风控热晕下椭圆激光光束质量的优化研究
下载: 776次
In practice, the beam produced by a high-energy laser is more nearly elliptical in its cross section, such as a semiconductor laser and a spectral beam combining system. When a high-energy laser propagates in the atmosphere, a fraction of the laser power is absorbed by the atmosphere along the propagation path. The absorbed power first heats the air and alters the refraction index of the path, and then causes laser distortion and divergence. This self-induced effect is called thermal blooming. Moreover, the energy transmission efficiency of a high-energy laser is severely limited by the thermal blooming effect. Thermal blooming depends on the distribution of the laser beam and the state of the atmosphere. Until now, the effect of thermal blooming on an elliptical Gaussian beam (EGB) propagating in the atmosphere has not been reported. In this paper, the beam quality optimization of an EGB under wind-dominated thermal blooming is studied analytically and numerically. The results obtained in this paper are useful for the applications of high-energy lasers propagating in the atmosphere.
Thermal blooming of a laser beam propagating through atmosphere can be described by the paraxial wave equation and the hydrodynamic equation. When the diffraction effect is neglected under the geometric optics approach, the intensity expression for steady-state thermal blooming and the distortion parameter of an EGB propagating in the atmosphere are respectively derived, and their correctness is proved. Besides, numerical simulation is very useful to study thermal blooming. We design 4D computer code of the time-dependent atmospheric propagation of a focused EGB by using the multi-phase screen method and the finite difference method. A grid size of 512×512 is used. The numerical calculation results remain almost constant when the number of grid size increases. The transient-state thermal blooming and steady-state thermal blooming are investigated in this paper.
In this paper, the intensity expressions for steady-state thermal blooming and the distortion parameter of an EGB propagating in the atmosphere are derived, respectively. It is proved that the distortion parameter (Eq. (4)) we obtained is valid. In the atmosphere, as the beam width in the windward direction of the source plane is large (the same spot area), the distortion parameter is small and the thermal blooming effect on the EGB is weak (Figs.1 and 2). The relative peak intensity of an EGB and its displacement at the target are discussed. As the beam width in the windward direction of the source plane is large, the relative peak intensity at the target is large, and its displacement is small (Fig. 3). The 4D computer code of the time-dependent atmospheric propagation of a focused EGB is designed. It is found that in free space, the long and short axes of an EGB are interchanged at the target. In particular, in free space, the focused EGBs with the same spot areas return to the symmetric Gaussian beam at a certain propagation distance, whose formula is derived (Eq. (13)). However, in the atmosphere, different EGBs do not become symmetrical at a certain propagation distance because of thermal blooming (Fig. 5). Due to the astigmatism of an EGB, the influence of atmospheric thermal blooming on its propagation depends on the wind direction. The thermal blooming can be weakened by making the short axis of a focused EGB along the general wind direction. As the beam width in the windward direction of the source plane is large (the same spot area), the thermal blooming effect on a focused EGB is weak, which results in a better symmetrical spot (Fig. 6) and a small focal shift (Fig. 8). The time required to achieve the steady-state thermal blooming for a focused EGB is proportional to the beam width along the wind direction in the source plane (Fig.10).
In this paper, the beam quality optimization of an elliptical Gaussian beam under wind-dominated thermal blooming is studied analytically and numerically. The expression of distortion parameter of an elliptical Gaussian beam propagating in the atmosphere is derived, and its correctness is proved. Due to the astigmatism of an elliptical Gaussian beam, the influence of atmospheric thermal blooming on its propagation depends on the wind direction. Thermal blooming can be weakened by making the short axis of a focused EGB along the general wind direction. In the atmosphere, as the beam width in the windward direction of the source plane is large (the same spot area), the thermal blooming effect on the EGB is weak, which results in a better symmetrical spot and energy focus ability at the target, i.e. better beam quality at the target. The time required to achieve steady-state thermal blooming for a focused elliptical Gaussian beam is proportional to the beam width along the wind direction in the source plane.
1 引言
高能激光在大气中传输时会遭遇非线性热晕效应。热晕效应会使激光畸变和发散,从而严重限制了高能激光的能量传输效率[1-4]。因此,人们对此进行了广泛的研究。在实验方面,Smith [5]研究了激光束的稳态热散焦效应以及湍流热晕的综合效应[6]。在理论解析方面,Gebhardt等[7]给出了激光束大气近场传输的稳态热晕光强解析公式,该公式可描述热晕的基本特征。文献[8]运用菲涅耳-基尔霍夫近似和格林函数法得到了热晕方程的积分表达式。数值模拟是研究热晕效应非常有效的一种方法[9-16]。文献[12]使用广义热畸变参数,较好地定标了自适应光学系统对聚焦光束热晕效应的相位补偿效果。Vorob’Ev 等[13]研究了环状光束在移动介质中传输时的热晕效应。最近,文献[14]报道了涡旋光束在大气中传输时的稳态热晕效应,指出在光束近场传播中,采用多层相位屏法与采用一阶扰动法获得的结果基本相同。本课题组用数值模拟方法研究了热晕效应对艾里光束[15]、平顶光束[16]及厄米高斯光束[11]等传输的影响,发现对于不同光强分布激光的传输,热晕效应的影响不同,且差异显著。
实际高能激光器出射的激光束存在椭圆分布。例如,半导体激光器快慢轴的非对称性导致出射的激光束存在椭圆分布[17];在光谱合束系统中,由于激光热效应致光栅畸变和各子束存在线宽,出射的激光束存在椭圆分布[18-19]。关于椭圆高斯光束在自由空间和湍流等线性介质中的传输研究已有报道[20-24]。吴逢铁课题组分析了椭圆高斯光束经轴棱锥后的近轴光场分布特性[20]。Zheng[21]研究了椭圆高斯光束通过带孔傍轴光学系统的传输特性。另一方面,椭圆高斯光束在非线性介质中的传输特性研究也有少量报道[25-30]。陈国柱等[26]利用椭圆高斯光束产生了266 nm紫外连续激光,并且指出椭圆高斯光束可以有效改善走离效应,提高倍频转化效率。Cornolti等[29]发现椭圆高斯光束在Kerr介质中的自陷阈值随着椭圆率的增加而增加。本课题组研究了聚焦像散椭圆高斯光束在Kerr非线性介质中的传输特性,发现利用光束像散可以调控光束焦点个数[30]。然而,大气非线性热晕效应对椭圆高斯光束传输特性的影响鲜有报道。
本文推导了椭圆高斯光束在大气热晕中的光强以及热畸变扭曲参数的解析公式。利用自编的激光大气四维仿真程序,详细讨论了具有不同像散程度的聚焦椭圆高斯光束的大气热晕效应的强弱以及热晕效应对其光束质量和时间尺度的影响情况,并给出了合理的物理解释。
2 稳态热晕下椭圆高斯光束的光强及其扭曲参数解析式
在直角坐标系(x,y,z)下椭圆高斯光束的光场强度定义[29]为
在几何光学近似下,激光束在沿x正轴方向的风速为
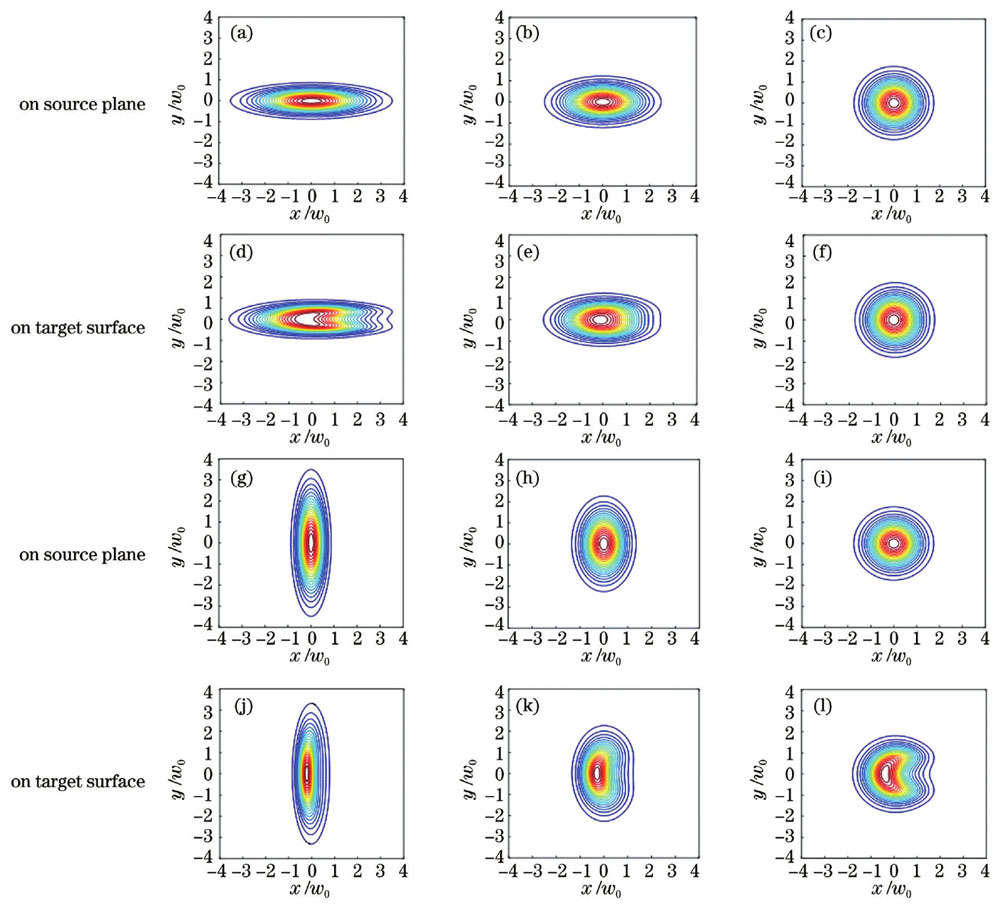
图 2. 椭圆高斯光束的光强分布。(a)β0=0.25,z=0,P=25 kW;(b)β0=0.49,z=0,P=25 kW;(c)β0=1.00,z=0,P=25 kW;(d)N=0.3025,z=2 km,P=25 kW;(e)N=0.1102,z=2 km,P=25 kW;(f)N=0.0378,z=2 km,P=25 kW;(g)β0=4.00,z=0,P=250 kW;(h)β0=1.69,z=0,P=250 kW;(i)β0=1.00,z=0,P=250 kW;(j)N=0.0473,z=2 km,P=250 kW;(k)N=0.1721,z=2 km,P=250 kW;(l)N=0.3781,z=2 km,P=250 kW
Fig. 2. Intensity distributions of elliptical Gaussian beam. (a) β0=0.25,z=0,P=25 kW; (b) β0=0.49,z=0,P=25 kW; (c) β0=1.00,z=0,P=25 kW; (d) N=0.3025, z=2 km, P=25 kW; (e) N=0.1102,z=2 km,P=25 kW; (f) N=0.0378,z=2 km, P=25 kW; (g) β0=4.00,z=0,P=250 kW; (h) β0=1.69,z=0,P=250 kW; (i) β0=1.00, z=0, P=250 kW; (j) N=0.0473,z=2 km,P=250 kW; (k) N=0.1721,z=2 km,P=250 kW; (l) N=0.3781,z=2 km,P=250 kW
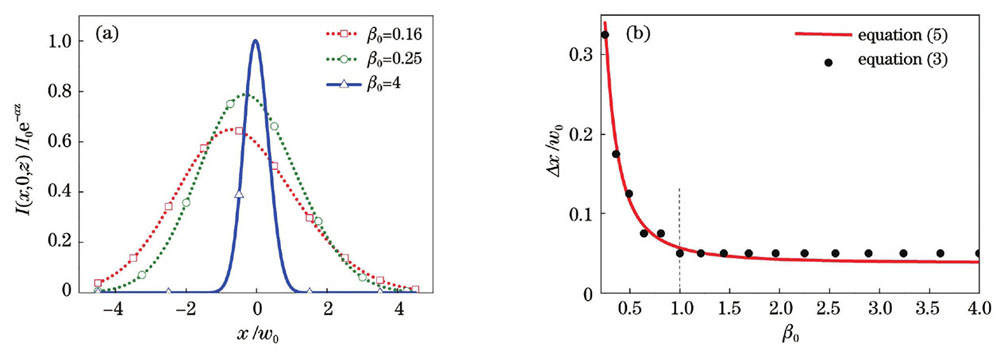
图 3. 靶面归一化光强分布以及峰值光强的位移。(a) 沿风向的归一化光强分布;(b)峰值光强的位移随β0的变化,P=25 kW
Fig. 3. Normalized intensity profiles at target and displacement of peak intensity. (a) Normalized intensity profiles in wind direction; (b) displacement of peak intensity versus β0 at P=25 kW
当β0=1时,(5) 式简化为文献[7]中的公式。
值得指出的是,以上解析方法仅适用于准直激光束达到稳态热晕并且热晕效应较小的情况,而数值模拟方法无此局限性。
3 聚焦椭圆高斯光束热晕效应的模拟仿真
傍轴近似下麦克斯韦波动方程表示[10]为
3.2 不同位置处的光强分布
由于椭圆高斯光束的像散特性,风向对热晕效应的强弱有着显著的影响。
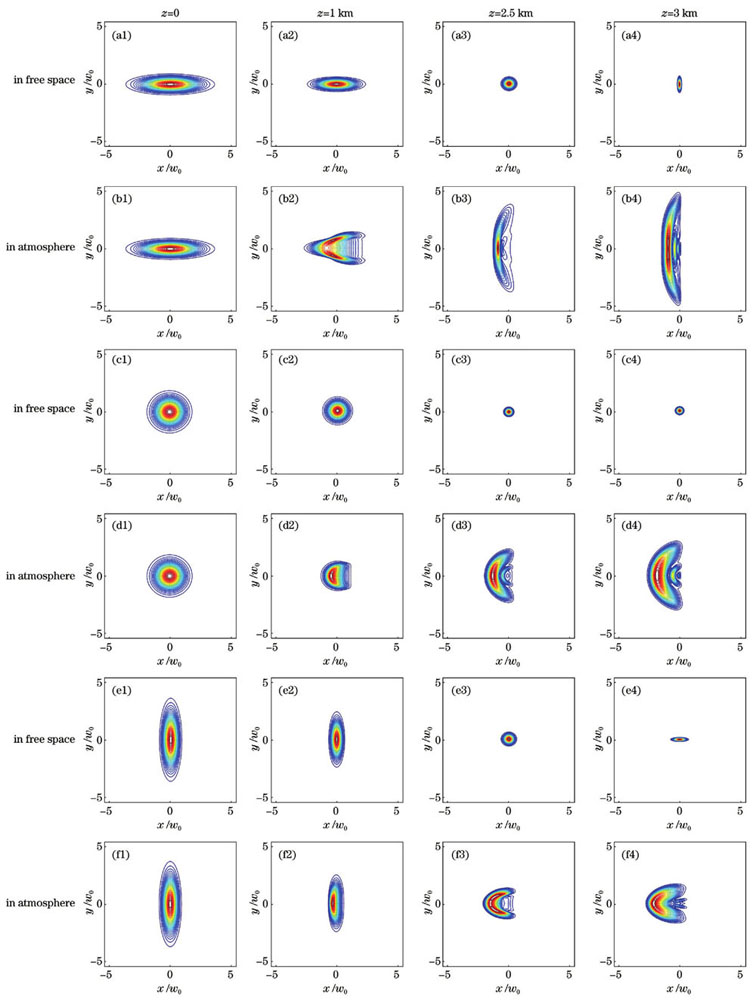
图 4. 聚焦椭圆高斯光束在不同传输距离处的光斑图。(a1)~(b4)β0=0.25;(c1)~(d4)β0=1.00;(e1)~(f4)β0=4.00
Fig. 4. Spots of focused elliptical Gaussian beam at different propagation distances. (a1)(b4) β0=0.25; (c1)(d4) β0=1.00; (e1)(f4) β0=4.00
在大气中,虽然β0=0.25和β0=4.00的光束在源平面处的像散程度相同,但是风分别沿着光束的长轴和短轴方向吹,即沿风向的束宽大小不一样,导致两者的热晕效应强弱不同。在近场传输的z=1 km处,β0=0.25的光斑畸变严重,甚至出现两个峰值[
3.3 光束质量
热晕效应对具有不同像散程度的椭圆高斯光束的大气传输的影响不同,为了描述光斑的散焦、畸变程度等,引入以下光束质量评价参数。
光束重心可以用来描述光斑的偏移程度:
二阶矩束宽和环围束宽可以用来描述光斑大小,表达式分别为
3.4 像散特性
在大气中,由于衍射效应和热晕效应的作用,椭圆光束的像散特性将发生改变。像散参数wy/wx可以描述光束的对称性,其值越接近1,光束光强分布越对称。
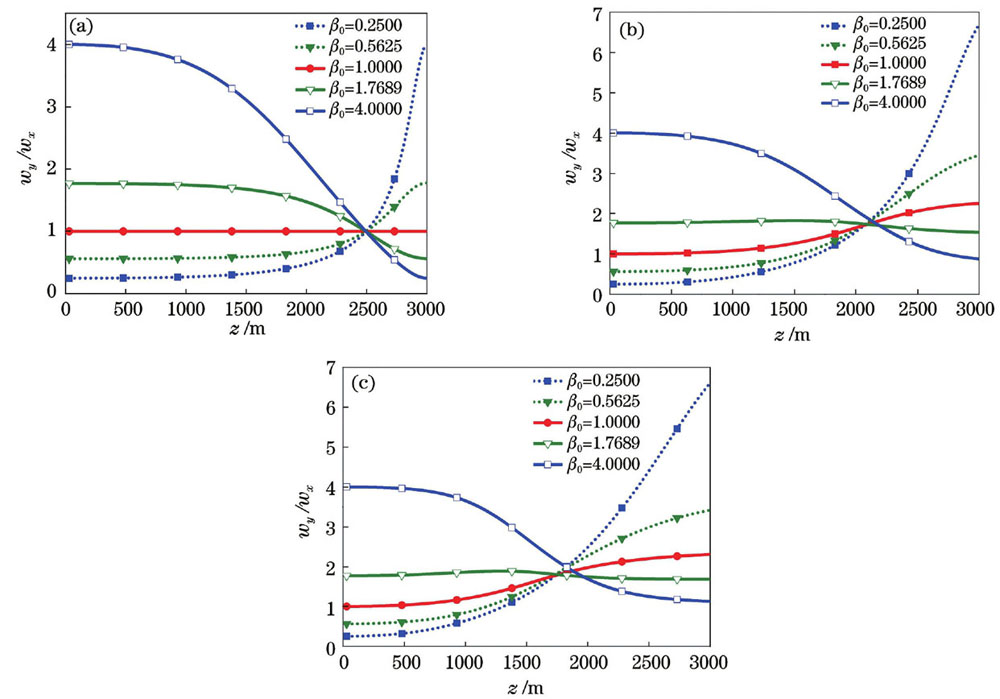
图 5. 光束对称性随传输距离的变化。(a)在自由空间;(b)在大气中,v=10 m/s;(c)在大气中,v=4 m/s
Fig. 5. Beam symmetry versus propagation distance. (a) In free space; (b) in atmosphere,v=10 m/s; (c) in atmosphere,v=4 m/s
利用ABCD定律,可得到聚焦椭圆高斯光束在自由空间传输时x和y方向的束宽[30]:
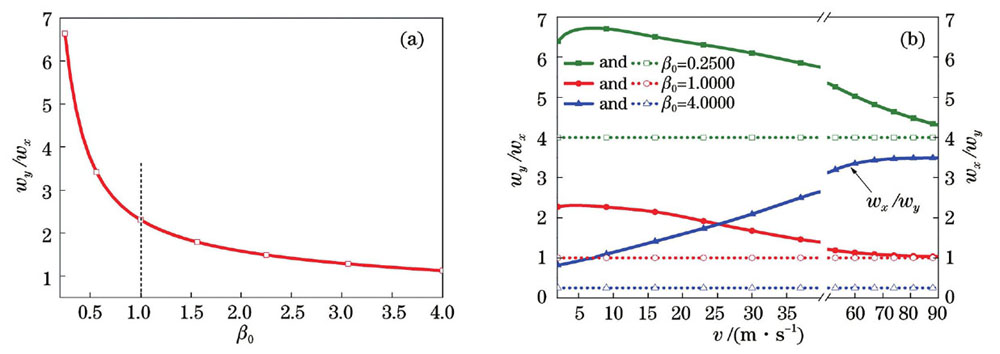
图 6. 靶面像散参数随不同参数的变化。(a) β0;(b) v(实线表示光束在大气中传输,虚线表示光束在自由空间中传输,仅标注的实线对应wx/w,其余对应wy/wx)
Fig. 6. Astigmatic parameter at target surface versus different parameters. (a) β0;(b) v (solid line indicates that beam is transmitted in atmosphere, while dashed line indicates that beam is transmitted in free space. Only marked solid curve corresponds to wx/wy,and other curves correspond to wy/wx)
3.5 焦移和重心漂移
热晕效应会致使光束出现不同程度的发散,因而光束聚焦位置将发生变化,即焦移量改变。
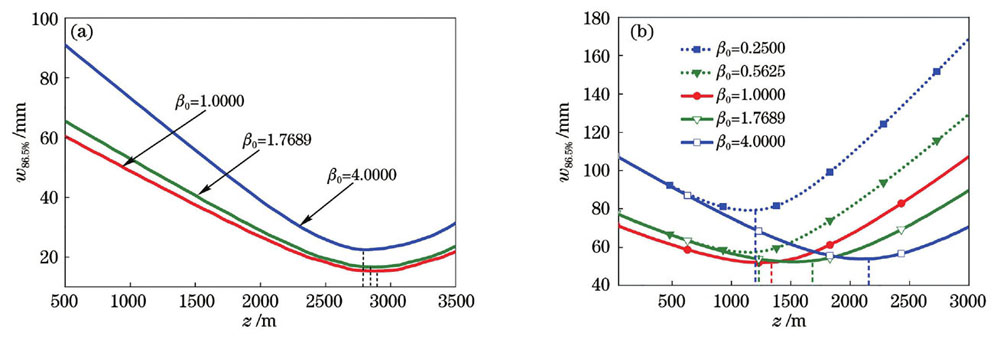
图 7. w86.5%随传输距离的变化。(a)在自由空间中;(b)在大气中
Fig. 7. w86.5% versus propagation distance. (a) In free space; (b) in atmosphere
焦移ΔzF定义为实际焦点位置与靶面之间的距离。
3.6 热晕效应的时间尺度
当风带走的热量和大气吸收的热量相等时,热晕效应达到稳态。这里研究的均为热晕效应达到稳态时的情况。

图 10. 热晕效应的稳态时间。(a)靶面光束重心随时间的变化;(b) 稳态时间ts随β0的变化
Fig. 10. Steady-state time of thermal blooming. (a) Centroid position at target surface versus time; (b) steady-state time ts versus β0
4 结论
自行编制了四维仿真程序,通过解析和数值模拟方法研究了风控热晕下椭圆激光光束质量的优化问题。推导出了椭圆高斯光束在大气中传输的稳态光强公式及其扭曲参数的解析公式,并且证明了其正确性。研究表明:源平面光束的像散参数β0越大,迎风方向的束宽越大,沿风方向的束宽越小,椭圆高斯光束受热晕效应的影响越弱。在自由空间中,椭圆高斯光束在靶面上的长短轴互换。特别地,源平面处具有相同光斑面积但像散参数不同的聚焦椭圆高斯光束在某一传输距离下均变成圆对称分布,并给出了该传输距离公式。但是,大气热晕效应会破坏该特点。由于椭圆光束的像散特性,大气热晕效应对其传输的影响与风向密切相关。在实际工作中,为了减小热晕效应的影响,应将椭圆光束的短轴尽量调整为沿着大气的总体风向。在大气中,源平面光束的像散参数β0越大,椭圆高斯光束靶面上的对称性越好,能量集中度越高且焦移越小。椭圆光束达到稳态热晕的时间也随β0的增大而缩短,与源平面上沿风方向的束宽成正比。所得结论对高能激光大气传输的应用有一定的指导意义。
[1] Gebhardt F G. Twenty-five years of thermal blooming: an overview[J]. Proceedings of SPIE, 1990, 1221: 2-25.
[2] Smith D C. High-power laser propagation: thermal blooming[J]. Proceedings of the IEEE, 1977, 65(12): 1679-1714.
[3] Banakh V A, Falits A V. Efficiency of combined beam focusing under thermal blooming[J]. Atmospheric and Oceanic Optics, 2014, 27(3): 211-217.
[4] 王英俭,范承玉,魏合理. 激光在大气和海水中传输及应用[M]. 北京:国防工业出版社,2015: 157.
WangY J, FanC Y, WeiH L. Laser beam propagation and applications through the atmosphere and sea water[M]. Beijing: National Defense Industry Press, 2015: 157.
[5] Smith D C. Thermal defocusing of CO2 laser radiation in gases[J]. IEEE Journal of Quantum Electronics, 1969, 5(12): 600-607.
[6] Rohde R S, Buser R G. Index of refraction turbulence effects on thermal blooming in laboratory experiments[J]. Applied Optics, 1979, 18(5): 698-704.
[7] Gebhardt F G, Smith D C. Self-induced thermal distortion in the near field for a laser beam in a moving medium[J]. IEEE Journal of Quantum Electronics, 1971, 7(2): 63-73.
[8] Yong K L, Yan J W, Huang S M, et al. Thermal blooming effect of pulse vortex laser beam propagating through the atmosphere[J]. Proceedings of SPIE, 2019, 1084: 108400G.
[9] Spencer M F. Wave-optics investigation of turbulence thermal blooming interaction: II. using time-dependent simulations[J]. Optical Engineering, 2020, 59(8): 081805.
[10] Fleck J A, Morris J R, Feit M D. Time-dependent propagation of high energy laser beams through the atmosphere[J]. Applied Physics, 1976, 10(2): 129-160.
[11] Ding Z L, Li X Q, Cao J Y, et al. Influence of thermal blooming on the beam quality of an array of Hermite-Gaussian beams propagating in the atmosphere[J]. Applied Optics, 2020, 59(34): 10944.
[12] 张鹏飞, 范承玉, 乔春红, 等. 聚焦光束热晕效应相位补偿定标规律研究[J]. 中国激光, 2012, 39(2): 0213002.
[13] Vorob’Ev V V, Murav’Ev N I, Sorokin Y M, et al. Thermal self-interaction of annular laser beams in a moving medium[J]. Soviet Journal of Quantum Electronics, 1977, 7(11): 1333-1336.
[14] Zhao L, Wang J, Guo M J, et al. Steady-state thermal blooming effect of vortex beam propagation through the atmosphere[J]. Optics & Laser Technology, 2021, 139: 106982.
[15] Ji X L, Eyyuboǧlu H T, Ji G M, et al. Propagation of an Airy beam through the atmosphere[J]. Optics Express, 2013, 21(2): 2154-2164.
[16] 李晓庆, 曹建勇, 丁洲林, 等. 列阵平顶光束大气传输的热晕效应[J]. 光学学报, 2019, 39(1): 0126020.
[17] 辛光泽, 陈东启, 蔡毅, 等. 提高半导体激光二极管功率密度的光束整形方法[J]. 红外与激光工程, 2019, 48(8): 0805010.
[18] Honea E, Afzal R S, Savage-Leuchs M, et al. Advances in fiber laser spectral beam combining for power scaling[J]. Proceedings of SPIE, 2016, 9730: 97300Y.
[19] Zheng Y, Yang Y F, Wang J H, et al. Effective dispersion compensation of variable-linewidth fiber amplifier by single-multilayer dielectric grating[J]. Journal of Optics, 2016, 18(6): 065610.
[20] 李冬, 梅小华, 吴逢铁. 椭圆高斯光束产生近似无衍射Bessel-Gauss光[J]. 强激光与粒子束, 2014, 26(5): 90-93.
Li D, Mei X H, Wu F T. Generation of non-diffracting Bessel-Gauss like beam by elliptical Gauss beam[J]. High Power Laser and Particle Beams, 2014, 26(5): 90-93.
[21] Zheng C W. Propagation of elliptical Gaussian beam passing through apertured paraxial optical systems[J]. Optik, 2014, 125(1): 264-267.
[22] Cai Y J, He S L. Average intensity and spreading of an elliptical Gaussian beam propagating in a turbulent atmosphere[J]. Optics Letters, 2006, 31(5): 568-570.
[23] Wang H Y, Li X Y. Spectral shifts and spectral switches of elliptical Gaussian beam propagating through turbulent atmosphere[J]. Optics & Laser Technology, 2009, 41(2): 107-111.
[24] Zhang J B, Xie J T, Ye F, et al. Effects of the turbulent atmosphere and the oceanic turbulence on the propagation of a rotating elliptical Gaussian beam[J]. Applied Physics B, 2018, 124(8): 1-11.
[25] Singh T, Saini N S, Kaul S S. Dynamics of self-focusing and self-phase modulation of elliptic Gaussian laser beam in a Kerr-medium[J]. Pramana, 2000, 55(3): 423-431.
[26] 陈国柱, 沈咏, 刘曲, 等. 利用椭圆高斯光束产生266 nm紫外连续激光[J]. 物理学报, 2014, 63(5): 054204.
Chen G Z, Shen Y, Liu Q, et al. Generation of 266 nm continuous-wave with elliptical Gaussian beams[J]. Acta Physica Sinica, 2014, 63(5): 054204.
[27] Kumar H, Aggarwal M, Richa , et al. Self-focusing of an elliptic-Gaussian laser beam in relativistic ponderomotive plasma using a ramp density profile[J]. Journal of the Optical Society of America B, 2018, 35(7): 1635-1641.
[28] Walia K, Tripathi D. Self-focusing of elliptical laser beam in cold quantum plasma[J]. Optik, 2019, 186: 46-51.
[29] Cornolti F, Lucchesi M, Zambon B. Elliptic Gaussian beam self-focusing in nonlinear media[J]. Optics Communications, 1990, 75(2): 129-135.
[30] 胡婧, 王欢, 季小玲. Kerr非线性介质中聚焦像散高斯光束的传输特性[J]. 物理学报, 2021, 70(7): 074205.
Hu J, Wang H, Ji X L. Propagation characteristics of focused astigmatic Gaussian beams in Kerr nonlinear media[J]. Acta Physica Sinica, 2021: 074205.
Article Outline
邓凌, 李晓庆, 穆轶, 季小玲. 风控热晕下椭圆激光光束质量的优化研究[J]. 中国激光, 2022, 49(4): 0405001. Ling Deng, Xiaoqing Li, Yi Mu, Xiaoling Ji. Beam Quality Optimization of Elliptical Laser Under Wind-Dominated Thermal Blooming[J]. Chinese Journal of Lasers, 2022, 49(4): 0405001.