可调谐二极管激光吸收光谱技术的应用研究进展
下载: 3721次特邀综述
1 引言
气体组分浓度与状态参数(温度、压力、流速等)的准确测量在许多领域都具有非常重要的作用,例如,在大气环境监测领域,大气污染物浓度测量是空气质量评估[1-3]、污染防治[4]的首要基础;在工业过程控制中,中间产物排放的连续检测[5]、气体泄漏检测[6-7]对产品质量、环境保护及安全生产等都具有重要作用;在燃烧流场诊断中,对燃烧温度、产物浓度以及流速测量[8-10],是提高燃烧效率以及评估发动机性能的重要手段;在风洞试验中[11],流场参数的精确测量是保证风洞试验有效性不可或缺的手段;在深海探测中,对深海溶解气体及其同位素精确检测,是了解深海热液和冷泉系统的关键途径,也是了解深海极端环境的窗口[12];人体呼吸气体检测,能实现对人体疾病的快速无损检查[13];对液态水(例如水膜、油膜)的实时快速测量,是了解液膜形成过程及蒸发现象的有效方法[14]。而传统气体测量方法存在着一些弊端,限制了其在实际气体测量中的应用,例如质谱法[15]响应速度慢,仪器体积质量较大,价格较高,很难实现原位在线测量;气相色谱法[16]无法实现原位在线测量;电化学传感[17]通常含有有毒有害材料,而且重复性差。光谱学方法利用气体分子对光的吸收、发射及散射的原理工作,具有非接触、快速响应、良好选择性等优点,能较好满足不同环境条件下气体测量的要求。
针对气体检测的需要,国内外发展了多种基于光谱学的气体探测方法[18]。主要有激光诱导荧光(LIF)[19-20]、拉曼光谱法(Raman)[21-22]、傅里叶变换红外光谱(FTIR)[23-24]、非色散红外光谱(NDIR)[25-26]、光声光谱(PAS)[27-29]、差分吸收光谱(DOAS)[30-32]、激光雷达(LIDAR)[33]、腔增强吸收光谱(CEAS)[34-35]、腔衰荡光谱(CRDS)[36-37]以及可调谐二极管激光吸收光谱 (TDLAS)[38-40]。其中TDLAS技术采用可调谐二极管激光器,通过改变激光器输入电流或温度来调谐激光器输出波长,使其扫描气体分子单根或多根完整的吸收线,获得高分辨率的气体吸收光谱,对光谱进行分析获得气体参数信息。与其他气体检测方法对比,TDLAS技术有如下特点:原位、连续、实时测量;环境适应性强(能适应高温高压、低温低压、高湿、高流速、腐蚀性环境);选择性强(测量目标气体光谱受到其他气体吸收光谱干扰小);灵敏度高(检测限可达到10-9量级);可靠性高;成本低,使用过程没有消耗品;无需预处理,免标定测量;操作简单,数据处理简便快速;易于小型化,非常适合实际工程应用。
近年来,国内外的研究机构对TDLAS技术在气体传感探测方面进行了大量的理论及应用研究。本文首先对TDLAS技术气体测量的原理及方法、各类激光器特性以及典型激光波长对应的气体检测限进行介绍,其次针对TDLAS技术在大气环境监测、工业过程监测、深海溶解气体探测、人体呼吸气体测量、流场诊断以及液态水测量六个应用领域的最新应用及存在的挑战进行综述,最后展望TDLAS技术在气体检测领域的发展与应用。
2 TDLAS技术原理
2.1 红外波段气体分子吸收光谱
一般来说,红外光谱为分子的振动跃迁光谱[41]。
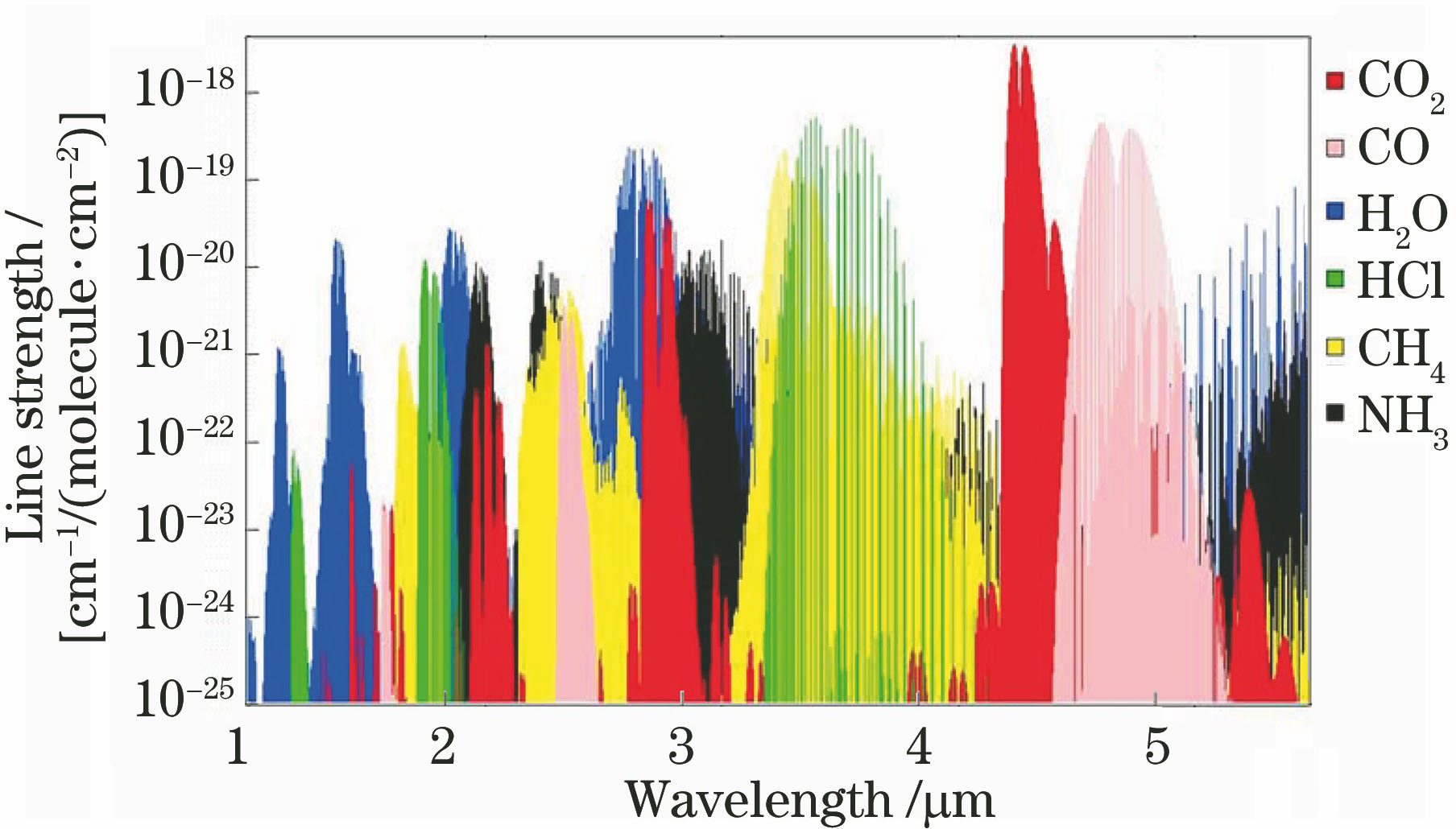
图 1. 根据HITRAN数据库模拟部分气体的吸收线
Fig. 1. Absorption spectra of several gas molecules, data from spectroscopic database HITRAN
红外波段包含了大量气体分子基频、泛频及合频谱带,所以具有大量的跃迁吸收线。HITRAN[42]、HITEMP[43]及GEISA[44]光谱数据库较详细地列出了各吸收谱线的光谱参数。由于数据库中光谱参数部分是理论计算的结果,与实际环境情况存在一定的误差。所以,国内外研究者在实验室利用可控式实验仪器,模拟不同环境,对各种气体的光谱参数进行了实际测量。例如,Mondelain等[45]运用TDLAS技术,测量得到了4条CH4气体
2.2 激光器
激光器作为激光气体传感器的光源,应该具备紧凑、稳定、寿命长以及输出功率高等特点;同时,由于TDLAS技术需要扫描气体的单根或多根完整的吸收线,要求激光器线宽远小于吸收光谱线宽。在实际TDLAS气体检测应用中,使用较多的激光器有分布反馈(DFB)二极管激光器、垂直腔表面发射激光器(VCSELs)、外腔二极管激光器(ECDL)、带间级联激光器(ICL)和量子级联激光器(QCL)。一般采用蝶形、TO、C-mount或高热负载HHL(High Heat Load)方式封装,并且含有一个波长选择器件(例如光栅)使其发射出特定波长的光。近红外DFB激光器典型的输出功率在10 mW左右,带宽2 MHz左右,工作波长范围一般在730~3000 nm[62-64],可在室温环境下工作,无需制冷,一般能带尾纤输出。近红外ECDL是通过腔外光栅运动来调谐激光器输出波长,实现更宽范围的连续波长扫描(例如1490~1580 nm)[65-66],但其调制频率不高,偏振噪声大,不适合气体的快速测量,通常只适用于实验室。Sonnenfroh等[67]利用ECDL测量了1.57 μm附近的CO和CO2吸收光谱。VCSEL是沿着激光器表面方向发射激光的,所以VCSEL具有较小的发射角 [68]。Shau和Ortsiefer等[69-72]在1.4~2.3 μm波长范围运用VCSEL进行气体测量。与DFB相比,VCSEL具有较宽的调谐范围(约5 nm),较小的阈值电流,电流调谐率(Δ
激光器频率稳定是TDLAS气体传感器性能稳定的前提,通常可以通过艾伦方差[81]对激光器频率稳定性进行分析,采用激光器温度补偿等方法[82-83]对激光器的频移现象进行修正。由于单只激光器的调谐范围较小(DFB:1~2 nm,VCSEL:5~10 nm),为了满足多种气体探测的需要,发展了波分复用技术、时分复用技术及频分复用技术等[84-85]。但是在某些波段处,一个激光器能同时覆盖多种气体的吸收线,能实现多种气体同时测量[86]。在实际测量中,需根据实际环境状态(温度、浓度、压力等),选择合适的激光器进行测量,Zhou等[84,87]详细介绍了吸收线选择要求及方法。
2.3 检测限
TDLAS技术通过选用不同激光波长,可以实现不同气体的检测。对于同一种气体测量,选用不同波长能获得不同的检测极限,假设最小的可探测吸光度为10-5,带宽为1 Hz,有效吸收光程为1 m,各种气体探测的典型波长与浓度检测限如
利用中红外调制光谱技术与长光程结合,能使浓度检测限继续降低至10-12量级[89]。文献[ 90-92]也给出了不同气体的检测限。
表 1. TDLAS 技术的典型浓度检测限[88]
Table 1. Typical concentration detection limits in TDLAS technology[88]
|
2.4 直接吸收光谱技术
直接吸收光谱技术是TDLAS中常用的一种方法,这种方法装置结构简单,信号处理相对简易,测量结果无需标定。
由Beer-lambert吸收定律,某条孤立吸收线的积分吸光度可表示为
式中:
浓度测量[97-99]是通过拟合获得的积分吸光度值或吸收光谱峰值,结合已知的环境总压及有效吸收光程,根据(1)式计算出气体体积分数。流速测量[100-101]是基于多普勒效应,当气体流速在激光传输方向有速度分量时,会造成气体分子吸收光子,探测器接收吸收峰频率与静态吸收频率会产生频移(多普勒频移),通过频移计算气体流速。
2.5 调制光谱技术
一般而言,由于激光器、探测器以及电子学等噪声的影响,直接吸收技术能探测的最小吸光度在10-3量级,对于更小的吸光度探测(例如10-4及更小),一般采用调制技术,包括波长调制光谱技术(WMS)[102]和频率调制光谱技术(FMS)[103]。与WMS和FMS不同的调制技术还有双频调制光谱技术[104],差分检测技术[105],自动平衡检测技术[106]等。导数吸收光谱分析技术[107-108]是利用电子学微分方法对硬件电路中的光谱信号进行微分处理,该方法能将某一痕量气体的光谱从较宽的干扰峰或者其他背景干扰中分离出来,并且能够分辨出吸光度随波长急剧上升所掩盖的弱吸收峰,但其对和吸收频率相近的噪声成分抑制能力较弱。
波长调制技术是将低频扫描锯齿波和高频调制正弦波同时加载至激光器上,被调制的激光光束被气体吸收后到达探测器,然后由锁相放大器解调出透过率各阶次谐波信号,根据二次谐波信号与气体体积分数成正比的关系实现气体体积分数的测量:
当
对于那些不易标定的测试环境,需要研究WMS免标定测量方法。近几年发展的WMS免标定方法主要以WMS-2
3 TDLAS技术的应用研究进展
3.1 大气环境监测
近年来,国家非常重视全球环境和气候变化问题,我国在近20年来,气象灾害损失已达到GDP的3%~6%,是世界上经济损失最严重的国家。同时,对大气环境进行监测,了解地球大气状态,为大气环境保护和污染修复提供数据参考,是我国履行国际公约不可回避的义务。
大气温室气体的测量,是研究其浓度变化及源和汇的基础。近地面的温室气体测量不仅需要测量其浓度水平,还需要了解浓度随时间的变化情况。为了更好地了解区域范围温室气体源和汇的分布,有必要研究温室气体在不同区域不同时间范围内的变化情况。对于大型的增汇复杂生态系统,下垫面的非均一性及天气过程的不可重复性,使得温室气体的分布存在较大的时空变异性,故需要分辨率高、监测尺度广、准确性高和可实现尺度转换的自动监测技术。另外,在气候变化研究中需要的是年增长量的监测,而传统仪器设备只能实现浓度监测。
在大气灰霾方面,二次细颗粒物污染主要是由NO、NO2、NH3、SO2、VOC这些前体物相互反应形成的。这些前体物的监测是研究PM2.5形成与演化过程的关键[2]。但这些污染气体,正常空气中体积分数较小(约10-9)、变化范围大(10-9~10-6)、变化速度快,现有的监测仪器因灵敏度低、响应时间慢、动态范围小等缺点,不能进行准确的测量。
TDLAS技术光谱分辨率非常高,检测灵敏度较高,非常适合大气环境气体实时连续在线监测。当前,包括中国科学院安徽光学精密机械研究所(以下简称中科院安光所)在内的多家国内外机构利用TDLAS技术实现了多种温室气体(例如H2O, CO2, CH4以及N2O等)的天地一体高精度实时测量[38-41,110,113],同时实现了NO, NO2, NH3, SO2以及H2S等污染气体的测量[31,36,85,99,114-115] 以及农业和森林等大尺度、大范围的气体监测,为大气气体立体监测提供了重要的技术保障。
![(a)基于波长调制光谱技术进行大气气体探测的装置图及(b) NO2, (c) NH3和(d) NO在线测量的结果[115]](/richHtml/zgjg/2018/45/9/0911001/img_2.jpg)
图 2. (a)基于波长调制光谱技术进行大气气体探测的装置图及(b) NO2, (c) NH3和(d) NO在线测量的结果[115]
Fig. 2. (a) Device diagram for atmospheric gas sensing based on wavelength modulation spectroscopy technique and the results of continuous online monitoring of (b) NO2, (c) NH3 and (d) NO[115]
TDLAS技术为视线平均测量,它测量得到的是光路上的平均浓度。对于大范围的空气质量评估需要进行大范围的采样或移动测量,这增加了测量过程的复杂性,另外,由于当前可调谐二极管激光器扫描范围具有一定的限制,对多种气体进行检测时需要多个激光器同时工作,这必然会导致仪器成本较高。未来随着半导体激光器的发展,激光器调谐范围将进一步增加,TDLAS测量系统的成本将随之降低,其在大气环境监测中的应用规模也将大大增加。
3.2 工业过程应用
在工业生产过程中,为了保证产品质量、生产安全以及工艺效率,需要对生产过程中的气体进行监测。钢铁制造业中,通过实时监测控制电弧炉O2的注入量,以实现脱磷、脱碳、去除杂质及迅速均匀加热至出钢温度的目的[116-118];火力发电厂中,为了节能减排以及提高发电效率,燃烧气体组分(CO2,CO,O2,SO2等)及温度的测量也非常重要[119-120];石油化工产业对气体测量有巨大的需求,例如催化裂化过程中O2、CO2、CO的测量,合成氨中的O2、CO2、CO、H2S、SO2、CH4、NH3等的测量,尿素合成中O2、CO2、NH3的测量以及硫磺回收中O2, H2S, SO2等的测量[121-122];半导体产业中,为保证精密产品的质量,对反应气体纯度要求极高,需要严格监测杂质气体的浓度[123];安全生产领域,对氨气逃逸及天然气泄漏进行监测[124-125],炸药存放过程中对三硝基甲苯等材料分解产生的NO和NO2进行实时在线监测[126]等,是保证安全生产的关键;人们生产生活环境中,尤其是工业园区的恶臭气体[127](例如:氨、硫化氢、二硫化碳、甲硫醇、甲硫醚、二甲二硫、苯乙烯、三甲胺等)也需要实时准确地进行监控。
针对工业过程TDLAS气体监测,已有大量的研究成果。Pisano等[128]利用TDLAS技术实现了柴油汽车痕量逃逸氨的测量,浙江大学王飞等[129]测量了含有颗粒的氨气浓度,Schloser和Qu等[130-131]利用TDLAS技术测量了高温煤燃烧过程中的钾原子浓度,中国石油大学[132]针对石油天然气开采现场可能泄露的甲烷和硫化氢气体,研制了开放光路下基于TDLAS技术的隧道及天然气开采现场甲烷及硫化氢气体复合一体检测装置,Jenkins等[133]运用TDLAS技术在燃煤电厂中进行了原位温度测量,Walter等[134]运用TDLAS技术对垃圾焚烧过程产生的气体进行测量,Ebert等[135]运用TDLAS技术对燃气电厂进行了温度及多组分气体浓度的测量,Hirmke等[136]运用TDLAS技术检测金刚石生长过程中产生的气体,Chou等[137]利用TDLAS技术测量了等离子体腐蚀炉中的HBr,中科院安光所[138-143]利用TDLAS技术实现了天然气管道泄漏的定量遥测,氨逃逸的在线监测,工业过程产生的H2S, CH4,O2以及燃烧过程产生的CO, CO2等的浓度测量,汽车尾气的路边监测,酒驾遥测等。
![(a)TDLAS技术与光闪烁互相关技术结合测量工业总排放装置示意图及(b)氧气浓度及(c)流速测量的结果[141]](/richHtml/zgjg/2018/45/9/0911001/img_3.jpg)
图 3. (a)TDLAS技术与光闪烁互相关技术结合测量工业总排放装置示意图及(b)氧气浓度及(c)流速测量的结果[141]
Fig. 3. (a) Schematic diagram of the online total emission measurement experimental setup and the continuous measurement results of (b) oxygen concentration and (c) oxygen flow rate[141]
TDLAS技术在工业应用过程中面临的问题主要体现在测量对象及测量环境越来越复杂,从层流到湍流、从清洁火焰到碳烟火焰(气态燃料到液体、固体燃料)、从常/低压强环境到高压强环境、从高温/常温到极低温环境等;测量的气体组分越来越复杂,需要监测的气体的浓度越来越低。这就对TDLAS技术的环境适应性及系统工作的长期稳定性提出了更加严格的要求,同时也对不同气体的检测限要求越来越低,这就需要更加先进的去噪技术及长光程技术。将来,随着TDLAS技术的进一步发展,及其与其他光谱学和非光谱学方法的交叉融合,其在工业领域中发挥的作用将越来越大,应用范围也必然会进一步扩大。
3.3 呼吸气诊断
呼吸气诊断是一种无创、精确且安全的疾病诊断或评估技术,是生物医学工程领域的一个热点发展方向[144]。通过人体生理或病理状态时呼出气中特定成分的存在与否或含量多少来实现对特定疾病的诊断。现已知呼出气体中一些可被即刻检测到的挥发性有机物(如烃类、二氧化碳、一氧化氮和一氧化碳等)和可在冷凝后检测到的非挥发性有机物(如8-异前列烷,过氧化氢、亚硝酸盐和硝基酪氨盐等)是某些呼吸系统疾病的潜在生物标志物[146]。
表 2. 生理症状与对应的气体分子标志物及TDLAS激光测量典型波长
Table 2. Physiological symptoms corresponding to their breath biomarker and typical laser wavelengths for TDLAS
|
![(a) TDLAS技术病人呼吸气测量原理图及(b) 15NO,14NO测量结果[152]](/richHtml/zgjg/2018/45/9/0911001/img_4.jpg)
图 4. (a) TDLAS技术病人呼吸气测量原理图及(b) 15NO,14NO测量结果[152]
Fig. 4. (a) Schematic configuration of a TDLAS system and (b) measurement of 15NO and 14NO concentration[152]
人体的呼吸气中,氮气、氧气、二氧化碳以及氩气占99%,其余1%包含约2000种以上不同气体,体积分数在10-12至10-6间[148,150-151]。目前已有应用TDLAS检测技术对部分疾病进行无创检测,并获得了较好的检测效果,例如斯坦福大学Wang等[152]应用中红外吸收光谱技术结合法拉第旋光光谱实现人体呼吸气中浓度较低的NO及其同位素测量(装置与实验结果如
人体呼吸气温度与环境温度之间存在着差异,使得呼出气中的水汽很容易液化而对测量造成影响,且不同气体的吸收干扰会影响测量精度,所以疾病与呼出气含量间的定量关系的普适性等是目前TDLAS呼吸气诊断中面临的问题。随着对人体呼吸气与相关疾病关系间的深入研究,以及具有更低检测限能力的TDLAS技术的发展,TDLAS技术呼吸检测未来发展的方向将是实现更多种呼吸气测量以及更多种疾病的无创诊断和评估,同时提高相关仪器的便携性、稳定性与安全性。
3.4 深海溶解气体探测
海洋是大气温室气体(例如甲烷和二氧化碳)的重要排放源,大气中甲烷的2%~4%来源于海洋[153],因此对深海溶解气体进行的原位测量,对海洋生态系统、碳循环系统的研究以及海底能源的勘探等都具有重要意义[154]。针对水下溶解气体的原位探测,国内外先后研制了水下质谱仪[155-156]、半导体气敏传感器[157]以及非分散红外吸收光谱技术(NDIR)。水下质谱仪无法满足水下长时间的实时准确探测需求;SnO2半导体气敏传感器因气体在半导体表面发生的氧化还原反应其敏感元件阻值发生变化,所以其测量结果受含氧量的影响较大,相关仪器的稳定性有待进一步强化;而NDIR技术因采用宽带光源,其抗干扰性和灵敏度会受到限制。
近年来,国内外科研机构将目标瞄向了高分辨的激光光谱探测方法。英国国家海洋中心Boulart[158]认为光学技术是溶解气体的未来。哈佛大学的Wankel等[159]利用离轴积分腔输出光谱分析仪(OA-ICOS)在美国蒙特雷湾区域实现了对3000 m海深的冷泉沉积物中CH4碳同位素的丰度测量,但是测量CH4浓度的下限只达5×10-4(如
![(a)离轴积分腔输出光谱分析仪实验装置图及(b)甲烷稳定同位素测量的结果[159]](/richHtml/zgjg/2018/45/9/0911001/img_5.jpg)
图 5. (a)离轴积分腔输出光谱分析仪实验装置图及(b)甲烷稳定同位素测量的结果[159]
Fig. 5. (a) Schematic of instrument layout and methane carbon stable isotopic composition (δ13CCH4) measured via the in situ ICOS analyzer and (b) a conventional isotope ratio mass spectrometry (IRMS)[159]
在深海海水溶解气体检测时,对TDLAS技术的耐压能力要求较高,同时要保证海水取样及水汽分离技术的精度。因此,深海溶解气体及其同位素检测中,需要使用气体检测范围宽的TDLAS技术、功率较高的激光器以及成熟的水汽分离技术。
3.5 流场诊断
随着**事业和航天航空技术的不断发展,航空发动机燃烧流场诊断、性能评估以及风洞流场测量受到越来越多的关注。传统的流场诊断设备如压力传感器、侵入式探针、热电偶等存在干扰待测流场、响应速度慢、灵敏度低等缺点。此外,很多探测设备无法满足高超音速飞行器在高温、高速等恶劣环境下的工作需求。TDLAS以H2O、CO2、CO、O2等气体组分为探针,可以有效实现发动机燃烧流场以及风洞流场中温度、压力、流速和气体浓度等参数的非侵入式、快速、实时在线测量,为超燃冲压发动机的气动研究、关键部件实验、地基性能评估、推助力计算以及天地一致性验证提供有力的技术支持[9,11]。
TDLAS流场诊断在测量维度上分为一维诊断和二维诊断,一维诊断是基于视线平均的测量,测量光路上流场参数(温度、浓度等)的平均值[8,9,95-98]。这种测量方法装置结构简单,数据处理算法简便,但是只能反映流场状态的视线平均值。二维诊断是随着断层扫描(CT)技术以及扫描系统和图像处理技术的进步而发展起来的,当前断层扫描技术已被广泛应用于流体力学及燃烧诊断领域[10]。对流场温度及浓度等信息的二维分布进行扫描测量,根据扫描形式可分为移动旋转方式[164]和空间固定方式[165]。流场二维诊断较一维测量数据处理算法更复杂,主要有代数迭代(ART)算法[166-167]、滤波反投影(FBP)算法[168],卷积反投影算法[169]、Abel变换、最大然近似估算法[170],超光谱重建法[171],虚拟光线方法[172]等。
美国Stanford大学最早在20世纪70年代开展了激波管流场激光吸收光谱测量研究,先后在实验室开展了激波管中氧气的速度、温度、压力和质量流量的测量研究,为动力学的研究和碳氢燃料分解速率的探测奠定了基础[173-174]。同时,该小组在实验室和全尺寸发动机的地面测试中都开展了将TDLAS技术用于脉冲爆震发动机(PDE)的研究,证明了TDLAS技术能成功被应用于恶劣和动态环境中。国内如中科院安光所、中国科学院力学研究所、西北核工业研究所、西北大学、装备指挥学院等单位都开展了燃烧流场TDLAS测量,并在地面台架实验研究中进行了应用。目前已经完成了汽化炉[175]、喷气发动机燃烧室羽流[176]、激波管[177]、航空发动机进气道/燃烧室[178]、超燃冲压发动机隔离段/燃烧室[179]、高超声速燃烧加热风洞等燃烧设备的温度、组分浓度和速度的测量。
![(a)高超声速燃烧加热风洞来流测量装置[180]及(b)~(d)测量结果](/richHtml/zgjg/2018/45/9/0911001/img_6.jpg)
图 6. (a)高超声速燃烧加热风洞来流测量装置[180]及(b)~(d)测量结果
Fig. 6. (a) Measuring device and (b)-(d) results of hypersonic combustion heating wind tunnel[180]
![重建结果。(a)温度二维分布结果;(b)水汽浓度(体积分数)分布的结果[181]](/richHtml/zgjg/2018/45/9/0911001/img_7.jpg)
图 7. 重建结果。(a)温度二维分布结果;(b)水汽浓度(体积分数)分布的结果[181]
Fig. 7. Reconstructed distribution results. (a) Temperature; (b) water vapour concentration (volume fraction) [181]
当前,TDLAS技术在燃烧诊断领域已经有了较为成熟的研究结果,但是由于我国在航空航天的迅速发展,对流场测量的需求越来越苛刻,如:从一维测量需求转向高维测量需求,从低重复频率测量需求转向高重复频率测量需求,集成的测量装置转向智能、小型的需求等。这些需求的提升使得TDLAS技术需要采用更加先进的测量策略(例如,采用高速采集测量分析超快过程),同时发展更加高效、智能的算法程序以用于多维重建等。
3.6 液态水测量
对液膜厚度进行定量分析,不仅可以设计和优化工业过程,保证工业过程的安全,也能为仿真模拟提供确切可信的数据,还可以对蒸发现象进行研究,因此液膜的测量广泛地应用于各领域中。例如Darke等[182]针对液体燃料油膜可能导致的碳氢化合物沉积及污染物排放量增加,对直喷发动机活塞表面油膜进行了测量;Hentschel等[183]利用激光诱导荧光技术对汽油机进油管道壁面油膜厚度进行了测量;Mawhinney等[184-188]利用TDLAS技术测量了不同溶液中水膜的厚度。
液态水与气态水不同,液态水由于范德瓦尔斯氢桥键的结合,阻碍了水分子的转动,在近红外光谱范围表现为O—H的伸缩振动,又由于H原子相对O原子较轻,其振动幅度比较大,加上诸多吸收峰的迭加,使得液态水吸收为宽带吸收,而且光谱位置会随着温度的升高而向短波方向移动[189], Maréchal[190]详细介绍了液态水在整个红外波段的吸收特性(如
对于
![普通液态水(实线,H区域)和重水(虚线,D区域) 在三个不同温度下的吸收光谱[190]](/richHtml/zgjg/2018/45/9/0911001/img_8.jpg)
图 8. 普通液态水(实线,H区域)和重水(虚线,D区域) 在三个不同温度下的吸收光谱[190]
Fig. 8. Absorption spectra of ordinary water (full lines, H area) and heavy water (dotted line, D area) at three selected temperatures[190]
利用光谱学的方法对液态水进行测量,具有高精度、高灵敏度及无干扰等优点。Mouza等[193]利用635 nm的激光器测量了低至1 mm的掺杂亚甲蓝的水膜厚度;Porter等[194]发明了一种双波长中红外激光吸收测量技术用于同时测量气态燃料的摩尔分数和油膜厚度;Yang等[185-187]采用的TDLAS技术,研制了基于波长在1.4 μm附近多个DFB二极管激光器的TDLAS系统,同时测量得到了普通液态水膜厚度、衰减系数、液态水温度及蒸发过程气态水温度。
4 结论
本文综述了TDLAS技术的应用研究现状,首先介绍了TDLAS技术气体检测的特点及原理,然后介绍了TDLAS在大气环境监测、工业过程监测、深海溶解气体测量、人体呼吸气体测量、流场诊断以及液态水测量中的应用背景以及研究现状。TDLAS技术在各领域气体检测中具备非接触测量、时间响应速度快、高精度、免标定、易于小型化等优势,其技术应用领域不断扩大。
TDLAS技术经过40多年的发展,已经成为了一种比较成熟的光谱检测技术。在检测原理发展成熟的基础上,相应的硬件(例如可调谐二极管激光器、探测器等)的发展,使得激光检测波长范围不断扩大,测量气体种类不断增多;多次发射池技术的不
![(a) TDLAS液态水挥发过程温度及膜厚度测量装置及(b)结果[186]](/richHtml/zgjg/2018/45/9/0911001/img_9.jpg)
图 9. (a) TDLAS液态水挥发过程温度及膜厚度测量装置及(b)结果[186]
Fig. 9. (a) Experimental setup and time-resolved measurement of film thickness and (b) temperature during film evaporation by TDLAS[186]
断优化,使得TDLAS气体检测浓度极限不断降低;光纤技术、电子学技术及光机结构设计技术的不断发展,使得TDLAS测量仪器趋近于小型化及具备长期稳定性,极大地提高了工程化应用的便利性。但是,TDLAS技术在各领域的应用中仍然面临着一些问题及挑战:首先表现为测量需求越来越苛刻,如从一维测量需求到高维测量需求、从低重复频率测量需求到高重复频率、集成的测量装置转向智能、小型的需求等;其次是测量对象越来越复杂,如从层流到湍流、从清洁火焰到碳烟火焰(气态燃料到液体、固体燃料)、从常/低压强环境到高压强环境、从高温/常温到极低温环境、从地面到深海环境等;最后是测量的气体组分越来复杂,气体的浓度越来越低。所以,TDLAS技术未来的发展主要将朝着以下方向发展:环境适应性更强,测量的光学结构、电子学系统长期稳定性更高,算法更加高效成熟,仪器更加小型智能、检测限更低以及测量参数逐渐增多以满足不同应用领域的需求等。最终,TDLAS技术将在工业、生态、**、健康等领域发挥越来越大的作用。
[1] 董凤忠, 阚瑞峰, 刘文清, 等. 可调谐二极管激光吸收光谱技术及其在大气质量监测中的应用[J]. 量子电子学报, 2005, 22(3): 315-325.
[2] Organization WH, Europe R O F. WHO Guidelines for indoor air quality: selected pollutants[M]. Geneva: World Health Organization, 2010.
[3] 王浩, 李轶, 高健, 等. APEC会议期间石家庄市大气污染特征及空气质量保障措施效果评估[J]. 环境科学研究, 2016, 29(2): 164-174.
[4] Li H M, Wang Q G, Yang M, et al. Chemical characterization and source apportionment of PM 2.5 aerosols in a megacity of Southeast China[J]. Atmospheric Research, 2016, 181: 288-299.
[5] Reidl-Leuthner C, Ofner J, Tomischko W, et al. Simultaneous open-path determination of road side mono-nitrogen oxides employing mid-IR laser spectroscopy[J]. Atmospheric Environment, 2015, 112: 189-195.
[8] LiF, Yu XL, GuH, et al. Measurement of temperature, velocity and water vapor concentration in a scramjet combustor based on near-infrared diode laser absorption[C]∥17 th AIAA International Space Planes and HypersonicSystems and TechnologiesConference, April 11-14, 2011. San Francisco, California. American Institute of Aeronautics and Astronautics, c2013: 167- 185.
[10] Wang F, Wu Q, Huang Q X, et al. Simultaneous measurement of 2-dimensional H2O concentration and temperature distribution in premixed methane/air flame using TDLAS-based tomography technology[J]. Optics Communications, 2015, 346: 53-63.
[13] Wang C J, Sahay P. Breath analysis using laser spectroscopic techniques: breath biomarkers, spectral fingerprints, and detection limits[J]. Sensors, 2009, 9(10): 8230.
[14] Porter J M, Jeffries J B, Hanson R K. Mid-infrared laser-absorption diagnostic for vapor-phase fuel mole fraction and liquid fuel film thickness[J]. Applied Physics B, 2010, 102(2): 345-355.
[18] 刘文清, 崔志成, 董凤忠. 环境污染监测的光学和光谱学技术[J]. 大气与环境光学学报, 2002, 15(5): 1-12.
[19] Stone D, Whalley L K, Ingham T. et al. Measurement of OH reactivity by laser flash photolysis coupled with laser-induced fluorescence spectroscopy[J]. Atmospheric Measurement Techniques, 2016, 9(7): 1-22.
[21] Depciuch J, Kaznowska E, Zawlik I, et al. Application of Raman spectroscopy and infrared spectroscopy in the identification of breast cancer[J]. Applied Spectroscopy, 2016, 70(2): 251-263.
[22] LarkinP. Infrared and Raman spectroscopy : principles and spectral interpretation[M]. Amsterdam: Elsevier, 2011.
[23] Hammer S. Griffith D W T, Konrad G, et al. Assessment of a multi-species in-situ FTIR for precise atmospheric greenhouse gas observations[J]. Atmospheric Measurement Techniques Discussions, 2013, 6(5): 1153-1170.
[24] 李相贤, 王振, 徐亮, 等. 温室气体及碳同位素比值傅里叶变换红外光谱分析的温度依赖关系研究[J]. 红外与激光工程, 2015, 44(4): 1178-1185.
[25] Silver JA, Chen S J. Carbon monoxide sensor for combustion feedback control[C]∥44 th AIAA Aerospace Sciences Meeting and Exhibit, January 9-12, 2006, Reno, Nevada, USA. American Institute of Aeronautics and Astronautics, c2006: 748.
[27] 张建锋, 潘孙强, 林晓露, 等. 可在线校准的大气CO2浓度光声光谱监测系统研究[J]. 光谱学与光谱分析, 2016, 36(1): 1-5.
[28] 马欲飞, 何应, 于欣, 等. 基于中红外量子级联激光器和石英增强光声光谱的CO超高灵敏度检测研究[J]. 物理学报, 2016, 65(6): 060701.
[29] Wojtas J, Gluszek A, Hudzikowski A, et al. Mid-infrared trace gas sensor technology based on intracavity quartz-enhanced photoacoustic spectroscopy[J]. Sensors, 2017, 17(3): 513.
[32] 沈兰兰, 秦敏, 孙伟, 等. 基于车载便携式DOAS对工业园区SO2, NO2和苯的走航观测[J]. 光谱学与光谱分析, 2016, 36(6): 1936-1940.
[33] Comerón A. Muñoz-porcar C, Rocadenbosch F, et al. Current research in lidar technology used for the remote sensing of atmospheric aerosols[J]. Sensors, 2017, 17(6): 1450.
[34] Liu S, Luo Z, Tan Z, et al. Gas trace detection with cavity enhanced absorption spectroscopy: a review of its process in the field[J]. Proceedings of the SPIE, 2016, 10030: 100301C.
[35] Amiot C, Aalto A, Ryczkowski P, et al. Cavity enhanced absorption spectroscopy in the mid-infrared using a supercontinuum source[J]. Applied Physics Letters, 2017, 111(6): 061103.
[36] 胡仁志, 王丹, 谢品华, 等. 二极管激光腔衰荡光谱技术测量大气NO2[J]. 光学学报, 2016, 36(2): 0230006.
[37] Banik G D, Som S, Maity A, et al. An EC-QCL based N2O sensor at 5.2 μm using cavity ring-down spectroscopy for environmental applications[J]. Analytical Methods, 2017, 9(15): 2315-2320.
[38] 姚路, 刘文清, 刘建国, 等. 基于TDLAS的长光程环境大气痕量CO监测方法研究[J]. 中国激光, 2015, 42(2): 0215003.
[39] Sur R, Sun K, Jeffries J B. et al. Scanned-wavelength-modulation-spectroscopy sensor for CO, CO2, CH4, and H2O in a high-pressure engineering-scale transport-reactor coal gasifier[J]. Fuel, 2015, 150: 102-111.
[40] Xia H, Kan R, Xu Z. et al. Measurements of axisymmetric temperature and H2O concentration distributions on a circular flat flame burner based on tunable diode laser absorption tomography[J]. Proceedings of SPIF, 2016, 10156: 101560S.
[41] 张允武. 分子光谱学[M]. 合肥: 中国科技大学出版社, 1988.
Zhang YW. Molecular spectroscopy[M]. Hefei: University of Science and Technology of China Press, 1988.
[44] Jacquinet-Husson N, Scott N A, Chédin A, et al. The GEISA spectroscopic database: current and future archive for earth and planetary atmosphere studies[J]. Journal of Quantitative Spectroscopy and Radiative Transfer, 2008, 109(6): 1043-1059.
[45] Mondelain D, Chelin P, Valentin A, et al. Line profile study by diode laser spectroscopy in the 12CH4ν2+ν4 band [J]. Journal of Molecular Spectroscopy, 2005, 233(1): 23-31.
[46] 王敏锐, 蔡廷栋. 1.5 μm处CO2与CO高温线强的实验分析与理论计算[J]. 物理学报, 2015, 64(21): 213301.
[47] Ding Y, Macko P, Romanini D, et al. High sensitivity CW-cavity ringdown and Fourier transform absorption spectroscopies of 13CO2[J]. Journal of Molecular Spectroscopy, 2004, 226(2): 146-160.
[49] Pogány A, Klein A, Ebert V. Measurement of water vapor line strengths in the 1.4-2.7 μm range by tunable diode laser absorption spectroscopy[J]. Journal of Quantitative Spectroscopy and Radiative Transfer, 2015, 165: 108-122.
[50] 聂伟, 阚瑞峰, 许振宇, 等. 6611-6618 cm -1之间氨气光谱线强的测量 [J]. 物理学报, 2017, 66(5): 054207.
[51] 聂伟, 阚瑞峰, 许振宇, 等. 基于TDLAS技术的水汽低温吸收光谱参数测量[J]. 物理学报, 2017, 66(20): 204204.
[52] 王贵师, 蔡廷栋, 汪磊, 等. 基TDLAS技术1.65 μm 附近乙烷分子高分辨吸收光谱测量[J]. 大气与环境光学学报, 2009, 4(1): 38-45.
[53] Leleux DP. Novel laser-based gas sensors for trace gas detection in a spacecraft habitat[D]. Houston: Rice University, 2002.
[54] Humlícek J. An efficient method for evaluation of the complex probability function: The Voigt function and its derivatives[J]. Journal of Quantitative Spectroscopy and Radiative Transfer, 1979, 21(4): 309-313.
[55] Ngo N H, Lisak D, Tran H, et al. An isolated line-shape model to go beyond the Voigt profile in spectroscopic databases and radiative transfer codes[J]. Journal of Quantitative Spectroscopy and Radiative Transfer, 2013, 129(11): 89-100.
[56] Wehr R, Ciuryło R, Vitcu A, et al. Dicke-narrowed spectral line shapes of CO in Ar: experimental results and a revised interpretation[J]. Journal of Molecular Spectroscopy, 2006, 237(1): 54-68.
[59] Goldenstein C S, Hanson R K. Diode-laser measurements of linestrength and temperature-dependent lineshape parameters for H2O transitions near 1.4 μm using Voigt, Rautian, Galatry, and speed-dependent Voigt profiles[J]. Journal of Quantitative Spectroscopy and Radiative Transfer, 2015, 152: 127-139.
[60] Goldenstein C S, Jeffries J B, Hanson R K. Diode laser measurements of linestrength and temperature-dependent lineshape parameters of H2O-, CO2-, and N2- perturbed H2O transitions near 2474 and 2482 nm[J]. Journal of Quantitative Spectroscopy and Radiative Transfer, 2013, 130(11): 100-111.
[62] Weldon V, Mcinerney D, Phelan R, et al. Characteristics of several NIR tunable diode lasers for spectroscopic based gas sensing: a comparison[J]. Spectrochimica Acta Part A: Molecular and Biomolecular Spectroscopy, 2006, 63(5): 1013-1020.
[63] PosthumusJ, DeningerA, LisonF. Spectral properties and spectroscopic applications of near-IR DFB diode lasers[C]∥5 th International Conference on Tunable Diodelaser Spectroscopy, July 11-15, 2005, Florence, Italy. New York: IEEE , 2005: 208.
[65] Chen F, James S, Tatam R. A 1.65 μm region external cavity laser diode using an InP gain chip and a fibre Bragg grating[J]. Proceedings of SPIE, 2012, 8421: 84215F.
[69] Shau, R, Ortsiefer, M, Bohm, G, et al. InP-based vertical-cavity surface-emitting lasers for 1.5-1.8 μm wavelength range[C]∥IEEE 17 th International Conference on Semiconductor Laser , 2000: 153- 154.
[70] Hofmann W, Amann M C. Long-wavelength vertical-cavity surface-emitting lasers for high-speed applications and gas sensing[J]. IET Optoelectronics, 2008, 2(3): 134-142.
[73] Weldon V. O' Gorman J, Pérez-Camacho J J, et al. Laser diode based oxygen sensing: A comparison of VCSEL and DFB laser diodes emitting in the 762 nm region[J]. Infrared Physics & Technology, 1997, 38(6): 325-329.
[74] WeheS, SonnenfrohD, AllenM, et al. Measurements of trace pollutants in combustion flows using room-temperature, mid-IR quantum cascade lasers[C]∥40 th AIAA Aerospace Sciences Meeting and Exhibit, January 14-17, 2002, Reno, Nevada. USA, American Institute of Aeronautics andAstronautics.c2002: 0824.
[77] Yu Y J, Sanchez N P, Griffin R J, et al. CW EC-QCL-based sensor for simultaneous detection of H2O, HDO, N2O and CH4 using multi-pass absorption spectroscopy[J]. Optics Express, 2016, 24(10): 10391-10401.
[78] Cao Y C, Sanchez N P, Jiang W Z, et al. Simultaneous atmospheric nitrous oxide, methane and water vapor detection with a single continuous wave quantum cascade laser[J]. Optics Express, 2015, 23(3): 2121-2132.
[82] 曾利忠, 陆亦怀, 阚瑞峰, 等. 高稳定低温漂半导体激光器驱动的电子学设计[J]. 量子电子学报, 2014, 31(5): 569-575.
[83] 袁松, 阚瑞峰, 何亚柏, 等. 可调谐半导体激光吸收光谱中激光器温度补偿[J]. 中国激光, 2013, 40(5): 0515002.
[85] YangC, LiuJ, HuM, et al. Multi-QCLs based open-path sensor for atmospheric NO, NO2 and NH3detections[C]∥Optics and Photonics forEnergy and theEnvironment2016, November 14-17, 2016, Leipzig, Germany. Fourier Transform Spectroscopy,c2016, JW4A. 26.
[87] Zhou X, Liu X, Jeffries J B, et al. Development of a sensor for temperature and water concentration in combustion gases using a single tunable diode laser[J]. Measurement Science and Technology, 2003, 14(8): 1459-1468.
[88] Southwest SciencesIncorporated. Gas Sensing Tutorial[EB/OL]. [2018-04-02].http:∥www.swsciences.com/technology/sensors.html.
[89] Fuchs H, Dubé W P, Lerner B M, et al. A sensitive and versatile detector for atmospheric NO2 and NOx based on blue diode laser cavity ring-down spectroscopy[J]. Environmental Science & Technology, 2009, 43(20): 7831-7836.
[90] David S. Bomse. Christian Hovde, et al. Early fire sensing using near-IR diode laser spectroscopy[J]. Proceedings of the SPIE, 2002, 4817: 73-81.
[92] Frish M B, Green B D, Allen M G. Progress in reducing size and cost of trace gas analyzers based on tunable diode laser absorption spectroscopy[J]. Proceedings of SPIE, 2004, 5586: 76-82.
[93] 王健, 黄伟, 顾海涛, 等. 可调谐二极管激光吸收光谱法测量气体温度[J]. 光学学报, 2007, 27(9): 1639-1642.
[94] 陶波, 胡志云, 张振荣, 等. TDLAS技术测量高温瞬态流场温度[ C]∥第十四届全国激波与激波管学术会议论文集(下册), 2010年7月14号, 安徽黄山. 北京: 中国力学学会, 2010: 30- 33
TaoB, Hu ZY, Zhang ZR, et al. Measurement of temperature of high temperature transient flow field by TDLAS technique[ C]∥National Symposium on Shock and Shock Tube(volume 2), July 14, 2010, Huangshan, Anhui. Beijing: Chinese Society of Theoretical and Applied Mechanics, 2010: 30- 33.
[95] Zhang G L, Liu J G, Kan R F, et al. Simulation studies of multi-line line-of-sight tunable-diode-laser absorption spectroscopy performance in measuring temperature probability distribution function[J]. Chinese Physics B, 2014, 23(12): 209-214.
[96] 许振宇, 刘文清, 刘建国, 等. 基于可调谐半导体激光器吸收光谱的温度测量方法研究[J]. 物理学报, 2012, 61(23): 234204.
[97] 陈玖英, 刘建国, 何俊峰, 等. 基于吸收光谱原理的高温水汽浓度测量方法研究[J]. 光谱学与光谱分析, 2014, 34(12): 3174-3177.
[98] Chen J Y, Li C R, Zhou M, et al. Measurement of CO2, concentration at high-temperature based on tunable diode laser absorption spectroscopy[J]. Infrared Physics & Technology, 2017, 80: 131-137.
[100] LiuJ, RuanJ, YaoL, et al. Tunable diode laser absorption based velocity sensor for local field in hypersonic flows[C]∥Proceedings of Optics and Photonics forEnergy and the Environment. November 14-17, 2016, Leipzig, Germany. Light, Energy and the Environment Congress,c2016:ETu2A. 4.
[101] 贾良权, 刘文清, 阚瑞峰, 等. 波长调制-TDLAS技术测量风洞中氧气流速方法研究[J]. 中国激光, 2015, 42(7): 0715001.
[102] Wei W, Chang J, Huang Q J, et al. Water vapor concentration measurements using TDALS with wavelength modulation spectroscopy at varying pressures[J]. Sensor Review, 2017, 37(2): 172-179.
[104] D'Amato F. Mazzinghi P, Castagnoli F. Methane analyzer based on TDL's for measurements in the lower stratosphere: design and laboratory tests[J]. Applied Physics B: Lasers and optics, 2002, 75(2/3): 195-202.
[105] Parvitte B, Zéninari V, Thiébeaux C, et al. Infrared laser heterodyne systems[J]. Spectrochimica Acta Part A: Molecular and Biomolecular Spectroscopy, 2004, 60(5): 1193-1213.
[106] Sonnenfroh D M, Rawlins W T, Allen M G, et al. Application of balanced detection to absorption measurements of trace gases with room-temperature, quasi-CW quantum-cascade lasers[J]. Applied Optics, 2001, 40(6): 812-820.
[107] 何俊峰, 阚瑞峰, 许振宇, 等. 可调谐二极管激光吸收光谱氧气测量中的导数光谱处理与浓度反演算法研究[J]. 光学学报, 2014, 34(4): 0430003.
[108] Green G L. O' Haver T C. Derivative luminescence spectrometry[J]. Analytical Chemistry, 1974, 46(14): 2191-2196.
[110] Wei M, Kan R F, Chen B, et al. Calibration-free wavelength modulation spectroscopy for gas concentration measurements using a quantum cascade laser[J]. Applied Physics B, 2017, 123(5): 149.
[111] 屈东胜, 洪延姬, 王广宇, 等. 基于免标定波长调制光谱技术的气体温度和组分浓度测量[J]. 光学学报, 2013, 33(12): 1230001.
[112] 潘虎, 王广宇, 宋俊玲, 等. 免标定波长调制光谱在气体温度和浓度测量中的应用[J]. 红外与激光工程, 2014, 43(3): 956-960.
[113] 姚路, 刘文清, 刘建国, 等. 球载TDLAS的对流层CO2浓度廓线探测[J]. 光谱学与光谱分析, 2015, 35(10): 2787-2791.
[115] Chen X, Yang C G, Hu M, et al. Highly-sensitive NO, NO2 and NH3 measurements with an open-multipass cell based on mid-infrared wavelength modulation spectroscopy[J]. Chinese Physics B, 2018, 27(4): 040701.
[116] WangL, ZhangY, HeY, et al. A laser diode sensor for in-situ monitoring of H2S in the desulfurizing device[C]∥Proceedings of 2011 International Conference on Electronics and Optoelectronics, 29-31 July, 2011, Dalian, China, New York: IEEE, 2011, V4: 276- 279.
[120] Wang A J, Yu D, Ye H, et al. Applications of optical measurement technology in pollution gas monitoring at thermal power plants[J]. Proceedings of SPIE, 2011, 8197: 819702.
[121] Wang Y, Wei Y B, Chang J, et al. Tunable diode laser absorption spectroscopy (TDLAS)-based detection of propane for explosion early warning by using a vertical cavity surface enhanced laser (VCSEL) source and principle component analysis (PCA) approach[J]. IEEE Sensors Journal, 2017, 15(17): 4975-4982.
[123] Cui X, Xu Y, Zhang X, et al. In-situ deposited flower-like Bi2MoO6, microspheres thin film based sensors for highly selective detection of ppb-level H2S at low temperature[J]. Sensors & Actuators B: Chemical, 2017, 247: 681-690.
[124] ZhangZ, ZouD, ChenW, et al. Online monitoring of escaped ammonia based on TDLAS[C]∥Proceedings of Nanophotonics, Nanoelectronics and Nanosensor, May 25-26, 2013, Wuhan, China. c2013:NSa3A. 22.
[126] Zakrzewska B. Very sensitive optical system with the concentration and decomposition unit for explosive trace detection[J]. Metrology & Measurement Systems, 2015, 22(1): 101-110.
[127] 杜振辉, 张哲远, 甄卫萌, 等. 基于中红外DFB-ICL激光器的硫系恶臭气体传感器[J]. 大气与环境光学学报, 2015, 10(2): 165-173.
[128] Pisano J T, Sauer C, Durbin T, et al. Measurement of low concentration NH3 in diesel exhaust using tunable diode laser adsorption spectroscopy (TDLAS)[J]. Sea Technical Papers, 2009: 1-10.
[129] 王飞, 黄群星, 李宁, 等. 利用可调谐半导体激光光谱技术对含尘气体中NH3的测量[J]. 物理学报, 2007, 56(7): 3867-3872.
[130] Schlosser E, Fernholz T, Teichert H, et al. In situ detection of potassium atoms in high-temperature coal-combustion systems using near-infrared-diode lasers[J]. Spectrochimica Acta Part A: Molecular and Biomolecular Spectroscopy, 2002, 58(11): 2347-2359.
[131] Qu Z, Steinvall E, Ghorbani R, et al. Tunable diode laser atomic absorption spectroscopy for detection of potassium under optically thick conditions[J]. Analytical Chemistry, 2016, 88(7): 3754-3760.
[132] 韩小磊. 基于TDLAS的天然气泄漏检测技术研究[D]. 青岛: 中国石油大学, 2009.
Han XL. The study of technique for natural gas leak detection based on TDLAS[D]. Qingdao: China University of Petroleum ( East China), 2009.
[133] Jenkins TP, Bergmans JL, Debarber PA, et al. In situ measurements of temperature in a coal-fired power plant using tunable diode laser absorption spectroscopy[C]∥American Flame Research Committee/Japanese Flame Research Committee2004Joint International Symposium, October 10-13, 2004, Maui.
[134] WalterM, SchäfersW, MarkusM, et al. Analysis and control of combustion in a waste combustion plant by means of tunable diode lasers[C]∥5th International Symposium on Gas Analysis by Tunable Diode Lasers, VDI Verlag, Düsseldorf, Germany, 1998.
[135] Ebert V, Fernholz T, Giesemann C, et al. Simultaneous diode-laser-based in situ, detection of multiple species and temperature in a gas-fired power plant[J]. Proceedings of the Combustion Institute, 2000, 28(1): 423-430.
[136] Hirmke J, Hempel F, Stancu G D, et al. Gas-phase characterization in diamond hot-filament CVD by infrared tunable diode laser absorption spectroscopy[J]. Vacuum, 2006, 80(9): 967-976.
[137] Chou S I, Baer D S, Hanson R K, et al. HBr concentration and temperature measurements in a plasma etch reactor using diode laser absorption spectroscopy[J]. Journal of Vacuum Science and Technology, 2001, 19(2): 477-484.
[138] 张帅, 刘文清, 张玉钧, 等. 基于激光吸收光谱技术天然气管道泄漏定量遥测方法的研究[J]. 物理学报, 2012, 61(5): 050701.
[141] Zhang Z R, Pang T, Yang Y, et al. Development of a tunable diode laser absorption sensor for online monitoring of industrial gas total emissions based on optical scintillation cross-correlation technique[J]. Optics Express, 2016, 24(10): A943.
[142] 耿辉, 张玉钧, 刘文清, 等. 酒精蒸汽近红外高分辨光谱获取方法研究[J]. 大气与环境光学学报, 2012, 7(1): 57-62.
[143] 汤媛媛, 刘文清, 阚瑞峰, 等. 基于量子级联激光器(QCL)高灵敏在线检测机动车尾气排放气体浓度[ C]∥中国光学学会2011年学术大会摘要集. 北京: 中国光学学会, 2011: 1.
Tang YY, Liu WQ, Kan RF, et al. High sensitivity online detection of vehicle exhaust gas concentration based on quantum cascade laser (QCL)[ C]∥Summary of the 2011 academic conference of the Chinese Academy of Optics. Beijing: Chinese Academy of Optics, 2011: 1.
[147] Azhar M, Mandon J, Neerincx A H, et al. A widely tunable, near-infrared laser-based trace gas sensor for hydrogen cyanide (HCN) detection in exhaled breath[J]. Applied Physics B, 2017, 123(11): 268.
[149] Marchenko D, Neerincx A H, Mandon J, et al. A compact laser-based spectrometer for detection of C2H2, in exhaled breath and HCN in vitro[J]. Applied Physics B, 2015, 118(2): 275-280.
[150] 姜琛昱, 孙美秀, 李迎新, 等. 激光光谱技术在呼吸气体分析中的发展与未来[J]. 中国激光, 2018, 45(2): 0207015.
[152] Wang Y, Nikodem M, Zhang E, et al. Shot-noiselimited faraday rotation spectroscopy for detection of nitric oxide isotopes in breath, urine, and blood[J]. Scientific Reports, 2015, 5(1): 9096.
[153] 方银霞, 黎明碧, 初凤友. 海底天然气水合物中甲烷逸出对全球气候的影响[J]. 地球物理学进展, 2004, 19(2): 286-290.
[155] Sommer S, Schmidt M, Linke P. Continuous inline mapping of a dissolved methane plume at a blowout site in the Central North Sea UK using a membrane inlet mass spectrometer: water column stratification impedes immediate methane release into the atmosphere[J]. Marine and Petroleum Geology, 2015, 68: 766-775.
[158] Boulart C, Connelly D P, Mowlem M C. Sensors and technologies for in situ dissolved methane measurements and their evaluation using technology readiness levels[J]. TrAC Trends in Analytical Chemistry, 2010, 29(2): 186-195.
[159] Wankel S D, Huang Y W, Gupta M, et al. Characterizing thedistribution of methane sources and cycling in the deep sea via in situ stable isotope analysis[J]. Environmental Science and Technology, 2013, 47(3): 1478-1486.
[161] Gonzalez-Valencia R, Magana-Rodriguez F, Gerardo-Nieto O, et al. In situ measurement of dissolved methane and carbon dioxide in freshwater ecosystems by off-axis integrated cavity output spectroscopy[J]. Environmental Science and Technology, 2014, 48(19): 11421.
[162] 王振南. 激光腔衰荡光谱用于水中溶存气体探测的可行性实验研究[D]. 青岛: 中国海洋大学, 2014.
Wang ZN. Development and feasibility investigation of cavity ringdown systems for trace dissolved gas detection[D]. Qingdao: Ocean University of China, 2014.
[163] Grilli R, Marrocco N, Desbois T, et al. SUBGLACIOR: an optical analyzer embedded in an Antarctic ice probe for exploring the past climate[J]. Review of Scientific Instruments, 2014, 85(11): 111301.
[164] Kasyutich V L, Martin P A. Towards a two-dimensional concentration and temperature laser absorption tomography sensor system[J]. Applied Physics B, 2010, 102(1): 149-162.
[165] BrownM, HerringG, CabellK, et al. Optical measurements at the combustor exit of the HIFiRE 2 ground test engine[C]∥AIAA Aerospace Sciences Meeting Including the New Horizons Forum and Aerospace Exposition Jan. 9-12, 2012, Nashville, Tennessee. AIAA, 2012: 210- 231.
[167] 庄天戈. CT原理与算法[M]. 上海: 上海交通大学出版社, 1992.
Zhang TG. CT principle and algorithm[M]. Shanghai: Shanghai Jiao Tong University Press, 1992.
[172] Song J L, Hong Y J, Wang G Y, et al. Algebraic tomographic reconstruction of two-dimensional gas temperature based on tunable diode laser absorption spectroscopy[J]. Applied Physics B, 2013, 112(4): 529-537.
[174] Hanson RK. High-resolution spectroscopy of shock-heated gases using a tunable infrared diode laser[C]∥Proceedings of the Eleventh International Symposium, Seattle, Wash., July 11-14, 1977.Seattle: University of Washington Press, 1978: 432- 438.
[176] JacksonK, GruberM, Barhorst T. The HIFiRE flight 2 experiment: an overview and status update[C]∥45th AIAA/ASME/SAE/ASEE Joint Propulsion Conference & Exhibit. August 02-05, 2009, Denver, Colorado USA. American Institute of Aeronautics and Astronautics, c2009: 5029.
[177] Lin X, Yu X L, Li F, et al. CO concentration and temperature measurements in a shock tube for Martian mixtures by coupling OES and TDLAS[J]. Applied Physics B, 2012, 110(3): 401-409.
[178] Bain, James RP. Near infrared tunable diode laser spectroscopy for aero engine related applications[D]. Britain: University of Strathclyde, 2012.
[180] WeheS, BaerD, HansonR, et al. Tunable diode-laser absorption measurements of temperature, velocity, and H2O in hypervelocity flows[C]∥Proceedings of the 33rd AIAA/ASME/SAE/ASEE Joint Propulsion Conference & Exhibit, July 6-9, 1997, Seattle, WA, USA. AIAA, 1997: 3267.
[181] Xia H H, Kan R F, Xu Z Y, et al. Measurements of axisymmetric temperature and H2O concentration distributions on a circular flat flame burner based on tunable diode laser absorption tomography[J]. Proceedings of SPIE, 2016, 10156: 101560S.
[182] Drake MC, Fansler TD, Solomon AS, et al. Piston fuel films as a source of smoke and hydrocarbon emissions from a wall-controlled spark-ignited direct-injection engine[M]. Warrendale, PA, USA: SAE International, 2003.
[183] HentschelW, GroteA, LangerO. Measurement of wall film thickness in the intake manifold of a standard production SI engine by a spectroscopic technique[M]. Warrendale, PA, USA: SAE International, 1997.
[185] 杨荟楠, 郭晓龙, 苏明旭, 等. 基于TDLAS技术在线测量气流道内液膜动态厚度[J]. 中国激光, 2014, 41(12): 1208010.
[186] Yang H, Greszik D, Wlokas I, et al. Tunable diode laser absorption sensor for the simultaneous measurement of water film thickness, liquid- and vapor-phase temperature[J]. Applied Physics B, 2011, 104(1): 21-27.
[187] Yang H N, Guo X L, Zhou W, et al. Investigation on liquid film of urea-water solutions with diode laser absorption spectroscopy[J]. Experiments in Fluids, 2015, 56(4): 1-7.
[188] Pan R, Jeffries J B, Dreier T, et al. Measurements of liquid film thickness, concentration, and temperature of aqueous urea solution by NIR absorption spectroscopy[J]. Applied Physics B, 2016, 122(1): 4.
[189] Water Structure and Science. Liquid water is not a bit player in the theatre of life—it's the headline act[EB/OL].[2018-04-02]. http: ∥www1.lsbu.ac.uk/water/water_structure_science.html.
[190] Maréchal Y. The molecular structure of liquid water delivered by absorption spectroscopy in the whole IR region completed with thermodynamics data[J]. Journal of Molecular Structure, 2011, 1004(1/2/3): 146-155.
[191] Hale G M, Querry M R. Optical constants of water in the 200-nm to 200-μm wavelength region[J]. Applied Optics, 1973, 12(3): 555-563.
[194] Porter J M, Jeffries J B, Hanson R K. Mid-infrared laser-absorption diagnostic for vapor-phase fuel mole fraction and liquid fuel film thickness[J]. Applied Physics B, 2010, 102(2): 345-355.
Article Outline
聂伟, 阚瑞峰, 杨晨光, 陈兵, 许振宇, 刘文清. 可调谐二极管激光吸收光谱技术的应用研究进展[J]. 中国激光, 2018, 45(9): 0911001. Nie Wei, Kan Ruifeng, Yang Chenguang, Chen Bing, Xu Zhenyu, Liu Wenqing. Research Progress on the Application of Tunable Diode Laser Absorption Spectroscopy[J]. Chinese Journal of Lasers, 2018, 45(9): 0911001.