Self-polarization emission based on coherent combination of intracavity eigenmodes in Nd:YAG/Cr4+:YAG lasers
Download: 802次
1. Introduction
The diffusion bonded passively Q-switched lasers have excellent characteristics, including low cost, compact structure, high damage threshold, high peak power, and narrow pulse width[1,2]; thus, they are widely applied in commercial devices. As a typical isotropic laser crystal[3], YAG was supposed to emit lasers with unpolarized or alternate polarization states[4,5], but it is confusing that many papers reported its direct linearly polarized output[6
The polarized laser emission of Q-switched laser was primarily considered to result from the anisotropic saturation absorption effects of the crystal[17,18]. There were many reports about the comparisons of polarization extinction ratio (PER) using with different crystallographic directions[19]. The [110]-cut was proved to represent more significant transmittance anisotropy, thus resulting in more high PER[20]. Il’ichev et al. described a polarization bistable state on such lasers based on self-induced absorption anisotropy in [13,17]. In addition, the pump polarization had also been proved to greatly affect the dynamics of intracavity polarization eigenmodes[21]. Bouwmans discussed the dependencies between laser polarization and pump polarization in two limit cases[15]. It indicated that there was a complex competition between the gain anisotropy, loss anisotropy, and phase anisotropy[22] associated with the polarization pump, saturated absorption, and residual birefringence[23], respectively. Through modes competition, radiation then occurs along preferred directions depending on dominant factors. The resultant polarization regime represents modes with the lowest radiation losses. In this paper, an interesting phenomenon of orientation-dependent beam profiles of polarizers was found. It indicated that the self-polarization output comes from the combined state of two orthogonally polarized intracavity eigenmodes, but not a polarized eigenmode. This work will be helpful for improving the sensibility and instability of polarization in laser devices.
2. Experiments and Results
The experimental configuration for analyzing the polarization in the monolithic 2 mm × 6 mm × laser is shown in Fig. 1. An input mirror film ( at 808 nm and at 1064 nm) was directly deposited onto the input surface of the composite crystal, and an output coupler coating ( at 808 nm and at 1064 nm) was deposited on the outer side of the saturable absorber (SA). The initial transmittance of the SA is 46.78%. A fiber-coupled 808 nm laser diode (LD) without apparent polarization characteristics was used as the pump source. A Glan prism (Ultra Photonics) was used as a polarizer. After passing through the prism, the polarization states of the output laser were measured by a power meter (Thorlabs). Meanwhile, beam profiles were monitored by a CCD camera (DataRay), after passing through two optical wedges (OWs) and a focusing lens (F). Besides, a combination of a polarizing beam splitter (PBS) and a half-wave plate was used to generate a polarized pump with an arbitrary azimuth angle.
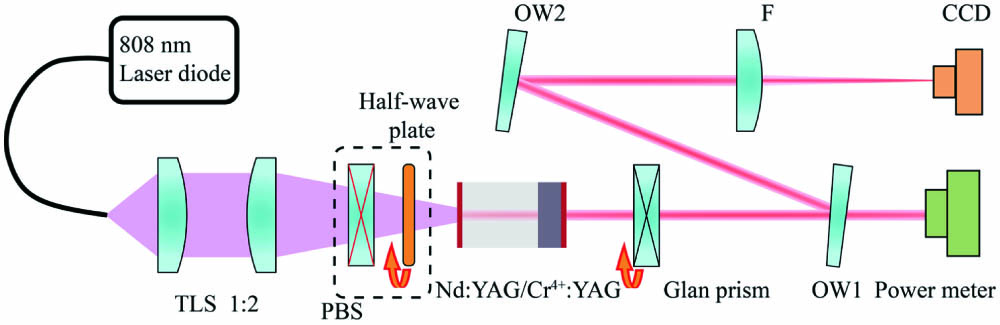
Fig. 1. Schematic diagram for measurement and analysis of linear polarization regime of passively Q-switched laser.
We firstly evaluated the laser polarization performance under a free and polarized pump using the resonator parameters above. The output power and PER as functions of the incident pump power are shown in Fig. 2. During the free pump, vertically linear polarization output with highest PER of about 24 dB was observed near the threshold. Then, the PER started to significantly decrease with the increasing pump power due to the thermal depolarization effect. Under the horizontal polarization pump, the output polarization was horizontal, and highest PER raised to near 26 dB. When the Glan prism was along with the polarization directions of the output lasers, the beam profiles were completely circular spots with approximate Gaussian intensity distribution.

Fig. 2. Output power and polarization extinction ratio (PER) as functions of the incident pump power under (a) free pump and (b) horizontal polarization pump. Insets show the polarization directions and beam profiles when the Glan prism was along the polarization directions.
In order to study the influence of polarization pumps with different angles on the output laser, polarized pumps with arbitrary angles were manipulated continuously by using a combination of a PBS and a half-wave plate. As shown in Fig. 3, when the pump polarization angle was rotated from 0° (horizontal) to 90° (vertical), there was a stable plateau with high PER of about 25 dB in the vicinity of 0° and 90°, respectively, corresponding to horizontal and vertical self-polarization emission. Between the two stable plateaus with orthogonal polarization directions, there was a short transition region with polarization instability and low PER. Continuing to rotate slowly to 180°, the emission was converted back to the horizontal polarization plateau, going through a longer transition region.

Fig. 3. PER versus pump polarization angle. 0° and 90° represent horizontal and vertical pump polarization, respectively, and other angles could be deduced.
Then, we analyzed the beam profiles of self-polarization output under maximum PER. After the laser beams passed through a Glan prism, the beam profiles were monitored by a CCD camera with the rotation of the prism. When the Glan prism was in the extinction direction precisely, two discrete spots could be observed, as in Fig. 4(b); when the prism slightly deviated from the extinction position, they evolved to an inclined elliptical spot in the situation of left or right deviation, respectively, as shown in Figs. 4(a) and 4(c). Moreover, major axes of the two elliptical spots were roughly crossed. In the case of the polarized pump, the pair of inclined elliptical spots was more evident, as depicted in Figs. 4(d)–4(f).

Fig. 4. Profile analyzation of the polarized beam near the extinction position, under (a)–(c) free pump and (d)–(f) horizontal polarization pump. The extinction position was defined as 0°.
3. Simulation and Discussion
Theoretically, there may be two kinds of polarization mechanisms to interpret the self-polarization emission. One is the intrinsic state of linear polarization [Fig. 5(a)], and the other one is the coherent combination state of two orthogonal polarization eigenmodes under fixed phase difference [Fig. 5(b)]. For the former, obviously, the profiles of the beam were almost unchanged with the rotating polarizer, and only the intensity will vary uniformly. The results mentioned above implied that it may not be eigen-polarization radiation like from an anisotropic laser. For the latter, small drifts of birefringence may lead to significant variations of the polarization states. Besides, if the spatial position and intensity distribution of the two eigenmodes are absolutely identical, meaning perfect overlapping, the orientation-dependent beam profiles of the polarizer could not be observed. However, due to aberration or other factors, there are inevitably tiny differences between the two intracavity eigenmodes. In other words, two eigenmodes in the laser cavity could not overlap perfectly in reality. The nonperfect overlapping would result in dislocation of the two vector fields, and unusual performance in the profile analyzation of the polarized beam could be inferred.
The coherent combination of two polarized beams signifies the same frequency between them[24,25]. According to Lamb’s semi-classical theory[26], under the low value of the coupling constant, isotropic lasers generally oscillate in dual-polarization eigenstates with tiny eigenfrequency splitting due to residual anisotropy. However, eigenfrequency degeneracy of intracavity eigenmodes has been proved[27] when the eigenfrequency difference was reduced to a certain extent by manipulating intracavity loss anisotropy. In this description, there was a frequency locking region for two orthogonally polarized eigenstates in the quasi-isotropic laser. These theories provided the possibility of coherent combination of two orthogonally polarized intracavity eigenmodes.
Following this thinking, we built a mathematical model to simulate the profile analyzation of the polarized beam, focusing on the performance of profiles in the process when the two intracavity eigenmodes could not overlap perfectly. We set up two main parameters in the model, including phase anisotropy and loss anisotropy . Among them, represented phase retardance between the and directions caused by residual anisotropy of the laser crystal, and represented anisotropic absorption of for the laser with different polarization. Then, a suit of a round-trip Jones matrix about the polarization states of intracavity eigenmodes was established, by assuming the self-consistency electromagnetic field in the resonator. Starting from the middle of the cavity, the matrix is then
represents the angle between cross coordinate systems of the two kinds of anisotropy. In the resonance condition , and were the eigenvectors and eigenvalues of matrix , corresponding to polarization vectors and relative phase differences of two intracavity eigenmodes after a round trip. The two eigenvectors were multiplied by a Gaussian beam function , containing ellipticity information () and position information () of eigenmodes, to simulate the eigenmodes, so that slight differences in ellipticity and position could be introduced to the two eigenmodes by adjusting the parameters above to manipulate nonperfect overlapping. Then, the two polarized eigenmodes were superposed by the principle vectorial combination:
The vector was then multiplied by a Jones matrix of the polarizer to obtain final optical vector . Then, the calculated intensity of can visualize the whole profile analyzing process of the polarized beam along with the changing of .
Simulated results of profile analyzing near the extinction position are shown in Fig. 6. When was at 0° (extinction position), two circular spots distributed horizontally are observed. When slightly deviated to left or right from 0°, one spot was elongated gradually, and the other one was weakened simultaneously to almost disappear. Then, only an inclined elliptical spot was observed on both near sides of the extinction position, respectively, and the major axes of two elliptical spots were roughly crossed to each other. There was a good agreement between the results of experimental observations and theoretical simulations about the profile analyzation of the polarized beam. That is to say, self-polarization emission was not a polarized eigenstate but a composite state from the coherent combination of eigenmodes essentially.
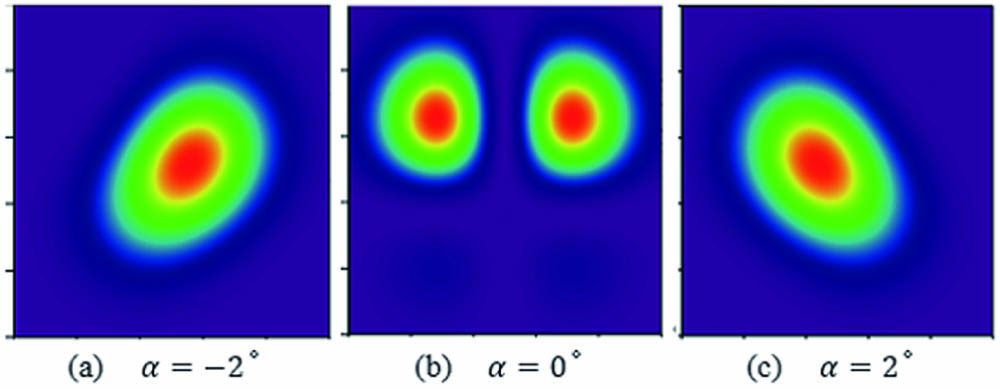
Fig. 6. (a)–(c) Theoretically simulated results of profile analyzation near the extinction position. represents the azimuth angle of the polarizer. The extinction position was defined as 0°.
According to the profile analyzation of the polarized beam above, it is proved that the laser system stably oscillated in dual-polarization eigenstates with frequency degeneracy. Apparently, the residual phase anisotropy in laser crystal resulted in the eigenfrequency splitting. Then, the introduction of loss anisotropy due to the anisotropic absorption of the SA partially offset the effect of phase anisotropy in gain media. Phase anisotropy is from intrinsic weak birefringence in gain media and thermal birefringence. So, the eigenfrequency difference was decreased to fall into the locking region. In addition, the same frequency of dual-polarization eigenmodes meant that coherent combination could occur, and final polarization states were dependent on the initial phase difference between them. Based on the fact of linear self-polarization output, it could be deduced that the phase difference was eventually stabilized at . Therefore, the polarization states strongly depend on the frequency degeneracy and phase locking, which could also reasonably explain the sensibility and instability of polarization. In other words, to stabilize the polarization states in the laser devices, some measures should be done to keep eigenfrequency differences falling in the locking region and phase difference locking strictly at . In addition, any perturbations to the locking state should be avoided.
4. Conclusions
In conclusion, through experimental and theoretical investigations, we successfully explained the mechanism of self-polarization in the monolithic laser. With frequency degeneracy and phase difference locking, the coherent combination of the two orthogonally polarized eigenmodes finally resulted in the linear polarization output. The mechanism could also be an important guideline to improve the sensibility and instability of polarization in similar laser devices. Furthermore, an effective method, profile analyzation of the polarized beam, was found to judge different types of linear polarization states: the intrinsic state or combined state.
[9] H. Liu, O. Hornia, Y. C. Chen, S.-H. Zhou. Single-frequency Q-switched Cr, Nd:YAG laser operating at 946-nm wavelength. IEEE J. Sel. Top. Quantum Electron., 1997, 3: 26.
[16] J. Dong, A. Shirakawa, K. Ueda. A crystalline-orientation self-selected linearly polarized Yb:Y3Al5O12 microchip laser. Appl. Phys. Lett., 2008, 93: 101105.
[22] G. Liu, S. Zhang, T. Xu, X. Wan. Modes competition in a birefringence cavity laser with optical feedback. Chin. Opt. Lett., 2005, 3: 694.
Kaifei Tang, Wenbin Liao, Da Lin, Bingxuan Li, Weidong Chen, Ge Zhang. Self-polarization emission based on coherent combination of intracavity eigenmodes in Nd:YAG/Cr4+:YAG lasers[J]. Chinese Optics Letters, 2021, 19(2): 021401.