Broadband spectrum generation with compact Yb-doped fiber laser by intra-cavity cascaded Raman scattering
Download: 1065次
The nonlinear polarization rotation (NPR) technique has become one of the main techniques to achieve mode-locked fiber lasers for its compactness, implementation, and low cost[1]. NPR mode-locked fiber lasers operating in the all normal dispersion (ANDi) regime[2] have been widely investigated for their high pulse energy[3–
Broadband spectrum generation at 1.0–1.2 μm has gained much attention for applications in gas sensing, optical communication systems, and wavelength tunable and optical coherence tomography (OCT). An alternative way to generate a broadband spectrum is by using noise-like pulse (NLP) emission in an optical fiber ring cavity. Reference [13] reported that the NLP with a spectrum bandwidth of up to 131 nm (FWHM) was obtained in a YDF laser. Raman scattering generated broadband spectrum in optical fiber lasers has also been widely investigated[14–
In this Letter, there are no physical filters and polarization controllers in the laser cavity. A mode-locked pulse train is achieved in the ANDi regime based on the NPR technique. The fiber birefringence-induced filtering effect (FBFE) is the main reason for mode locking in our laser setup. The extra 20 m long single mode fiber (SMF) is inserted in two different positions, where the DS operation and NLP operation are achieved correspondingly. The nonlinear effect is obviously enhanced in the NLP regime, and broadband spectrum generation is owed to the enhanced SRS progress. The maximum output average power and peak power are 14.75 nJ and 18.0 W, so the laser is an ideal source for OCT.
The experimental setup of a simplified Yb-doped mode-locked fiber laser is shown in Fig.
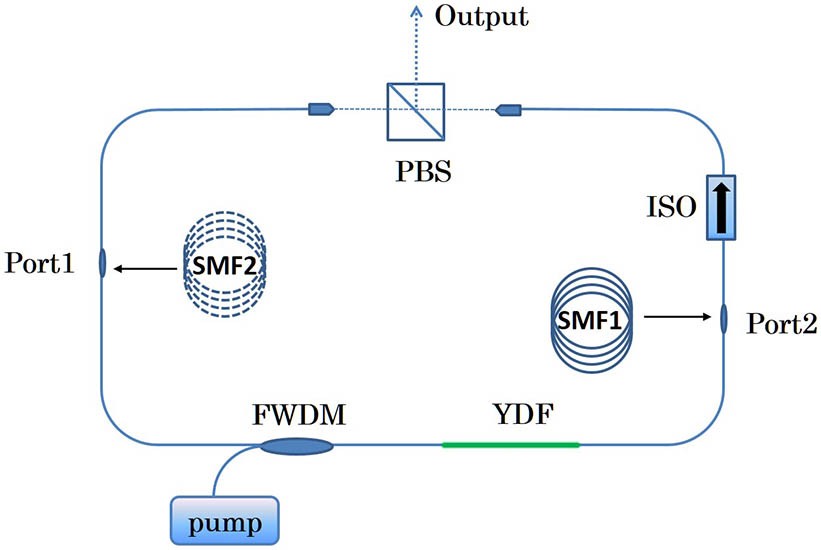
Fig. 1. Setup of the simplified Yb-doped mode-locked fiber laser. Port 1, the extra 20 m SMF (dotted line) located behind the output port; Port 2, the extra 20 m SMF (solid line) located before the output port.
First, we placed an extra SMF (

Fig. 2. (Color online) Characteristics of mode-locked operation. (a) The pulse shape and the pulse train (the insert) at pump power of 210 mW, and (b) the spectra under different pump powers.
Owing to the laser operated in the ANDi regime, the continuous pulse train formation should balance the cavity dispersion, fiber nonlinearity, gain, and loss. Different from the anomalous dispersion regime, where the pulse shaping is mainly due to the “self-balance” ability between the anomalous dispersion and fiber nonlinearity, in the ANDi regime, a bandwidth limited component is crucial for mode locking. There were no physical bandpass filters or polarization controllers in the oscillator, but mode locking is still obtained. The mechanism of the FBFE[10] can explain the intrinsic mode locking in this oscillator. The cavity birefringence is equivalent to the effective cavity transmission bandwidth. Although the YDF has a broad gain bandwidth, due to the bandpass limitation of the FBFE, the effective gain of the laser has a narrow bandwidth. We demonstrated a more simplified model utilizing FBFE for mode locking without the physical filter and polarization controller in the oscillator. Moreover, Fig.
The FBFE acts as a spectral filter in a simplified mode-locked fiber laser, and a DS was achieved[12,28]. The cavity design is applicable for the laser operating in the DS regime. The extra SMFs is long enough to accumulate the nonlinear phase delay for mode locking and obtain a large dispersion to avoid a pulse split. The placing of extra SMF behind the output is important for the pulse type, owing to the nonlinearity accumulation that is not larger than the critical saturation power (CSP) of the FBFE. If the peak power passes over the CSP, the oversaturation of the FBFE can give rise to high modal fluctuations and instabilities, thereby possibly leading to the formation of NLPs[10,19,28,29].
Secondly, we changed the position of the extra SMF and put it before the output as port 2 (solid line), as illustrated in Fig.
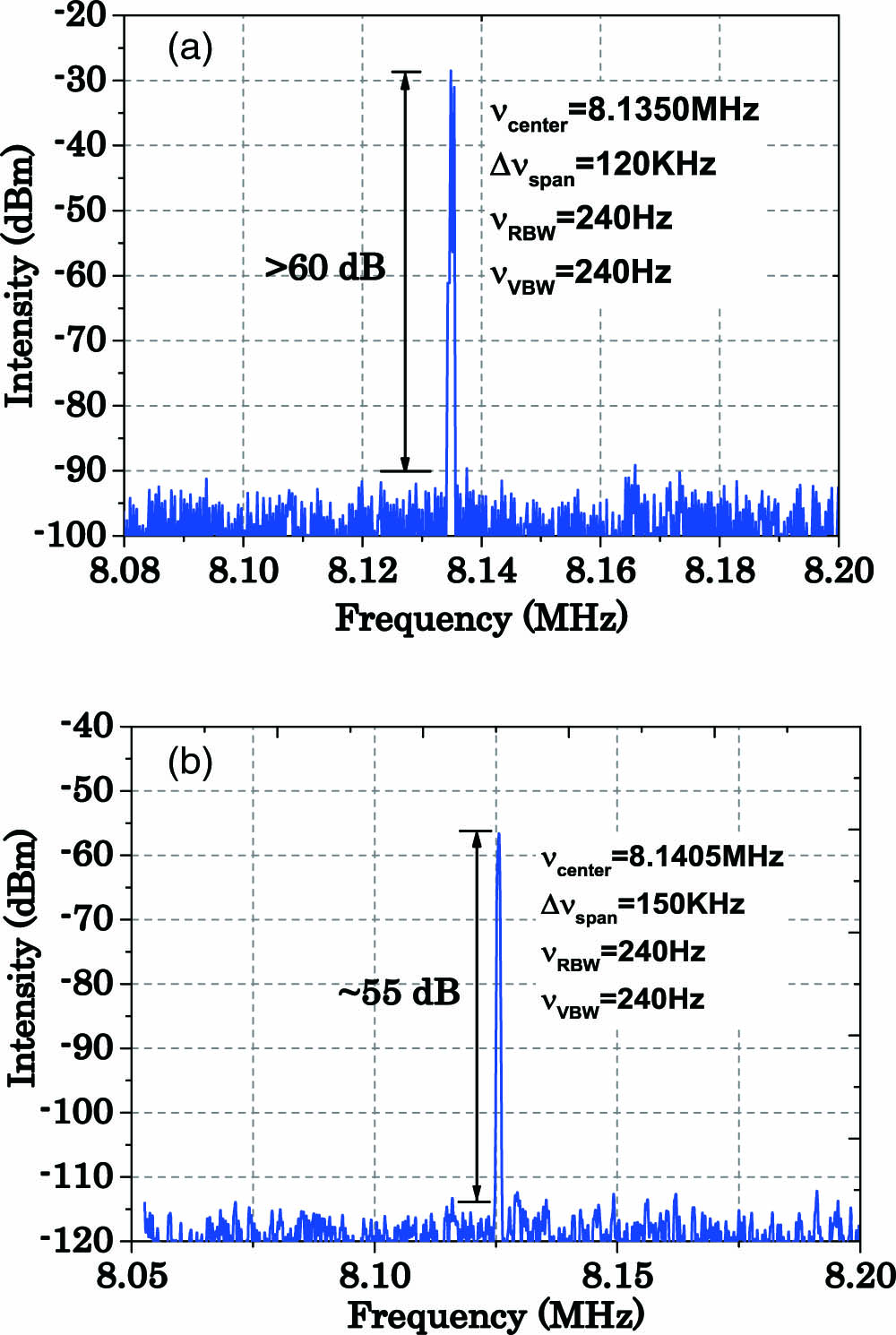
Fig. 3. RF spectrum of the different mode-locked operations. (a) The SMF2 spliced in the cavity, and (b) the SMF1 spliced in the cavity.
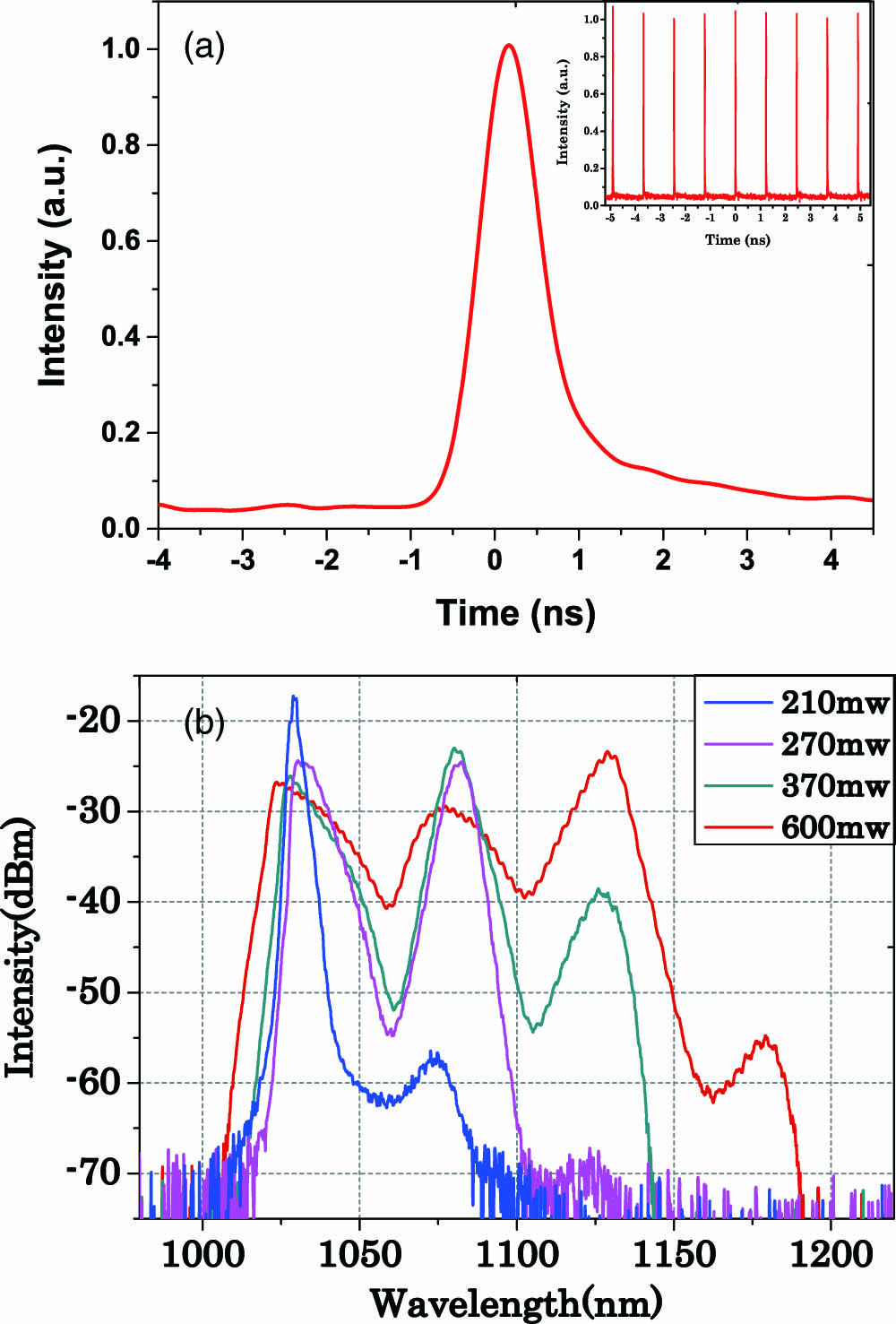
Fig. 4. (Color online) Characteristics of mode-locked operation. (a) Temporal pulse shape is measured at the pump of 370 mW and (b) spectra under different pump powers.
The evolution of spectra with different pump powers shows that the broader and smoother spectra are generated in the higher pump power. The evolution is closely relative to Raman scattering and the Kerr effect in the fiber oscillator. The result indicates that the laser may operate in the NLP regime[19,28], and the enhanced Raman scattering may collapse the DSs into NLPs[10]. Considering the laser setup, the place of the extra SMF is the only changeable parameter for our contrast experiments, so the FBFE changes a little. It is noteworthy that although the nonlinear phase delay is an influence factor of the FBFE, and the nonlinear accumulation in the oscillator is far above the disturbance of the FBFE, the place of the extra SMF in the oscillator is the main reason for the different results. For instance, once the peak power eventually reaches the CSP, any further increment in the pulse peak power confirms that the strong gain observed at the Stokes wavelength of the spectrum is due to the SRS progress. New frequency components generated and thereby disrupted the DS pulse formation in the cavity and finally triggered the NLP formation.
In conclusion, we experimentally demonstrate a simple compact fiber laser mode-locked by the FBFE in the ANDi regime based on the NPR technique and give a reason for the nonlinear dynamics of the DS operation and the NLP operation, corresponding the extra SMF location in the cavity. The intra-cavity enhanced SRS progress is a thought for special wavelength generation. The next work is ongoing for an amplifier based on the broadband spectrum oscillator.
[1]
[2]
[3]
[4]
[5]
[6]
[7]
[8]
[9]
[10]
[11]
[12]
[13]
[14]
[15]
[16]
[17]
[18]
[19]
[20]
[21]
[22]
[23]
[24]
[25]
[26]
[27]
[28]
[29]
Zikai Dong, Yanrong Song, Runqin Xu, Yu Zheng, Jinrong Tian, Kexuan Li. Broadband spectrum generation with compact Yb-doped fiber laser by intra-cavity cascaded Raman scattering[J]. Chinese Optics Letters, 2017, 15(7): 071408.