The influence of the characteristics of a collection of particles on the scattered spectral density and its applications
Download: 1163次
The far-zone property of light waves on scattering from a medium is a topic of considerable importance due to its potential applications in areas such as remote sensing, detecting, and medical diagnosis. During the past two decades, a lot of papers that discussed the relationship between the character of a scattering medium and the property of the far-zone scattered field were published. For example, the spectrum of polychromatic light waves on scattering both from a continual medium[1,2] and from a collection of particles[3] was discussed, and a condition for the isotropy of the far-zone scattered spectrum was presented by Wolf[4]. The spectral coherence of a light wave on scattering from a quasi-homogenous medium was discussed by Visser
In any discussion of light wave scattering, the inverse problem, i.e., the determination of the characteristics of a scatterer from measurements of the far-zone scattered field, is always a highlight[13,14]. In 2007, Zhao and his collaborators presented a method to reconstruct the correlation function of a homogeneous medium from the measurement of the far-zone scattered spectral density[15]. Soon afterwards, this method was generalized to reconstruct the correlation function of a quasi-homogeneous medium[16]. In practice, the scatterer one encounters is not always a continuous medium, but a collection of particles. In this case, one should determine the number, the type, and the location of each particle in the collection to reconstruct the structure of the collection. In this manuscript, the relationship between the characteristics of a collection of particles and the distribution of the far-zone scattered spectral density will be discussed. It will be shown that the far-zone scattered spectral density is closely related to the number of particles and their locations in the collection. This phenomenon may have potential application in the reconstruction of the structure of a collection of particles.
Assuming that a spatially coherent polychromatic plane light wave with a propagation direction specified by a unit vector
Let us assume that the scattering medium is composed of a collection of particles. In the case that all of the particles and their locations in the collection are deterministic, then the scattering potential
Assuming that the scatter is weak so that the scattering can be analyzed within the accuracy of the first-order Born approximation[19]. Then, the cross-spectral density function of the far-zone scattered field, at two positions specified by a pair of position vectors
The far-zone scattered spectral density can be obtained from the cross-spectral density function by letting the two position vectors
As an example, let us assume that the scattering potential of each particle in the collection has a distribution of the Gaussian function[20], i.e.,
Upon substituting the values from Eq. (
Upon substituting the values from Eq. (
As shown in Eq. (
First of all, let us consider the influence of the locations of the particles in the collection on the distribution of the far-zone scattered spectral density. For the sake of simplicity, let us consider a two-particle collection with different intervals (see Fig.
Table 1. Relationship between the Distance of Two Particles and the Scattering Angle at which the First Minimum and the Second Maximum appeared in the Scattered Spectral Density
|
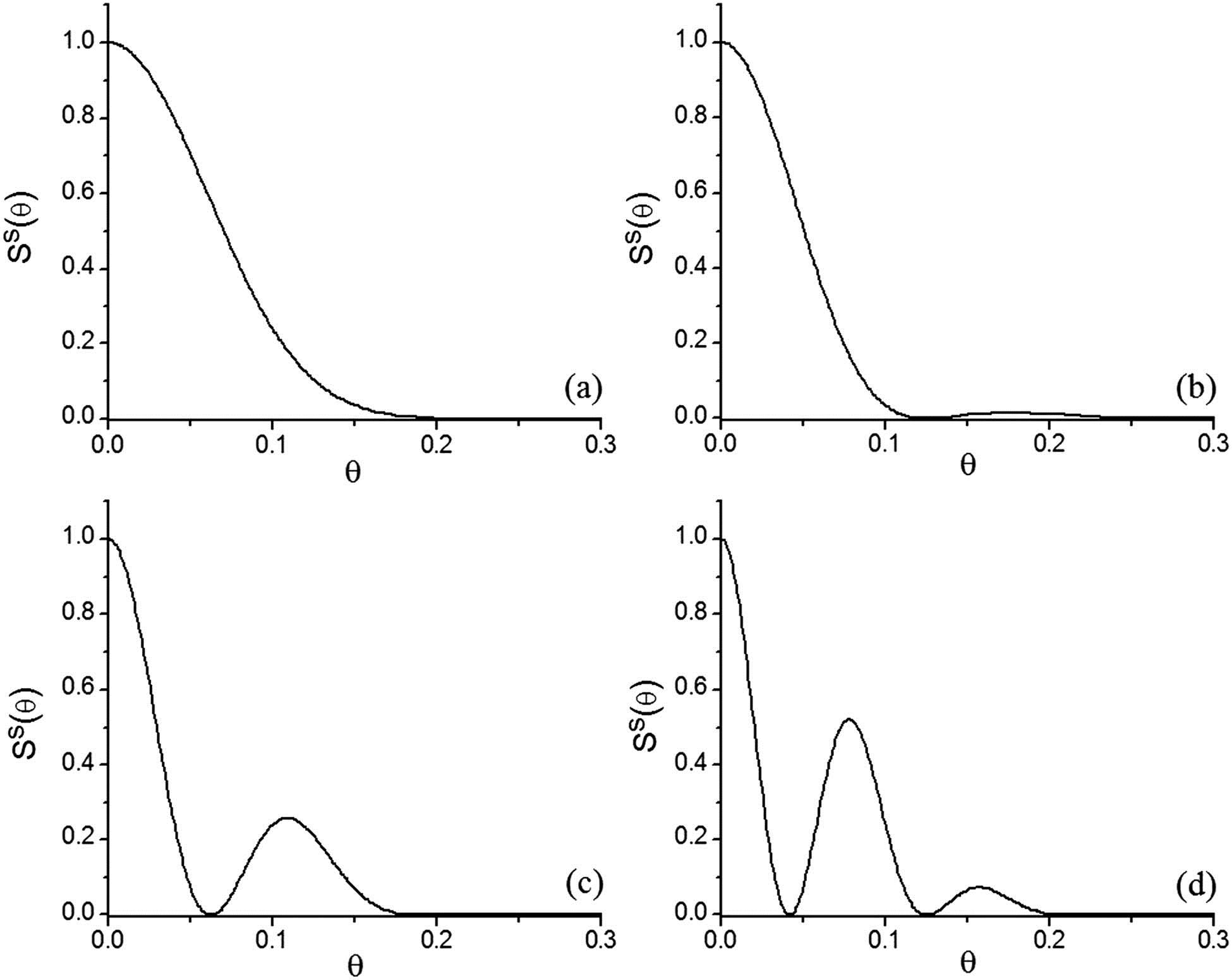
Fig. 2. The normalized scattered spectral density of a light wave on the scattering from a two-particle collection with different intervals corresponding to Fig. 1 . The parameters for the calculations are as follows: , , , and .
Now let us consider the influence of the number of particles on the far-zone scattered spectral density. Assume that all of the particles in the collection are equidistant (see Fig.

Fig. 4. The normalized scattered spectral density of a light wave on the scattering from collection with different numbers of particles corresponding to Fig. 3 . The parameters for the calculations are as follows: , , , and .
Finally, let us discuss the potential application of the above phenomenon, i.e., the reconstruction of the character of a collection, including the number of particles and their locations in the collection from the measurements of the far-zone scattered spectral density. As shown in Fig.
It should be noted that the above discussion is based on a collection of identical spherical particles. This method can be generalized to a collection of spherical particles with different sizes, i.e., particles with different effective widths of their scattering potentials. For a more complex case, i.e., a collection of particles with non-spherical particles, the scattered field should be discussed with the help of a tensor integral, and the density of the scattered field is then anisotropic[21]. In this case, one needs more information about the scattered field to find the structure information of the collection.
In conclusion, we discuss the far-zone spectral density of a light wave on the scattering from a collection of identical isotropic particles with different distributions. It is shown that the far-zone scattered spectral density is closely related to the location and the number of the particles in the collection. This phenomenon may provide a simple and effective method for the determination of the structure of a collection of identical isotropic particles.
[1]
[2]
[3]
[4]
[5]
[6]
[7]
[8]
[9]
[10]
[11]
[12]
[13]
[14]
[15]
[16]
[17]
[19]
Tao Wang, Yi Ding, Xiaoling Ji, Daomu Zhao. The influence of the characteristics of a collection of particles on the scattered spectral density and its applications[J]. Chinese Optics Letters, 2015, 13(10): 102901.