Dual-modal photoacoustic/CT imaging system
Small animals, especially mice and rats, are widely used in biomedical research. Various imaging techniques have been developed for small animal studies[1]. In comparison with conventional imaging techniques like magnetic resonance imaging (MRI), X-ray computed tomography (CT), positron emission tomography (PET), and single-photon emission computed tomography (SPECT), optical imaging has superior characteristics, including non-radioactivity, high sensitivity, and cost-effectiveness. Although tissues’ optical properties contain rich structural and functional information, the strong light scattering by tissues makes pure optical imaging methods always suffer low spatial resolution when imaging deep in live animals. Over the past decade, the emerging photoacoustic (PA) tomography (PAT) has become a very promising method for small animal imaging, including structural[2
PAT is a hybrid imaging technique that combines optical absorption contrast and ultrasonic detection[16–
In this study, we integrated PAT with an X-ray-based CT to develop a standalone system. Our results demonstrated that this dual-modality imaging system has the advantages of both PAT and CT. The dual-modal imaging system in this study contains a PAT and a micro-CT, as shown in Fig.
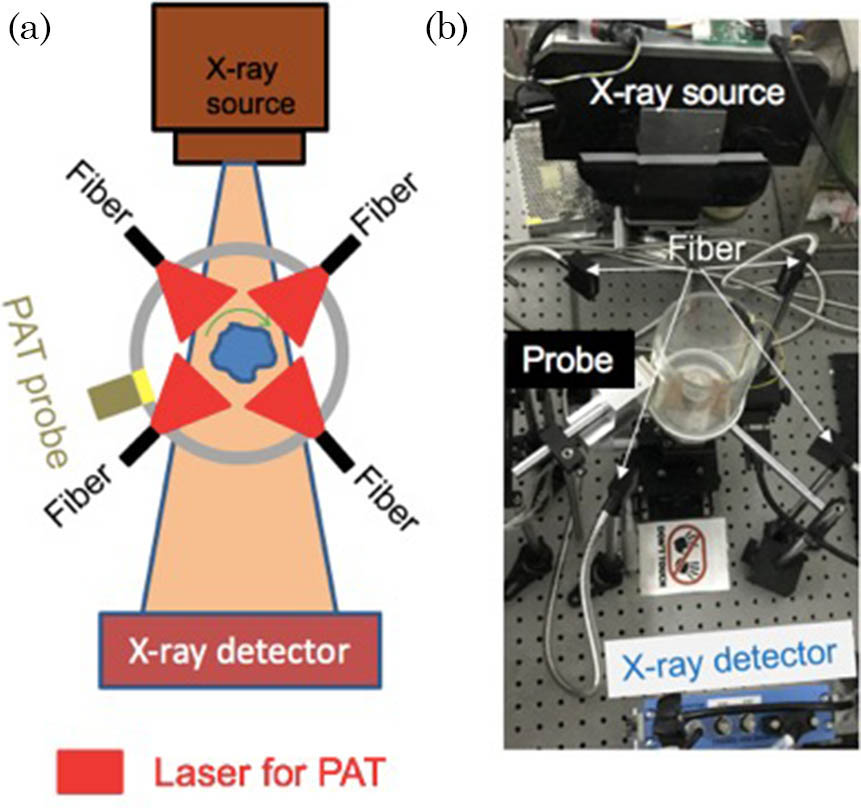
Fig. 1. PAT-CT imaging system. (a) A schematic top view of the system describing the main components. (b) A photograph of the system. The phantom is positioned in the center.
The PAT system contains a 48-element customized US linear array (by TomoWave. Inc., Houston, Texas, USA) to detect the PA signal. Each element has a size of
The micro-CT system consists of an X-ray tube (XRS-125-2-P-CW, Source-ray. Inc., USA) and a planar detector (Dexela 1512 CMOS X-ray Detector, Dexela Ltd., UK). The electrical voltage and the current of the tube were set to 80 kV and 0.5 mA, respectively. The anode is tungsten with a focal spot of 0.5 mm. To alleviate the beam-hardening artifact, a 4.5 mm thick aluminum sheet was placed in front of the X-ray tube. The X-ray detector has
We performed the imaging sequentially in two steps (the reason for this procedure will be explained later): CT imaging followed by a PAT. During the CT imaging, no water was filled in the water tank, and CT recordings were evenly acquired along 360 deg. Then we filled the water tank with deionized (DI) water and started the PAT imaging. The rotational stage moved continuously at a speed of 1.2 deg/s. The data acquisition was triggered by the laser at a repetition rate of 10 Hz. A total of 3000 PA data sets (each set stored
For PAT reconstruction, we used the image reconstruction algorithm described in Ref. [30] for a 2D cylindrical detection configuration. The CT image reconstruction was based on the Feldkamp, Davis, and Kress reconstruction method[31]. The images were reconstructed to a
Spatial calibration must be done to co-register PAT and CT images. In this study, we used a painted thin metal wire as the dual-modal contrast. After image reconstruction for both modalities, we loaded these two images into a 3D Slicer (version 4.6, slicer.org) and set the CT image of the wire in the center. Then we manually rotated and translated the corresponding PAT image to fuse them, finding the calibration parameters. Therefore, we can get the spatial rotational and translational parameters to be used for later image fusing.
The agar phantom was a cylinder made of 1% intralipid and 3% agar, and it had a diameter of 27 mm. There were three objects buried in the agar: one black carbon rod with a diameter of 0.5 mm, one helix, and one transparent glass tube, as shown in Fig.
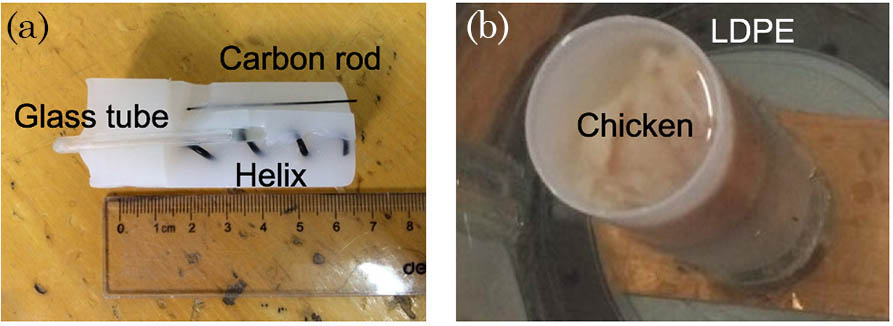
Fig. 2. Photographs of the two phantoms. (a) The agar phantom with the carbon rod, helix, and glass tube. The photo was taken after the experiment. (b) The chicken breast tissue phantom.
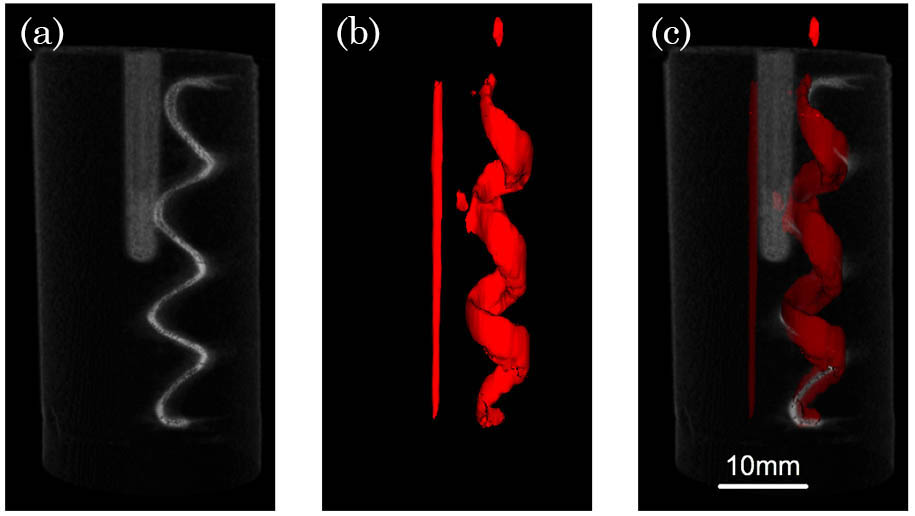
Fig. 3. Agar phantom imaging results. (a) The 3D rendering result for the CT reconstruction. (b) The 3D rendering result for the photoacoustic imaging. (c) The co-registered rendering of the PAT and CT images (Video 1 ).
To demonstrate the potential of the system for tissue study, chicken breast tissue was also used in the study. One carbon rod with a diameter of 0.5 mm, one metal wire with a diameter of 0.4 mm, and one thin glass tube were imbedded in chicken breast tissue. Part of the metal wire was painted black. We inserted the chicken tissue into a plastic bottle made of low-density polyethylene (LDPE), which has a near-acoustic impedance with water.
In order to remove air bubbles, the bottle was also filled with water, as shown in Fig.
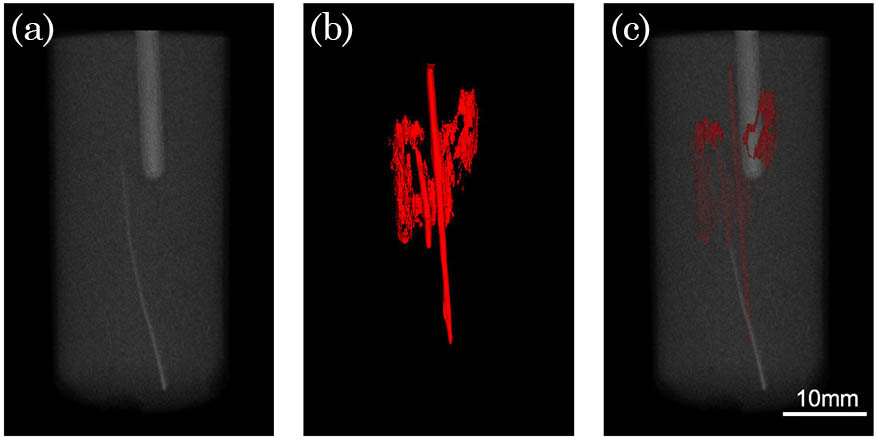
Fig. 4. Tissue phantom imaging results. (a) The 3D rendering result for the CT reconstruction. (b) The 3D rendering result for the photoacoustic reconstruction. (c) The co-registered image of the PAT and CT imaging (Video 2 ).
Then we carried out the animal experiment on a 20 g BALB/c female nude mouse. The mouse was euthanatized by
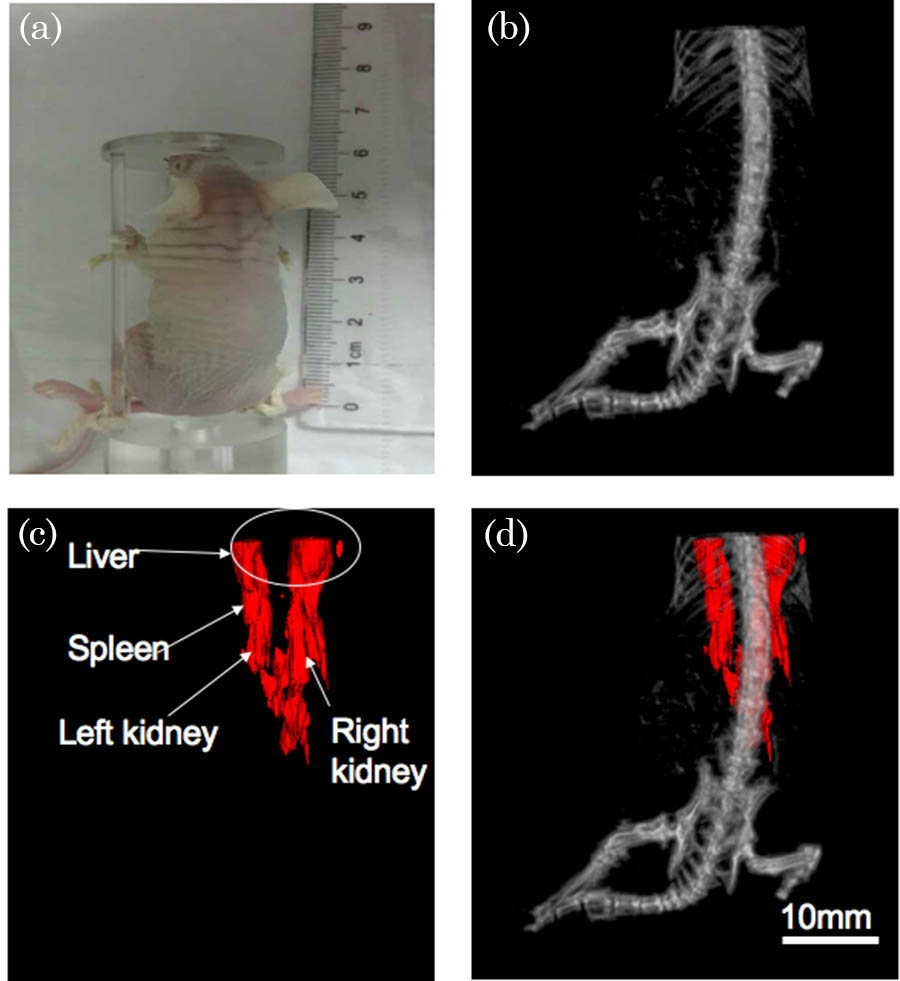
Fig. 5. Mouse imaging results. (a) The nude mouse picture that was taken before the experiment. (b) The 3D rendering result for the CT reconstruction. (c) The 3D rendering result for the photoacoustic reconstruction. (d) The co-registered image of the PAT and CT imaging (Video 3 ).
In this study, we presented a standalone dual-modal PAT-CT imaging system. PAT provides the optical absorption contrast, and CT gives the mass density distribution. The system obtains these two important complementary pieces of information with a highly accurate spatial registration. The imaging depth was demonstrated to be more than 2 cm in scattering biological tissues. The performance of this system has been demonstrated by two types of phantom studies and mouse experiments.
Because the X-ray source used in this study has a relative low power, the attenuated X-ray, after passing through the water in the tank, is too weak to be detected. Therefore, we first imaged the target by CT without filling water in the tank. A more powerful X-ray source would enable two modalities to image simultaneously in future. In addition, because it is limited by the element’s size, the geometry of the linear probe, and the bandwidth, our current PAT system has an imaging resolution of
[2] 2SongK. H.WangL. V., Med. Phys.35, 4524 (2008).MPHYA60094-240510.1118/1.2977534
[9] 9XuM.LeiP.FengJ.LiuF.YangS.ZhangP., Chin. Opt. Lett.16, 031702 (2018).CJOEE31671-769410.3788/COL
[12] 12WeberJ.BeardP. C.BohndiekS. E., Nat. Meth.13, 639 (2016).1548-709110.1038/nmeth.3929
[15] 15ChenH.ChenZ.XuJ.HuJ., Chin. Opt. Lett.13, 111701 (2015).CJOEE31671-769410.3788/COL
[16] 16WangL. V., Nat. Photonics3, 503 (2009).NPAHBY1749-488510.1038/nphoton.2009.157
[17] 17LiC. H.WangL. V., Phys. Med. Biol.54, R59 (2009).PHMBA70031-915510.1088/0031-9155/54/19/R01
[18] 18WangL. V.HuS., Science335, 1458 (2012).SCIEAS0036-807510.1126/science.1216210
[19] 19BeardP., Interface Focus1, 602 (2011).10.1098/rsfs.2011.0028
[20] 20BrechtH. P., J. Biomed. Opt.14, 064007 (2009).JBOPFO1083-366810.1117/1.3259361
[24] 24LiL.ZhuL.MaC.LinL.YaoJ.WangL.MaslovK.ZhangR.ChenW.ShiJ., Nat. Biomed. Eng.1, 0071 (2017).
[25] 25HuangG.YangS.YuanY.XingD., Appl. Phys. Lett.99, 125 (2011).APPLAB0003-6951
[28] 28TianG.ZhangX.ZhengX.YinW.RuanL.LiuX.ZhouL.YanL.LiS.GuZ., Small10, 4160 (2015).SMALBC1613-6810
[29] 29TanY.XiaK.RenQ.LiC., Opt. Express25, 8022 (2017).OPEXFF1094-408710.1364/OE.25.008022
[30] 30XuM.WangL. V., Phys. Rev. E71, 016706 (2005).10.1103/PhysRevE.71.016706
Kaiye Xia, Xiaohui Zhai, Zhaoheng Xie, Kun Zhou, Yutao Feng, Guangjie Zhang, Changhui Li. Dual-modal photoacoustic/CT imaging system[J]. Chinese Optics Letters, 2018, 16(12): 121701.