保偏光纤陀螺横向磁场误差的温度依赖性
下载: 1160次
1 引言
光纤陀螺(FOG)是一种基于Sagnac效应的角速度传感器,通过检测在光纤环中相向传输的两束光波的非互易性相移来测量角速度。由于其具有全固态设计、启动快、重量轻、动态范围大、结构灵活等特点,被广泛应用于各种军用及民用惯性导航领域[1]。经过近四十年的发展,光纤陀螺研制技术逐步成熟,目前研究工作主要集中在小型化[2]、低成本[3]、新型结构[4]和高精度[5]等方面。为研制出高精度光纤陀螺,需要对多物理场(温度、磁场、应力等)作用下光纤陀螺的误差产生机理进行深入的研究和探索。
目前针对单一的磁、热物理场对光纤陀螺的影响已有大量报道。当磁场方向与光纤环敏感轴垂直时,保偏光纤因扭转与法拉第效应引起的圆双折射使得光波由线偏振态演变为椭圆偏振态,进而导致横向磁场误差产生[6]。横向磁场误差与光纤的线双折射、扭转、弯曲、Verdet常数、长度等参数相关。常见的横向磁场误差抑制方案有:在光纤环外添加磁屏蔽罩[7],将常用的保偏光纤替换为光子带隙光纤[8],添加补偿光纤环[9],软件补偿[10],以及光学补偿[11-13]等。另一方面,当环境温度发生变化时,保偏光纤的折射率会发生变化。由于光纤环中的Shupe效应以及热应力效应,光纤陀螺会产生温度漂移[14-17]。
在实际应用中,磁场与温度场对光纤陀螺的影响是同时存在的。研究显示,在-20~60 ℃温度范围内,由于光纤环骨架与光纤材料的热膨胀系数不同,热应力导致保偏光纤的线双折射变化值可达到500 rad·m-1,进而导致光纤陀螺的横向磁场误差随温度变化而变化[18]。当磁、热物理场同时存在时,带骨架光纤陀螺的横向磁场误差存在温度依赖性。
实际上,为了减小光纤环与光纤环骨架之间的热应力,当光纤环绕制成型后可以取出骨架而制得无骨架光纤环。研究无骨架光纤环中横向磁场误差与温度的关系对提高光纤陀螺的环境适应性具有一定的理论指导意义。本文利用琼斯矩阵的方法建立了无骨架光纤陀螺的横向磁场误差与温度的关系模型,并实验验证了该模型的合理性。研究表明,无骨架光纤陀螺的横向磁场误差会随着温度的不同而发生变化,产生这种现象的原因是保偏光纤的线双折射和Verdet常数固有的温度依赖性[19-21]。
2 理论与仿真
保偏光纤陀螺系统结构如
光纤环是光纤陀螺中产生磁场误差以及温度漂移的主要误差源。如

图 2. (a)空间磁场作用下的光纤环;(b)横向磁场分解
Fig. 2. (a) Fiber coil under the effect of space magnetic field; (b) decomposition of transverse magnetic field
如
式中
同样,第
式中

图 3. 横向磁场和温度场作用下的光纤环
Fig. 3. Fiber coil under the action of transverse magnetic field and temperature field
从端口0#入射的光波沿着光纤环传播一圈后再从端口0#射出。整个光路中顺时针光波的电场分量为
式中
对应的横向磁场误差
式中
当温度场作用在光纤环时,熊猫型光纤的纤芯材料性能和纤芯区所受的应力会随着温度变化,进而导致光纤双折射Δ
式中Δ
此外,光纤的Verdet常数与温度的关系可表示为[20]
式中
光纤的线双折射和Verdet常数的温度依赖性如
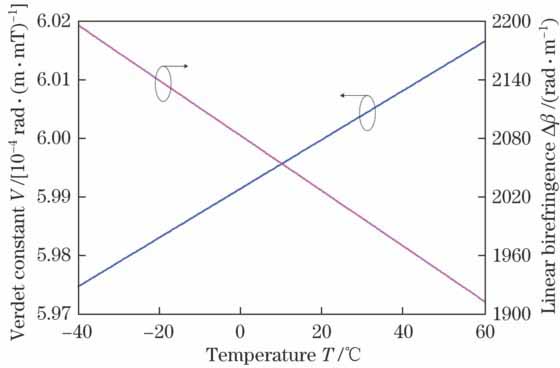
图 4. Verdet常数与线双折射的温度依赖性
Fig. 4. Temperature dependence of Verdet constant and linear birefringence
将(8)式和(9)式代入(7)式可知,光纤陀螺的横向磁场误差也会随着温度变化,而且线双折射的温度依赖性对这种现象的产生起主导作用。利用
对于波长
场误差与温度的关系如

图 5. 横向磁场误差与温度的关系
Fig. 5. Relationship between the transverse magnetic field error and the temperature
3 实验结果与分析
为了验证上述理论分析的合理性,依据
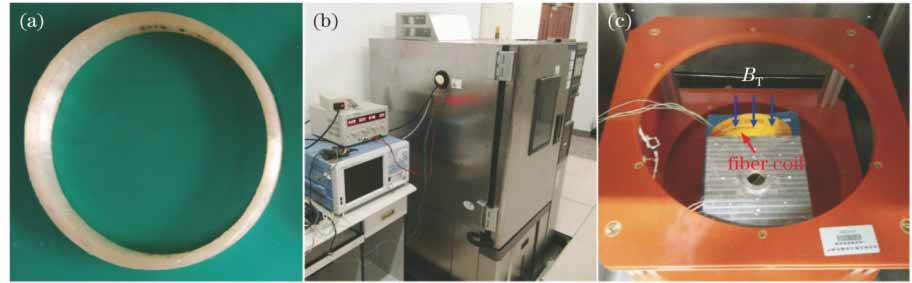
图 6. 实验系统图。(a)无骨架光纤环;(b)温箱;(c) Helmholtz线圈与光纤环
Fig. 6. Experimental system. (a) Non-skeleton coil; (b) temperature chamber; (c) Helmholtz coil and fiber coil
如
为了消除Helmholtz线圈的温度依赖性,一个带温度补偿的磁场传感器被用来实时检测光纤环周围的磁场强度。在1 mT横向磁场作用下,光纤陀螺的横向磁场误差与温度的关系如
由于上述实验需要在不同温度点进行,温度变化引起的温度漂移可能会对实验结果产生影响。实验中采用四极子对称绕法制作光纤环,并且在恒定的温度点测量横向磁场误差(温度变化速率近似为0 ℃·min-1),因此Shupe效应引起的温度漂移可以忽略。此外,

图 8. 不同温度点下的横向磁场误差实验结果
Fig. 8. Experimental results of transverse magnetic field error under different temperatures
引起的温度漂移不会影响到横向磁场误差。综上所述,经过合理的实验设计,温度漂移不会影响到
为了验证温度漂移对
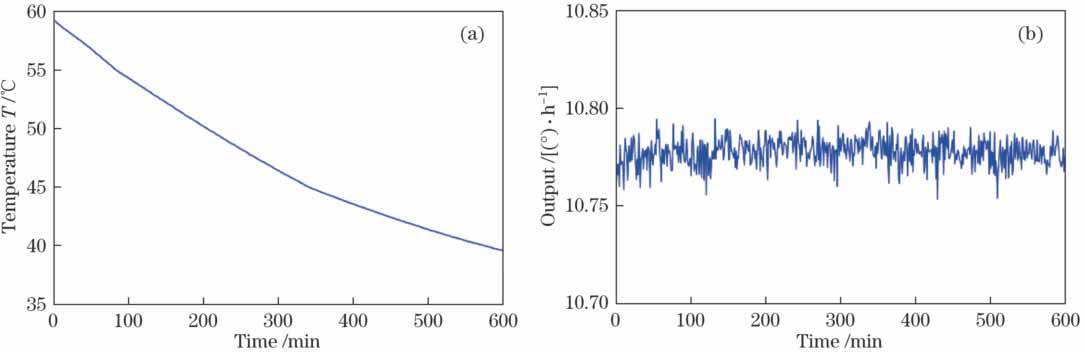
图 9. 温度漂移实验结果。(a)温度输出;(b)光纤陀螺输出
Fig. 9. Experimental results of thermal-induced bias drift. (a) Temperature output; (b) output of the polarization maintaining FOG
由以上的分析可知,采用无骨架光纤环的保偏光纤陀螺的横向磁场误差存在温度依赖性。文献[ 18]中指出横向磁场误差的温度依赖性产生原因是光纤环与光纤环骨架之间的热应力产生的线双折射会随着温度发生变化,本次实验发现保偏光纤的线双折射和Verdet常数固有的温度依赖性也能导致横向磁场误差的温度依赖性。此外,与无骨架光纤环相比,带骨架光纤环的线双折射对温度变化更加敏感。
4 结论
分析与研究了无骨架保偏光纤陀螺横向磁场误差的温度依赖性。由于保偏光纤线双折射和Verdet常数固有的温度依赖性,当环境温度发生变化时,光纤陀螺的横向磁场误差会随着温度变化而变化。在考虑横向磁场误差的抑制措施时,如软件补偿和添加补偿光纤环等,也需要考虑到温度对横向磁场误差的影响,避免补偿措施的失效。另外,在进行温度漂移算法补偿时,尽量减小磁场误差的影响,提高补偿效率。后期将探索一种有效的横向磁场误差抑制措施,使光纤陀螺在温度变化的情况下仍然能够高效地抑制横向磁场误差。
[1] 张桂才. 光纤陀螺原理与技术[M]. 北京: 国防工业出版社, 2008: 1- 4.
张桂才. 光纤陀螺原理与技术[M]. 北京: 国防工业出版社, 2008: 1- 4.
张桂才. 光纤陀螺原理与技术[M]. 北京: 国防工业出版社, 2008: 1- 4.
Zhang GC. The principles and technologies of fiber-optic gyroscope[M]. Beijing: National Defence Industry Press, 2008: 1- 4.
Zhang GC. The principles and technologies of fiber-optic gyroscope[M]. Beijing: National Defence Industry Press, 2008: 1- 4.
Zhang GC. The principles and technologies of fiber-optic gyroscope[M]. Beijing: National Defence Industry Press, 2008: 1- 4.
[2] 王立辉, 徐晓苏, 刘锡祥, 等. 小型化光纤陀螺的轴向磁场误差特性建模方法探讨[J]. 中国惯性技术学报, 2012, 20(1): 84-89.
王立辉, 徐晓苏, 刘锡祥, 等. 小型化光纤陀螺的轴向磁场误差特性建模方法探讨[J]. 中国惯性技术学报, 2012, 20(1): 84-89.
王立辉, 徐晓苏, 刘锡祥, 等. 小型化光纤陀螺的轴向磁场误差特性建模方法探讨[J]. 中国惯性技术学报, 2012, 20(1): 84-89.
Wang L H, Xu X S, Liu X X, et al. Investigation on modeling methods of axial magnetic field error characteristics in small fiber optic gyroscope[J]. Journal of Chinese Inertial Technology, 2012, 20(1): 84-89.
[3] Sanders GA, Sanders SJ, Strandjord LK, et al. Fiber optic gyroscope development at Honeywell[C]. International Society for Optics and Photonics, 2016: 985207.
Sanders GA, Sanders SJ, Strandjord LK, et al. Fiber optic gyroscope development at Honeywell[C]. International Society for Optics and Photonics, 2016: 985207.
Sanders GA, Sanders SJ, Strandjord LK, et al. Fiber optic gyroscope development at Honeywell[C]. International Society for Optics and Photonics, 2016: 985207.
[4] 徐宏杰, 张文艳, 徐小斌, 等. 双光程光纤陀螺偏振误差模型与仿真[J]. 光学学报, 2014, 34(10): 1006002.
徐宏杰, 张文艳, 徐小斌, 等. 双光程光纤陀螺偏振误差模型与仿真[J]. 光学学报, 2014, 34(10): 1006002.
徐宏杰, 张文艳, 徐小斌, 等. 双光程光纤陀螺偏振误差模型与仿真[J]. 光学学报, 2014, 34(10): 1006002.
Xu H J, Zhang W Y, Xu X B, et al. Polarization bias error model and simulation of fiber-optic gyroscope with double optical length[J]. Acta Optic Sinica, 2014, 34(10): 1006002.
[7] 李金涛, 房建成. 高精度光纤 IMU 的磁屏蔽方法及实验研究[J]. 航空学报, 2011, 32(11): 2106-2116.
李金涛, 房建成. 高精度光纤 IMU 的磁屏蔽方法及实验研究[J]. 航空学报, 2011, 32(11): 2106-2116.
李金涛, 房建成. 高精度光纤 IMU 的磁屏蔽方法及实验研究[J]. 航空学报, 2011, 32(11): 2106-2116.
Li J T, Fang J C. Magnetic shielding method and experiment study of inertial measurement unit based on high precision fiber-optic gyroscope[J]. Acta Aeronautica et Astronautica Sinica, 2011, 32(11): 2106-2116.
[9] 刘军, 肖程, 潘欣, 等. 抑制光纤陀螺径向磁敏感性研究[J]. 中国激光, 2015, 42(3): 0305005.
刘军, 肖程, 潘欣, 等. 抑制光纤陀螺径向磁敏感性研究[J]. 中国激光, 2015, 42(3): 0305005.
刘军, 肖程, 潘欣, 等. 抑制光纤陀螺径向磁敏感性研究[J]. 中国激光, 2015, 42(3): 0305005.
[11] 胡宗福, 姜润知, 周剑. 保偏光纤干涉型陀螺的磁场误差分析与抑制方法[J]. 光学学报, 2014, 34(6): 0606003.
胡宗福, 姜润知, 周剑. 保偏光纤干涉型陀螺的磁场误差分析与抑制方法[J]. 光学学报, 2014, 34(6): 0606003.
胡宗福, 姜润知, 周剑. 保偏光纤干涉型陀螺的磁场误差分析与抑制方法[J]. 光学学报, 2014, 34(6): 0606003.
[14] 宋凝芳, 关月明, 贾明. 光纤陀螺光纤环 Shupe 误差的多参数影响仿真分析[J]. 北京航空航天大学学报, 2011, 37(5): 569-573.
宋凝芳, 关月明, 贾明. 光纤陀螺光纤环 Shupe 误差的多参数影响仿真分析[J]. 北京航空航天大学学报, 2011, 37(5): 569-573.
宋凝芳, 关月明, 贾明. 光纤陀螺光纤环 Shupe 误差的多参数影响仿真分析[J]. 北京航空航天大学学报, 2011, 37(5): 569-573.
Song N F, Guan Y M, Jia M. Analysis of multi-parameters effect on Shupe error in fiber optic gyroscope fiber coil[J]. Journal of Beijing University of Aeronautics and Astronautics, 2011, 31(5): 569-573.
[15] 李家垒, 许化龙, 何靖. 基于小波网络的光纤陀螺启动漂移温度补偿[J]. 光学学报, 2011, 31(5): 0506005.
李家垒, 许化龙, 何靖. 基于小波网络的光纤陀螺启动漂移温度补偿[J]. 光学学报, 2011, 31(5): 0506005.
李家垒, 许化龙, 何靖. 基于小波网络的光纤陀螺启动漂移温度补偿[J]. 光学学报, 2011, 31(5): 0506005.
[16] 刘元元, 杨功流, 李思宜. BP-AdaBoost模型在光纤陀螺零偏温度补偿中的应用[J]. 北京航空航天大学学报, 2014, 40(2): 235-239.
刘元元, 杨功流, 李思宜. BP-AdaBoost模型在光纤陀螺零偏温度补偿中的应用[J]. 北京航空航天大学学报, 2014, 40(2): 235-239.
刘元元, 杨功流, 李思宜. BP-AdaBoost模型在光纤陀螺零偏温度补偿中的应用[J]. 北京航空航天大学学报, 2014, 40(2): 235-239.
Liu Y Y, Yang G L, Li S Y. Application of BP-AdaBoost model in temperature compensation for fiber optic gyroscope bias[J]. Journal of Beijing University of Aeronautics and Astronautics, 2014, 40(2): 235-239.
[17] 李绪友, 凌卫伟, 许振龙, 等. 基于交叉法绕制的光纤环的槽体设计[J]. 光学学报, 2015, 35(6): 0606002.
李绪友, 凌卫伟, 许振龙, 等. 基于交叉法绕制的光纤环的槽体设计[J]. 光学学报, 2015, 35(6): 0606002.
李绪友, 凌卫伟, 许振龙, 等. 基于交叉法绕制的光纤环的槽体设计[J]. 光学学报, 2015, 35(6): 0606002.
李绪友, 刘攀, 光星星, 郭衍达, 孟庆文, 李光春. 保偏光纤陀螺横向磁场误差的温度依赖性[J]. 光学学报, 2018, 38(1): 0106006. Xuyou Li, Pan Liu, Xingxing Guang, Yanda Guo, Qingwen Meng, Guangchun Li. Temperature Dependence of Transverse Magnetic Error in a Polarization Maintaining Fiber Optic Gyroscope[J]. Acta Optica Sinica, 2018, 38(1): 0106006.