动探测器的位置,记录了0级和±1级的衍射强度。对每个光栅单元重复此操作,得到了所有光栅单元的0级和1级强度。为了消除激光抖动对测量结果的影响,采用多次测试的方法对测量结果进行了平均,所得结果是20次测量的平均值。将所测量数据进行归一化处理,可以得到8个光栅单元的0阶和±1阶衍射效率。
通过改变OPO的波长,测试了8个光栅单元对0.34 THz辐射波的衍射光强。光栅单元对0.5 THz、0.34 THz辐射的衍射效率理论模拟曲线和测量结果如
5 结 论
本文介绍了一种THz波段的新型MPMG,它由一系列光栅单元组成,这些光栅单元的槽深梯度与傅立叶变换光谱系统中动镜的不同位置相对应。对MPMG的夫琅和费衍射场分布和衍射效率的计算表明,MPMG的零级衍射光具有相位信息,其衍射强度由槽深引入的相位调制。接着,研制了由8个光栅单元组成的MPMG,对其在0.5 THz、0.34 THz下的零级和一级衍射效率进行了测试,结果与模拟结果吻合较好。由此可知,MPMG的零级衍射光携带相位信息,其衍射强度由槽深引入的相位调制。
[2] LUO Y C, LIU X X, HAYTON D J, et al.. Fourier phase grating f THz multibeam local oscillats[C]. Proceedings of the 26th International Symposium on Space Terahertz Technology, International Symposium on Space Terahertz Technology, 2015: 7778.
[3] MIRZAEI B, SILVA J R G, LUO Y C. Efficiency of multi-beam Fourier phase gratings at 1.4 THz[J]. Optics Express, 2017, 25(6): 6581-6588.
[7] MANZARDO O, MICHAELY R, SCHÄDELIN F. Miniature lamellar grating interferometer based on silicon technology[J]. Optics Letters, 2004, 29(13): 1437-1439.
[12] 中国科学院上海技术物理研究所. 一种太赫兹一维立体相位光栅: 中国, CN106125176B[P]. 20180626.Shanghai Institute of Technical Physics of the Chinese Academy of Sciences, CAS. Terahertz onedimensional stereo phase grating: CN, CN106125176B[P]. 20180626. (in Chinese)
[13] HUANG J G, HUANG ZH M, TONG J CH. Intensive terahertz emission from GaSe0.91S0.09 under collinear difference frequency generation[J]. Applied Physics Letters, 2013, 103(8): 081104.
[15] HUANG Z M, HUANG J G, GAO Y Q, et al.. Highresolution terahertz spectrometer with up to 110 m singlepass base[C]. Proceedings of 2016 IEEE International Conference on Infrared, Millimeter, Terahertz Waves, IEEE, 2016: 12.
[16] XIAO ZH Y, YANG Q J, HUANG J G. Terahertz communication windows and their point-to-point transmission verification[J]. Applied Optics, 2018, 57(27): 7673-7680.
Article Outline
杨秋杰, 何志平, 糜忠良. 太赫兹立体相位光栅衍射特性分析[J]. 中国光学, 2020, 13(3): 605. Qiu-jie YANG, Zhi-ping HE, Zhong-liang MI. Diffraction characteristics analysis of multi-depth phase modulation grating in terahertz band[J]. Chinese Optics, 2020, 13(3): 605.