Subwavelength metal structures with tunable transmission characteristics by light
Download: 635次
In 1998, Ebbesen et al. reported extraordinary optical transmission (EOT) in the subwavelength metal structures with periodic hole arrays[1,2] for the first time. Since then, extensive research on the transmission in subwavelength structures has been carried out[3
Although the physical mechanism of EOT is not completely clear, it has been recognized that the shape and size of the hole, the period of the groove, the material of the film, and the refractive index of the environment have a significant effect on the transmission characteristics. Once a structure and the parameters of the structure are proposed, the transmission characteristics of the structure are fixed. It is impossible to achieve dynamic regulation of their transmission continuously. People have to etch a variety of samples with different parameters for different requirements.
In this Letter, we propose a strategy to adjust the transmission of a subwavelength metal structure by employing external light. Because the bullseye structure[24] is a typical subwavelength structure and it has been widely applied in many fields[16,17] such as selective lens and novel sensor, we use it as an example to verify the validity of this solution.
As shown in Fig.
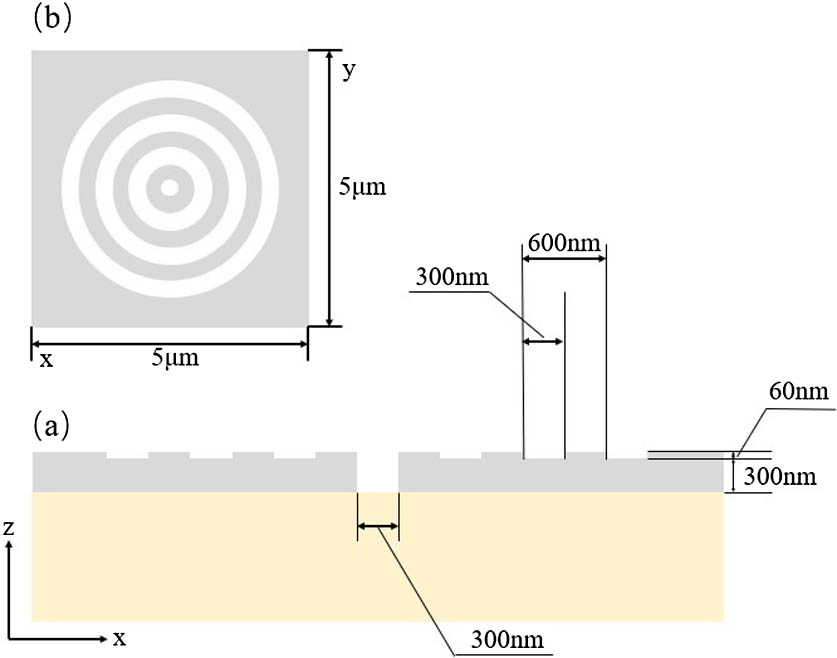
Fig. 1. Cross section of the bullseye structure (a) x-z cross section (elevation view) and (b) x-y cross section (top view).
As shown in Fig.
Using the finite-difference time-domain (FDTD) method, we simulate the transmission of the structure for a signal light (from 600 nm to 1300 nm) at five different controlling lights with power densities of
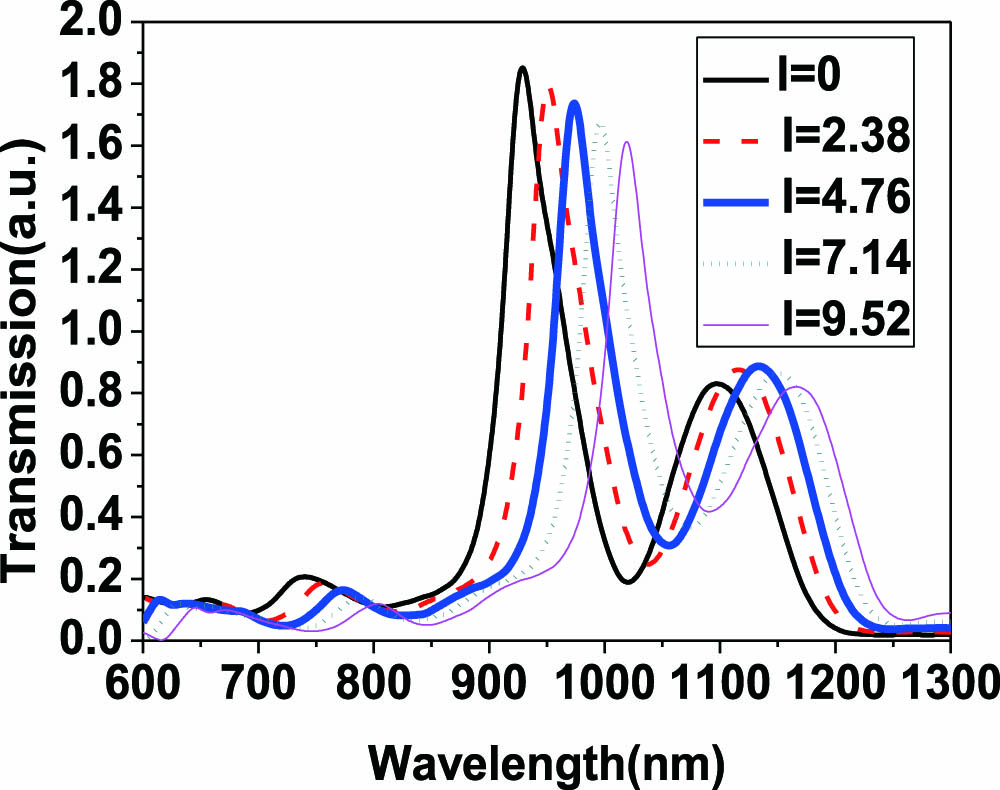
Fig. 3. Transmission spectra of the signal light under different powers of controlling light.
In Fig.

Fig. 4. Wavelength of the primary transmission peak under different powers of controlling light.
From Fig.
Generally, the mechanism of the EOT phenomenon can be considered as the coupling of surface plasmon resonance (SPR) and local surface plasmon (LSP)[6]. The incident light generates the diffraction wave in the subwavelength structure that satisfies the phase condition, thus excites SPR and enhances transmission at a specific resonance wavelength[24" target="_self" style="display: inline;">–
In addition, multiple peaks in the transmission spectrum can be considered as the dominating effect of surface plasmon and local surface plasmon, respectively. The transmission spectra of both modes are nonlinearly related to the permeability of the medium[5]. Therefore, when the third-order magnetic permeability of carbon disulfide was changed by the controlling light, the transmission peaks dominated by the two resonance modes exhibited different transmittances. So, the height of peaks in the transmission spectrum varied irregularly with the power of the controlling light.
As discussed above, plane light can realize a tuneable device. However, in the view of application, the laser is the best choice. Usually, the transverse mode of the laser pulses was nearly Gaussian. Hence, we further simulated the transmission of the structure under the laser as the controlling light. In this case, the nonlinear refractive index of
By using FDTD, we simulated the transmission spectra under the Gaussian controlling light with the center power density of
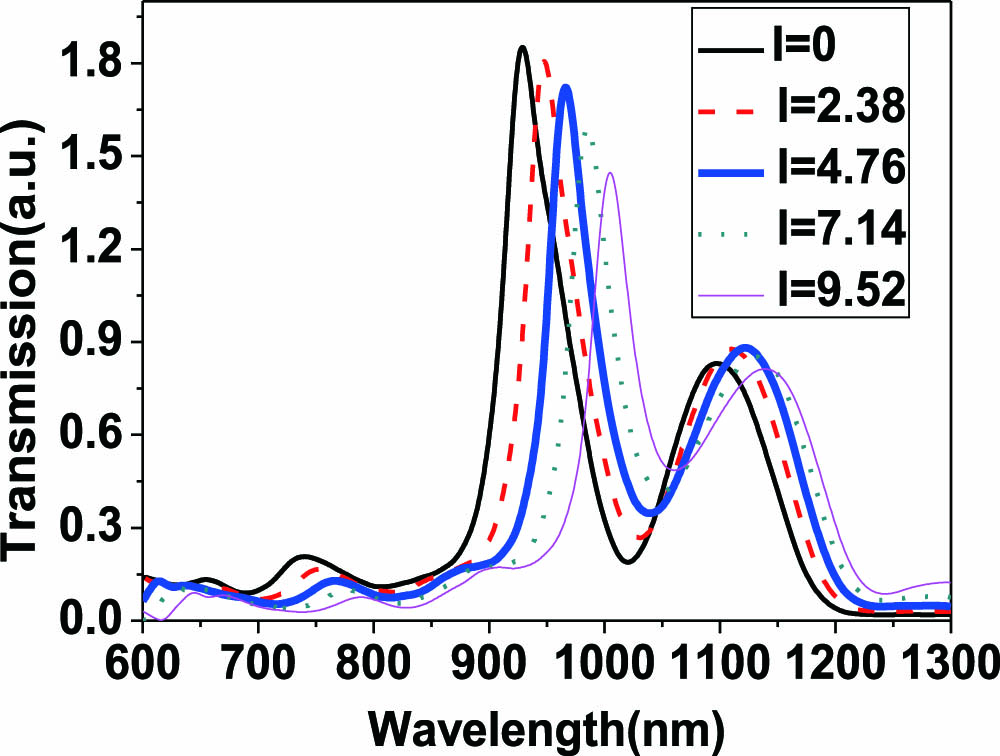
Fig. 6. Transmission spectra of the signal light under different powers of controlling light.
In Fig.
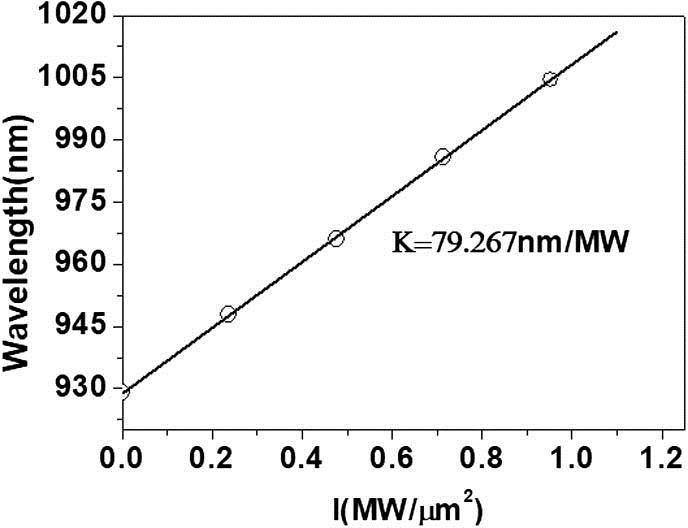
Fig. 7. Wavelengths of the primary transmission peak under different powers of controlling light.
To summarize, a strategy to adjust the transmission of a subwavelength metal structure by employing external light was proposed. Specifically, we immersed the bullseye structure in a nonlinear medium and employed a controlling light to change the refractive index of the medium to adjust the wavelength of the transmission peak of the structure. By using FDTD, we verify the feasibility of our scheme. It is necessary for us to carry out experimental studies of the design in the future. In fact, we think the strategy can also be used in other structures. Furthermore, we infer that other forms of external fields such as electric fields, magnetic fields, and thermal fields may be used to adjust the transmission characteristics of subwavelength metal structures effectively via appropriate design. More research can be conducted.
[1]
[2]
[3]
[4]
[5]
[6]
[7]
[8]
[9]
[10]
[11]
[12]
[13]
[14]
[15]
[17]
[18]
[19]
[20]
[21]
[22]
[23]
[24]
[25]
[26]
[27]
Zehua Yang, Jing Han, Wenzhi Wu, Degui Kong, Yachen Gao. Subwavelength metal structures with tunable transmission characteristics by light[J]. Chinese Optics Letters, 2019, 17(12): 122302.