High absorption large-mode area step-index fiber for tandem-pumped high-brightness high-power lasers
Download: 760次
1. INTRODUCTION
Tandem pumping is an attractive solution in power scaling of fiber laser as it greatly eases out heat-related limits such as transverse mode instability (TMI) and helps single mode operation [1,2]. Adoption of the tandem pumping requires a long length of a gain fiber because of lower absorption cross section of rare-earth ions [such as ytterbium (Yb)] at a tandem pumping wavelength. Consequently, nonlinear scattering such as stimulated Raman scattering (SRS) becomes an ultimate limit of the tandem pumping scheme in a large-mode area (LMA) step-index fiber when the core size cannot be scaled further in the interest of beam quality control [1]. On the other hand, good beam quality in fiber lasers is achieved through preferential fundamental mode (FM) amplification in a gain fiber. The most common gain fiber is the step-index fiber, which is known for its robustness, reliability, and cost effectiveness in mass production. For a step-index fiber, the number of guided modes is determined by the V number, , where is the core diameter, NA is the numerical aperture, and is the wavelength; and the fiber supports only one mode if [3]. Most single-mode high-power lasers, however, employ LMA gain fibers, e.g., with and for better power handling [4,5]; despite at for these fibers, effective FM operation was demonstrated through bend-induced losses of the higher-order modes (HOMs) [68" target="_self" style="display: inline;">–
To power-scale single-mode lasers further to several kilowatts, the threshold for nonlinear effects has to be raised, and this is commonly attained by increasing the core diameter to reduce the signal intensity; however, increases as a result and it becomes more difficult to filter the HOMs. In addition, the most practical method to suppress the TMI is perhaps utilizing a modal discrimination bending effect [9], which is more profound in a low number. Thus, the core NA must be reduced to maintain a low number for beam quality control and power scaling. A straightforward method to reduce the core NA is through reducing the dopant concentration of Yb ions. The Yb ions have a high molar refractivity ( per mol% in [10]) compared to other common dopants in a laser fiber, and thus contribute the most to the core refractive index. Through this approach, Yb-doped fibers (YDFs) with NA as low as 0.04 have recently been demonstrated in Refs. [10,11]. However, they come at the cost of a poorer pump absorption rate (0.2 dB/m [9] and 0.5 dB/m [12] at 976 nm), which clearly illustrates the problem of a step-index design. Low NA () and high absorption ( core absorption) cannot be simultaneously satisfied [1315" target="_self" style="display: inline;">–
Here, we report a highly efficient tandem pumped fiber amplifier using a very short absorption length YDF that features a low-index fiber of core NA 0.04 with simultaneous high pump absorption rate of 27 dB/m at 976 nm. The low NA highly doped Yb core is achieved by replacing the silica cladding with a germanium (Ge)-doped cladding and subsequently reducing the NA of the core. In particular, our fiber consists of the Ge-doped cladding and an Yb-doped alumino-phosphosilicate core that was tested to exhibit excellent photodarkening (PD) suppression [27]. Our previous report presented the feasibility of this Ge cladding approach via a standard modified chemical vapor deposition (MCVD) process and solution doping method [28]. In this work, we demonstrate high-power monolithic fiber amplifier using an LMA Ge cladding Yb fiber with a very low core NA of 0.04 and a record high cladding absorption of 27 dB/m for a step-index LMA fiber. The fiber has a core with average step-index of with respect to the Ge cladding. The index fluctuations are kept within for the Ge cladding and for the Yb core—close to the tolerance commonly reported for the MCVD process [28]. The achieved low NA promotes single-mode propagation and a large FM area, while the high pump absorption rate shortens the gain fiber length; these traits enable power scaling of single-mode lasers via tandem pumping. The fiber was tandem-pumped at 1 kW 1018 nm pump power to generate 1064 nm signal power of 836 W (pump power limited) with an excellent efficiency of 82.5%. Thanks to the high absorption low NA design, the amplifier was optimized at of the YDF that delivered a near-Gaussian beam of at maximum output power. Hence, our results show greater potential for enabling multi-kW lasers [29].
2. FIBER DESIGN AND FABRICATION
To lase efficiently with near-single-mode output in a step-index LMA fiber, a simple fiber bending technique was found effective to impose differential bending loss of in HOMs and in an FM, which was determined via rate equation simulations [4,30]. As our fiber, despite the Ge cladding, has a step-index profile, these criteria should be applicable to safeguard the desired FM operation, as represented in Fig.
![Simulated bend losses of LP01 FM (blue) and LP11 HOM (red) for a 30 μm step-index fiber modeled with 2×10−4 index fluctuations (as fabrication tolerance) in the core and cladding. The solid lines are the predicted performance based on the measured RIP of the fiber reported in this work [see Fig. 2(a)].](/richHtml/prj/2020/8/10/10001599/img_001.jpg)
Fig. 1. Simulated bend losses of FM (blue) and HOM (red) for a 30 μm step-index fiber modeled with index fluctuations (as fabrication tolerance) in the core and cladding. The solid lines are the predicted performance based on the measured RIP of the fiber reported in this work [see Fig. 2 (a)].
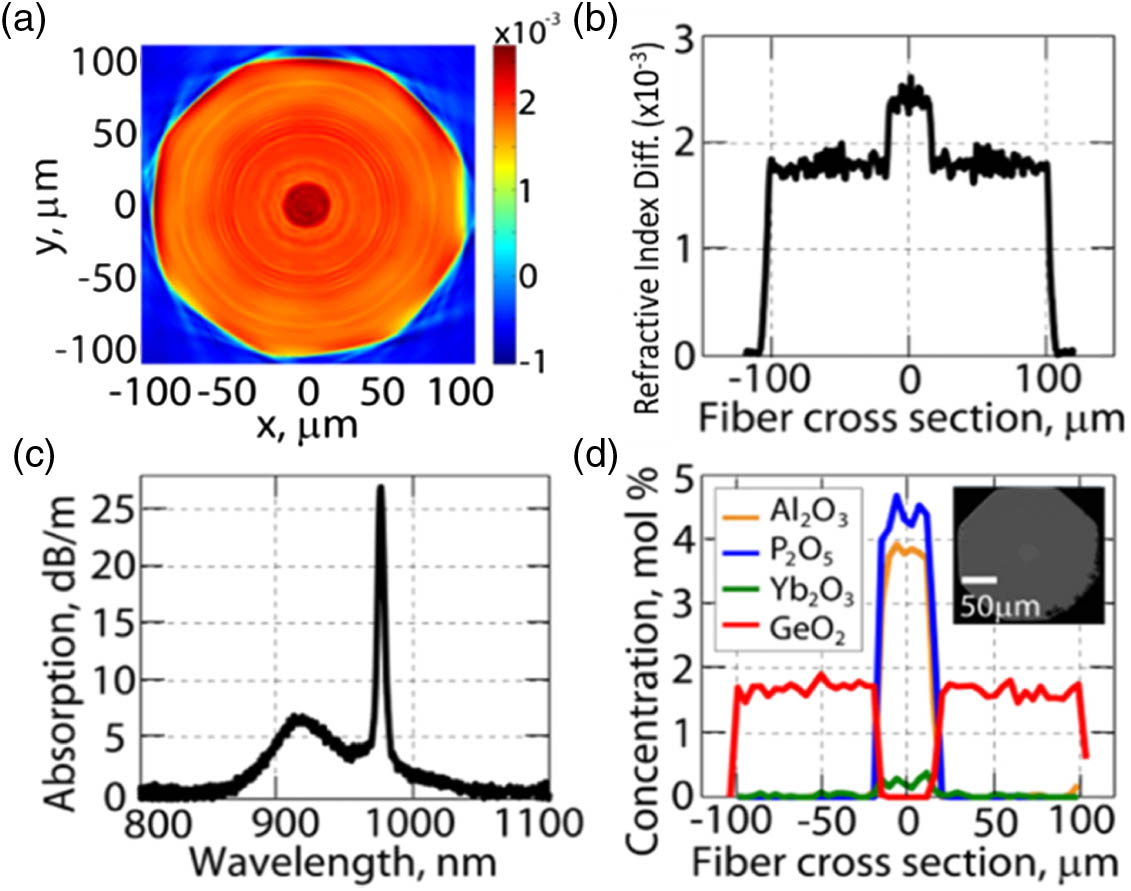
Fig. 2. Characterization results of the 30/200 low NA double-clad YDF that consists of a Ge-doped cladding and Yb/Al/P-doped cores. (a) 2D RIP, (b) 1D RIP, (c) cladding absorption, and (d) EDX dopant concentration profiles (SEM image of fiber cross section in inset).
The lower and upper bounds on the NA required to meet the good beam quality criteria were found to be 0.037 and 0.044, respectively, as shown in Fig.
The low NA YDF in this work consists of a Ge-doped cladding to raise the cladding’s refractive index by replacing a conventional silica cladding. The intent is to negate the index-raising Yb ions in the core to maintain a low NA. Using this approach, the trade-off between NA and Yb absorption is overcome to enable simultaneous low NA and high absorption. However, the required tolerances on the index fluctuations become tighter due to the smaller core index step in a fiber, making fabrication challenging. Here, the MCVD and fiber drawing processes are optimized to achieve a reproducible recipe that demonstrates the highest cladding absorption in an Yb-doped low-NA LMA fiber to date, setting the stage for tandem-pumped higher-power single-mode lasers with a simple step-index design.
The fabrication process involves first depositing the Ge-doped cladding, followed by solution doping of the Yb-doped alumino-phosphosilicate core. During the deposition of Ge-doped cladding in MCVD, the and flow ratio is optimized to yield the required refractive index for the targeted core NA of 0.04, and pressurizing is employed to deposit multiple layers in order to build sufficient thickness of the cladding. Deposition of a phosphorus-doped (P) silica soot layer is then carried out and presintered to achieve the targeted soot porosity for solution doping, where Yb and aluminum (Al) are doped into the P soot via an alcohol-based solution to form the core. The preform subsequently undergoes oxidation and collapse steps before being sealed. P overdoping is carried out to completely remove the index dip in the core.
The round preform is subsequently shaped into an octagon using an ultrasonic milling machine to obtain the required cladding-to-core size ratio while ensuring low offset in core concentricity. The octagonal shaping is required to promote mode mixing of the pump light to enhance cladding absorption. A silica cladding inherited from a substrate tube was removed during the shaping. Hence, the octagonal cladding consists purely of the deposited Ge-doped cladding. Finally, mechanical and fire polishing are carried out on the preform to produce an optically transparent surface finish before fiber draw. The draw condition is optimized to produce a YDF that is mechanically strong for handling and able to undergo standard fiber stripping, cleaving, and splicing processes just like a conventional silica cladding step-index fiber.
3. FIBER CHARACTERIZATION
The RIP of the fiber is measured with Interfiber Analysis IFA-100 fiber index profiler using index oil with a matching refractive index to a silica substrate tube. The two-dimensional (2D) refractive index representation of the YDF is given in Fig.
From Fig.
The resistance of the fiber against PD is important for its long-term stability and reliability. We conducted an accelerated PD test under core pumping as detailed in Ref. [28]. The fiber showed insignificant PD loss. We repeated the PD test in a cladding-pump configuration to confirm the PD performance. With a 15 cm sample under near 40% inversion by a 915 nm laser diode cladding pumping, the probe wavelength loss at 635 nm was nearly negligible. We attribute this good PD performance to the 15 times higher concentration of (compared to ), which is known to be effective in mitigating PD [35].
4. ALL-FIBER HIGH-POWER AMPLIFIER
To study the optical performance of the fabricated low NA YDF, we employed an all-fiber forward-pumped amplifier configuration, as shown in the top of Fig.
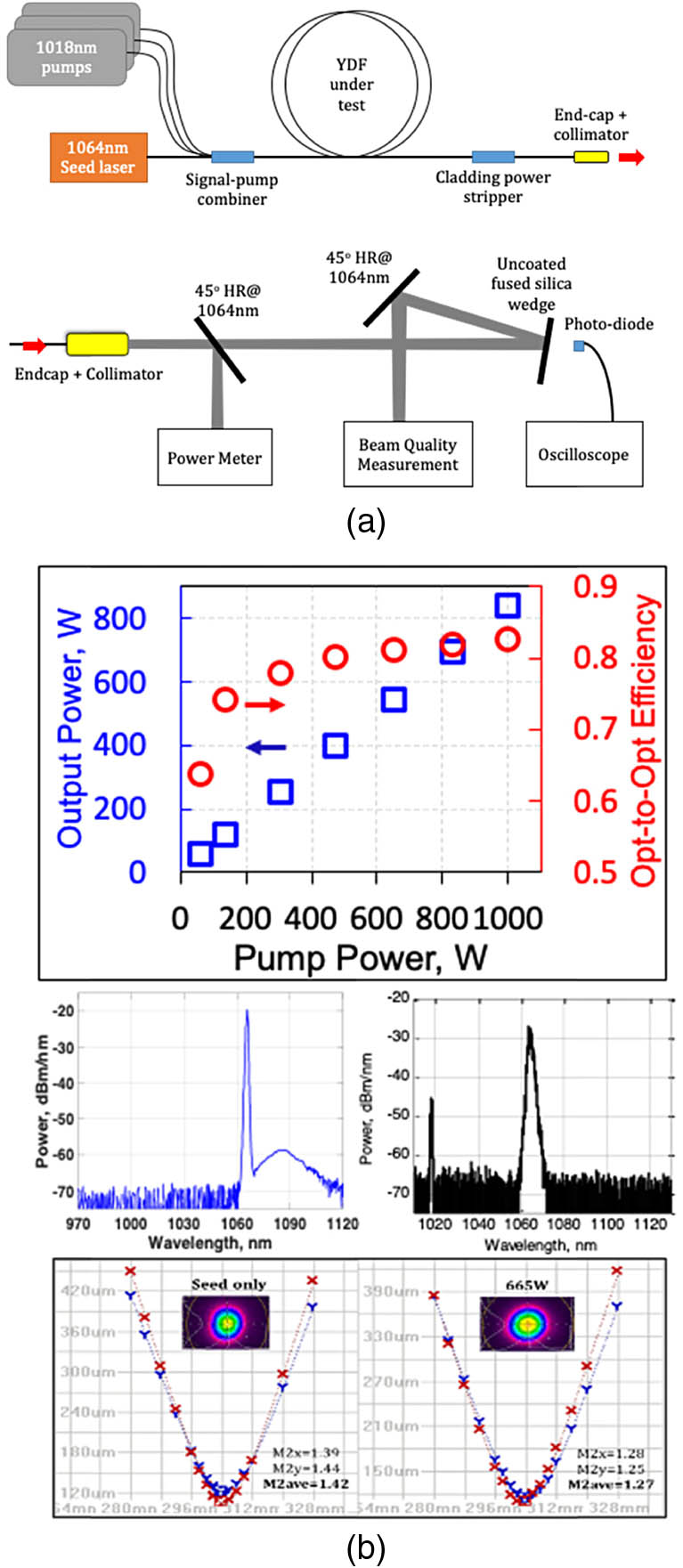
Fig. 3. (a) All-fiber tandem-pump laser setup (top) with its free-space beam diagnostics layout (bottom). The red solid arrow indicates direction of the laser output beam. (b) Optical performance of the fabricated low NA YDF. Top, the output power and efficiency of the amplified signal at 1065 nm, plotted with respect to the pump power at 1018 nm; middle, the spectra of the seed (left) and the amplified signal (right); bottom, of the laser output before (left) and after (right) amplification.
The amplifier was tested up to 1 kW of pump power to produce an output of 836 W at 1065 nm, achieving an optical-to-optical efficiency of 82.5% at maximum power and a slope efficiency of 83.0% [see the top plot of Fig.
The laser beam quality was measured with a Spiricon beam propagation analyzer; the results are shown in the bottom plot of Fig.
5. CONCLUSION
We fabricated a low NA 0.04 step-index YDF that possesses simultaneously high cladding absorption of 27 dB/m at 976 nm. This was enabled by an index-raised Ge-doped cladding to negate the index raising by the high Yb concentration, as well as low refractive index fluctuations of below across the fiber. The FM field area calculated from the measured RIP was . Furthermore, negligible PD-induced loss at 635 nm was detected over 1 h at near 40% inversion. To our knowledge, this is the highest cladding absorption attained in any low NA step-index YDF. The YDF was employed in a tandem-pump amplifier and tested up to 1 kW of pump power to produce an output of 836 W and an optical-to-optical efficiency of 82.5%, with no sign of rollover. Despite the lower absorption cross section at 1018 nm ( of 976 nm), the amplifier was constructed with a YDF length of only 13.8 m, thanks to the high absorption, and thus beneficial to nonlinearity suppression. Furthermore, because of the inherent low thermal load in a tandem pumping scheme, such a short length fiber does not raise a thermal issue. Hence, we demonstrated a power scaling route through tandem pumping for low thermal load and low nonlinear scattering. The beam quality improved under amplification to give of 1.27 enabled by the step-index design.
The laser experimental results validate the reported low NA YDF design and our fabrication processes, in addition to the YDF’s ability to handle high power and withstand thermal load. Furthermore, our low NA YDF is demonstrated in an all-fiber monolithic laser setup, which further illustrates its robustness to cleaving and splicing. These results exhibit the potential of the Ge-doped cladding YDFs to outdo the conventional trade-off between beam quality, LMA, and high cladding absorption in order to enable further power scaling in single-mode fiber lasers. Our recent demonstration of ultrafast fiber lasers using the same fiber approach [40] suggests another meaningful application enabled by the presented fiber design.
6 Acknowledgment
Acknowledgment. We acknowledge helpful discussions with Dr. Kin-Seng Lai, David Men-Seng Yue, Huizi Li, and Jian-Wei Lua.
Article Outline
Kang-Jie Lim, Samuel Kai-Wen Seah, Joash Yong’En Ye, Wendy Weiying Lim, Chu-Perng Seah, Yunn-Boon Tan, Suting Tan, Huiting Lim, Raghuraman Sidharthan, Arumugam Rajendra Prasadh, Chen-Jian Chang, Seongwoo Yoo, Song-Liang Chua. High absorption large-mode area step-index fiber for tandem-pumped high-brightness high-power lasers[J]. Photonics Research, 2020, 8(10): 10001599.