Spurious-free dynamic range improvement in a photonic time-stretched analog-to-digital converter based on third-order predistortion
Download: 887次
1. INTRODUCTION
Analog-to-digital converters (ADCs), which convert continuous-time signals to digital form, are among the keys to the success of signal processing systems, including medical imaging, sonar, radar, and telecommunications [1]. However, the performance of the conventional electronic ADCs is limited by the input-referred noise, aperture jitter, and comparator ambiguity [2]. In order to overcome the bottleneck of electronic ADCs, photonic ADCs that use photonics in the digitalization process have been proposed in recent years [3]. Compared with electronic-based ADCs, the photonic method enjoys the advantages of low loss, high time-bandwidth product, and immunity to electromagnetic interference [4]. Photonic ADCs can be divided into several classes depending on whether the sampling and quantization are performed in the photonic domain. Among those, the photonic time-stretched ADC with sampling and quantization performed in the electronic domain can use photonic methods to enhance the performance of the electronic ADC. The enhancements include an increase in the sampling rate and the input bandwidth, reduction in the digitizer sampling jitter noise, and interchannel mismatch errors [5]. Meanwhile, one of the disadvantages of the photonic time-stretched ADC is that the photonic processing of the electronic signal usually requires a Mach–Zehnder modulator (MZM) to achieve electro-optic conversion. However, the transfer function of the MZM generally has nonlinear response, within which nonlinear distortions such as harmonic distortions and intermodulation distortions (IMDs) are induced into the system. These undesired components will reduce the performance of the electronic ADC, such as the reduction of the spurious-free dynamic range (SFDR), which is a usually accepted measure for the dynamic performance of an ADC [1]. To cancel the even-order harmonics and IMDs in the photonic time-stretched ADC, differential pull–push modulation was proposed in [6]. However, the amplitude and phase mismatches will weaken the effectiveness of the differential operation by reducing the distortion rejection ratio (DDR) [7]. Meanwhile, in most cases, the third-order IMD (IMD3) is the largest limitation of the SFDR since IMD3 always exists in the spectrum of interest and is difficult to filter out.
In this paper, we propose a new scheme to improve the IMD3-determined SFDR of the photonic time-stretched ADC based on the third-order predistortion. The technique of high-order predistortion has been widely used to achieve linearization in both electronics and photonics [810" target="_self" style="display: inline;">–
2. PRINCIPLE OF OPERATION
A photonic time-stretched ADC is usually composed of two parts, the photonic time-stretched preprocessor, which is used to stretch the input radio frequency (RF) signal in the time domain, and an electronic ADC, as shown in Fig.
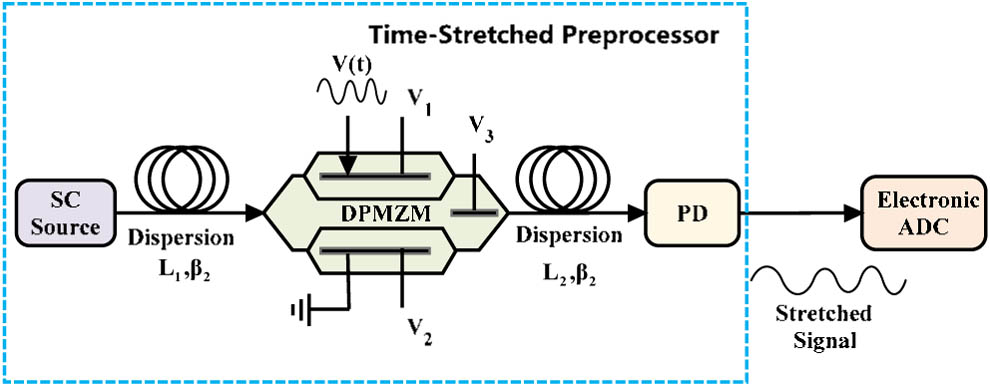
Fig. 1. Schematic illustration of the proposed photonic time-stretched ADC based on third-order predistortion (SC, super-continuum).
Assume that the optical pulse is a Gaussian-shaped pulse generated by the super-continuum optical source. In the frequency domain, the electric field of the optical pulse can be written as [11] where
Then the chirped optical pulse is sent to an X-cut integrated DPMZM, which can be seen as a combination of three sub-MZMs: an upper MZM, a lower MZM, and a main MZM. The DPMZM is used to modulate the input RF signal onto the chirped optical pulse and predistort the IMD3 component. Within the DPMZM, the RF signal is connected to the input of the upper MZM, the RF input of the lower MZM is shorted, and three sub-MZMs are biased at
Then the output of the DPMZM passes through the second dispersive medium, which has a same GVD parameter with the first dispersive medium, and the electric field in the frequency domain becomes where
Finally, the stretched optical pulse is sent to a PD to accomplish photoelectric conversion. The intensity of the current
In order to analyze the third-order IMD components, a two-tone RF signal is input to drive the DMPZM, where
By applying a Bessel expansion to Eq. (
In this case, Eq. (
Ignoring the high-order components, the IMD3 can be divided into two parts; one is the beating between the carrier and the IMD3 components generated by the modulation, and the other is the beating between fundamental and second harmonics thorough PD. So the coefficient of the IMD3 is given by where
By expanding Eq. (
In this condition, if the biases of the DPMZM satisfy the first term of the
It should be noted that a perfect third-order predistortion may enhance the even-order harmonics and third-order harmonics, which can reduce the performance of the scheme. In most cases, these harmonics are far from the spectrum of our interest, and can be easily removed by a filter. In some cases, the frequency selectivity of the photonic time-stretched preprocessor will weaken the enhancement and make these components have little influence on the improvement of SFDR. Since the transfer function of the photonic time-stretched preprocessor can be written as [5] if the frequency of the input signal is known in advance, for example, in digital communications or a radar system, the parameter of the optical link can be adjusted to suppress certain harmonics.
3. EXPERIMENTS AND RESULTS
Figure
And the photoelectric convention is achieved by a PD with a bandwidth of 880 MHz. In order to analyze the SFDR of the photonic time-stretched ADC, the stretched electrical signal is sent to a spectrum analyzer instead of an electronic ADC.
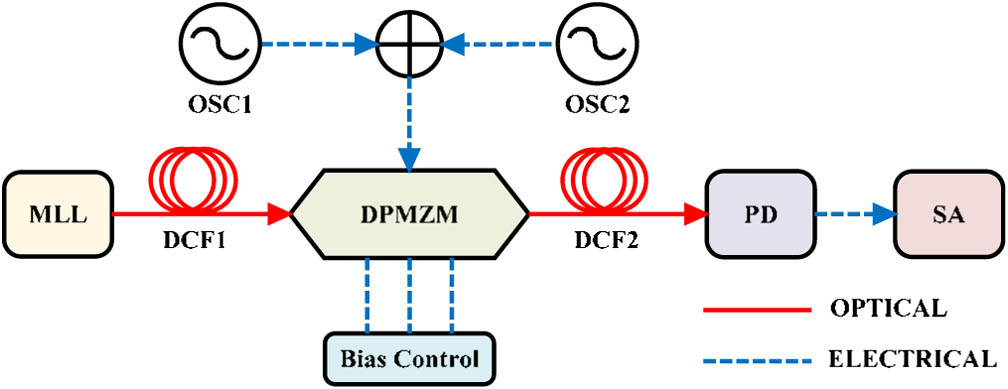
Fig. 2. Experimental setup of the proposed photonic time-stretched ADC based third-order predistortion (OSC, oscillator; SA, spectrum analyzer).
A comparison with a conventional scheme without predistortion that uses a common MZM biased at the quadrature point is performed. The RF signal input to the modulator has a power level of 20 dBm, and the optical power input to the PD is about 3 dBm. The frequency of the RF signal
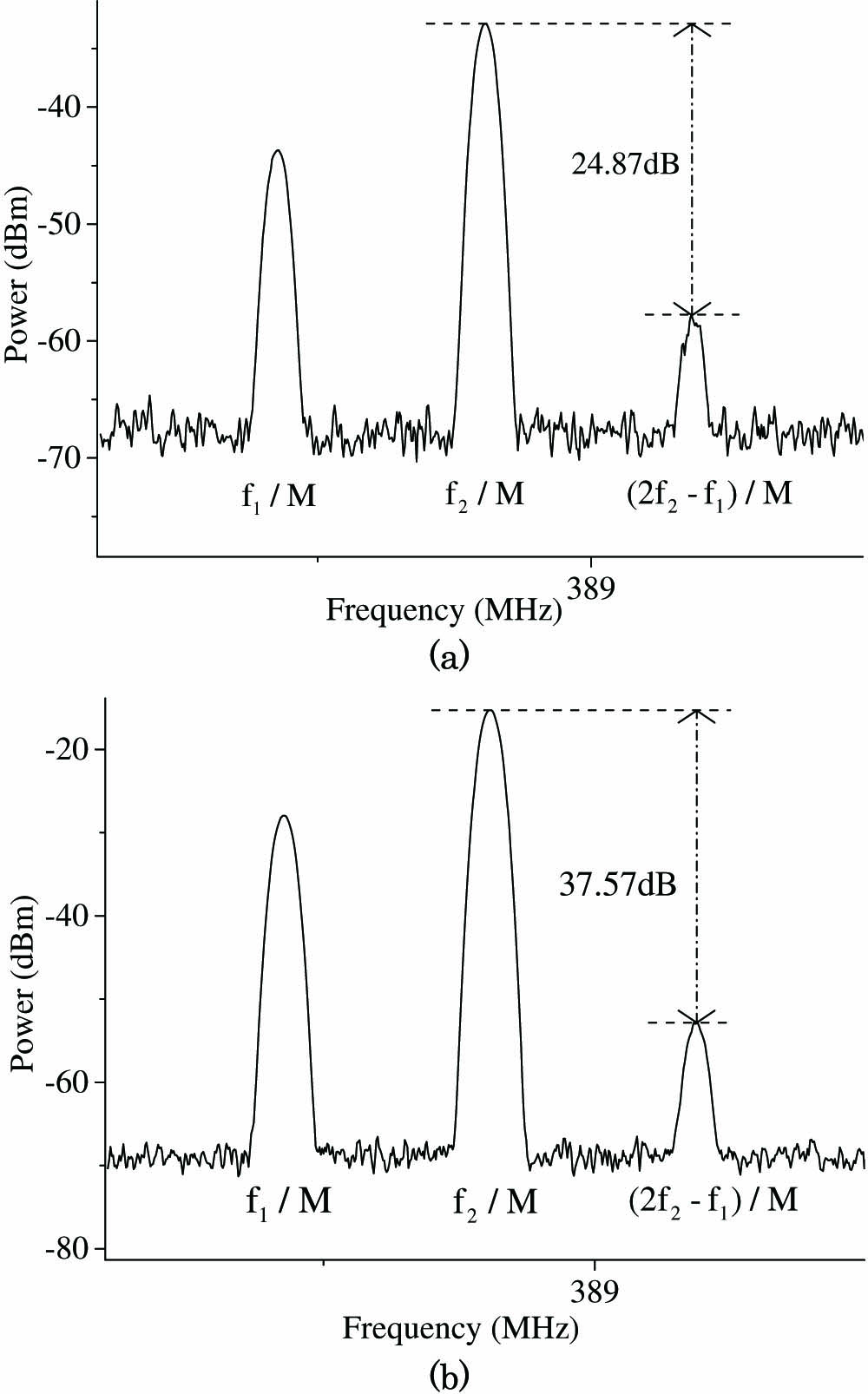
Fig. 3. (a) Electronic output spectrum for the conventional scheme without predistortion by using a common MZM. (b) Electronic output spectrum for the highly linearized scheme based on third-order predistortion by using DPMZM.
Figure
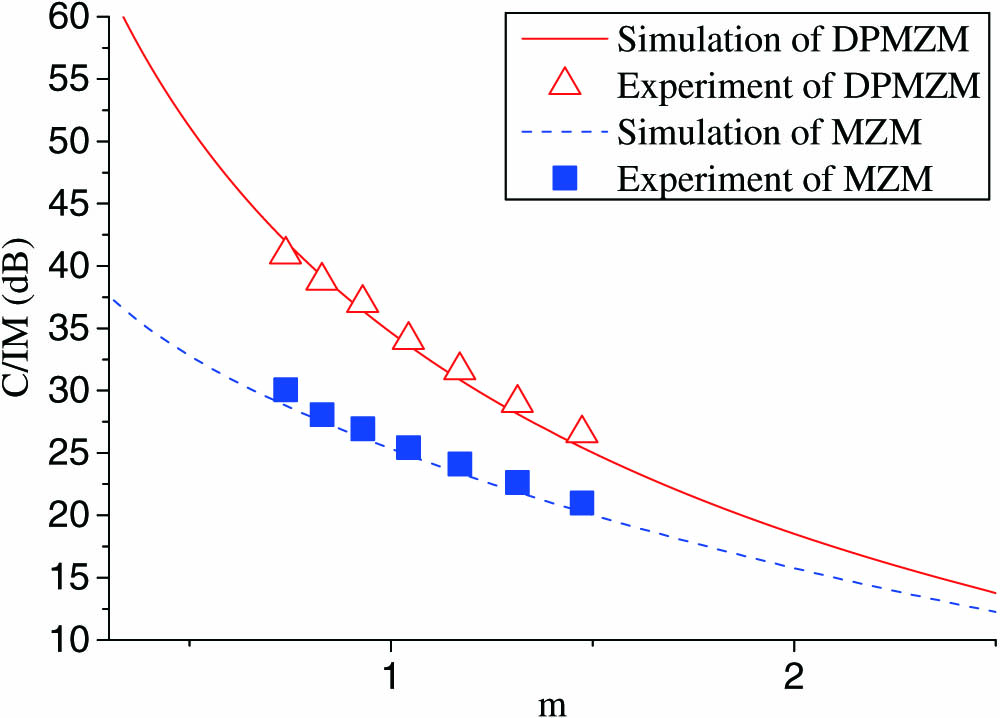
Fig. 4. Simulation and experimental results show the C/IM as a function of the modulation index m.
Figure
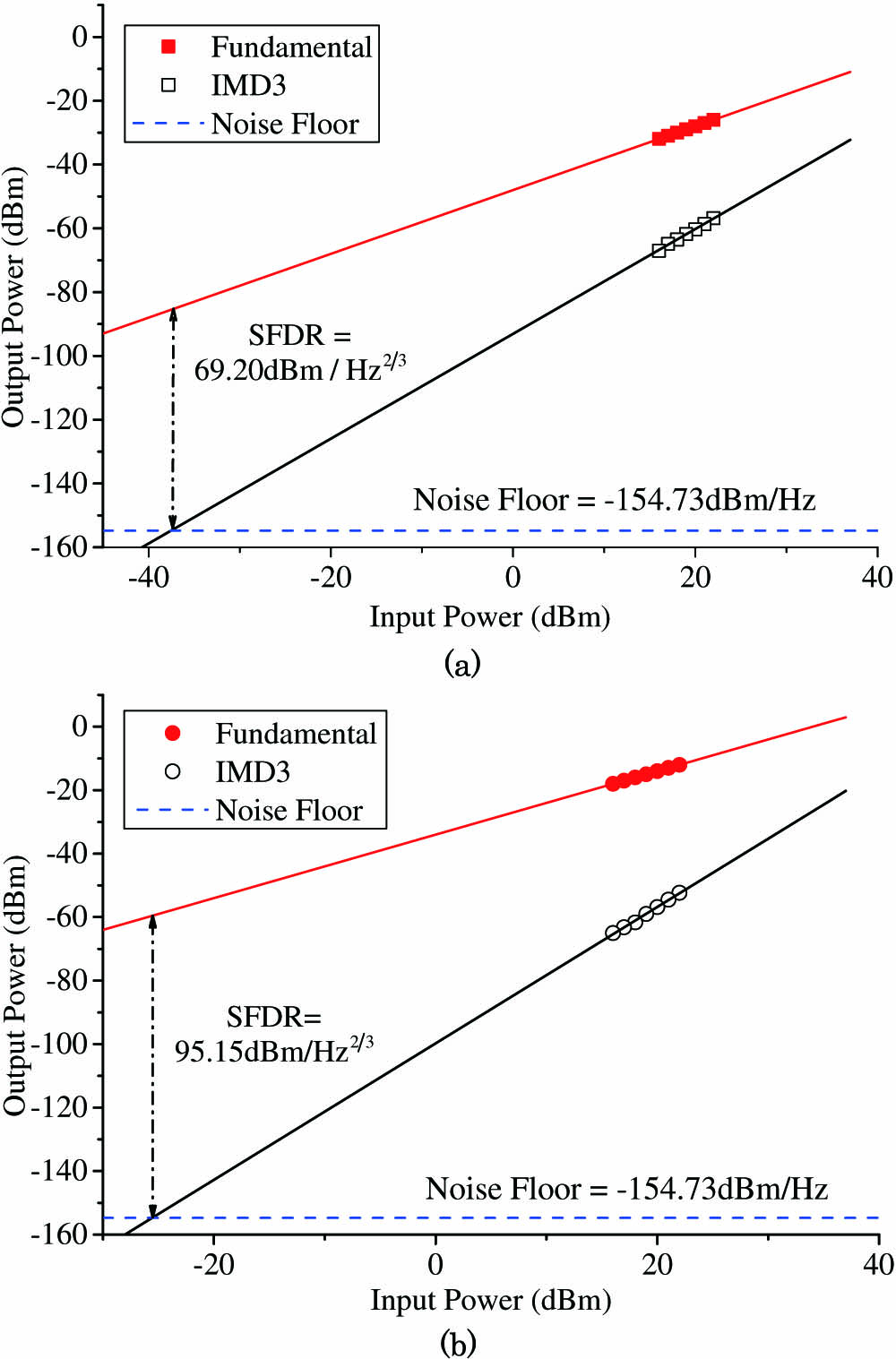
Fig. 5. Power of the stretched signal as a function of electronic input power for our proposed scheme and conventional scheme without predistortion. (a) Squares and lines represent the experimental data and linear fits to the fundamental and limiting IMD3 of common MZM scheme. (b) Circles and lines represent the experimental and linear fits to the fundamental and limiting IMD3 of the proposed DPMZM scheme.
Compared with other optical links, such as the linear radio-over-fiber (ROF) system, of which the SDFR can be above
4. CONCLUSION
A novel linearized scheme based on third-order predistortion by using an integrated DPMZM as a predistorter in a photonic time-stretched ADC is proposed in this paper. The experimental result shows that the SFDR of the proposed system is
Boyu Xu, Wulue Lv, Jiamu Ye, Jinhai Zhou, Xiaofeng Jin, Xianmin Zhang, Hao Chi, Shilie Zheng. Spurious-free dynamic range improvement in a photonic time-stretched analog-to-digital converter based on third-order predistortion[J]. Photonics Research, 2014, 2(5): 05000097.