Measurement of Brillouin gain coefficient in fluorocarbon liquid
Download: 684次
Stimulated Brillouin scattering (SBS) has broad applications, such as in phase conjugation mirrors[1,2], optical limiting[3], optical sensing[4,5], and high-efficiency signal amplification[6,7]. Pulse compression from nanoseconds to sub-nanoseconds[8
Up until now, many kinds of gases, liquids, and solids have been investigated to find an efficient SBS medium. Fluorocarbon is now widely used as a new series of SBS media. It has many advantages, such as a high gain coefficient, high damage threshold, and short photon lifetime, all of which contribute to higher energy reflectivity and a short Stokes pulse[13,14].
The gain coefficient is essential for the SBS medium, which has a great relationship with the scattering threshold and Brillouin amplification. So far, the reported Brillouin gain coefficient values of fluorocarbon are mainly calculation results[15]. This method, however, suffers the disadvantages of the uncertainty of the electrostriction coefficient and the bandwidth. In order to obtain an accurate value, an experimental method is presented[16,17] based on small-signal Stokes amplification in a Brillouin amplifier.
In this Letter, we report the experiment results of the Brillouin gain coefficient measurement with a pump light wavelength of 532 nm, and demonstrate the theoretical basis of the method. Four kinds of different fluorocarbons are measured. Compared to the theoretical values, the experimental results have a higher reliability.
In the small-signal situation (
The experimental optical path is shown in Fig.
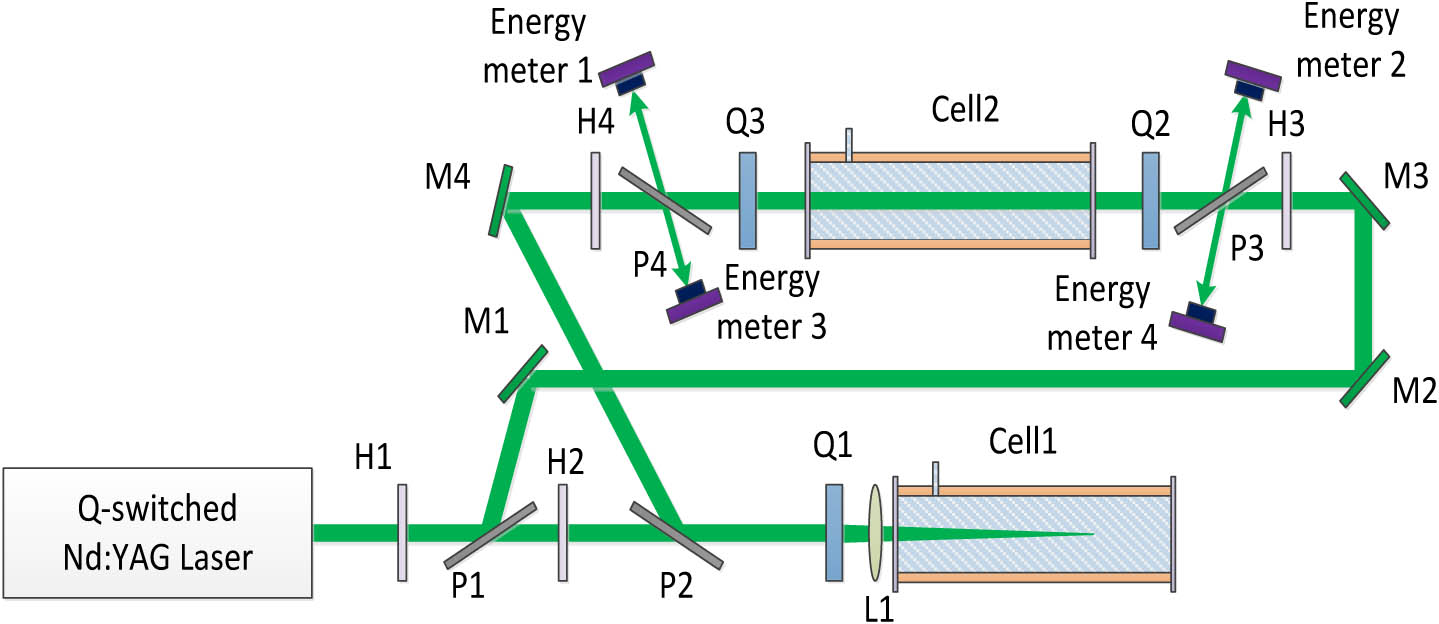
Fig. 1. Optical path used for Brillouin gain coefficient measurements, M1–M4, 532 nm reflection mirrors; H1–H4, half-wave plates; Q1–Q3, quarter-wave plates; P1–P4, Polarizers; L1, lens, focal length 400 mm; Cell 1, SBS oscillator; Cell 2, SBS amplifier.
These media all belong to perfluorinated amines; they all have chemical structure
In the amplifier cell, we make sure that the meeting position of the Stokes pulse and the pump pulse is in the middle of the cell. A 50 cm cell limits the interaction length in amplification. There is a difference in the pulse width overlap. It should take a bounded integration in the separation of the pulse peaks:
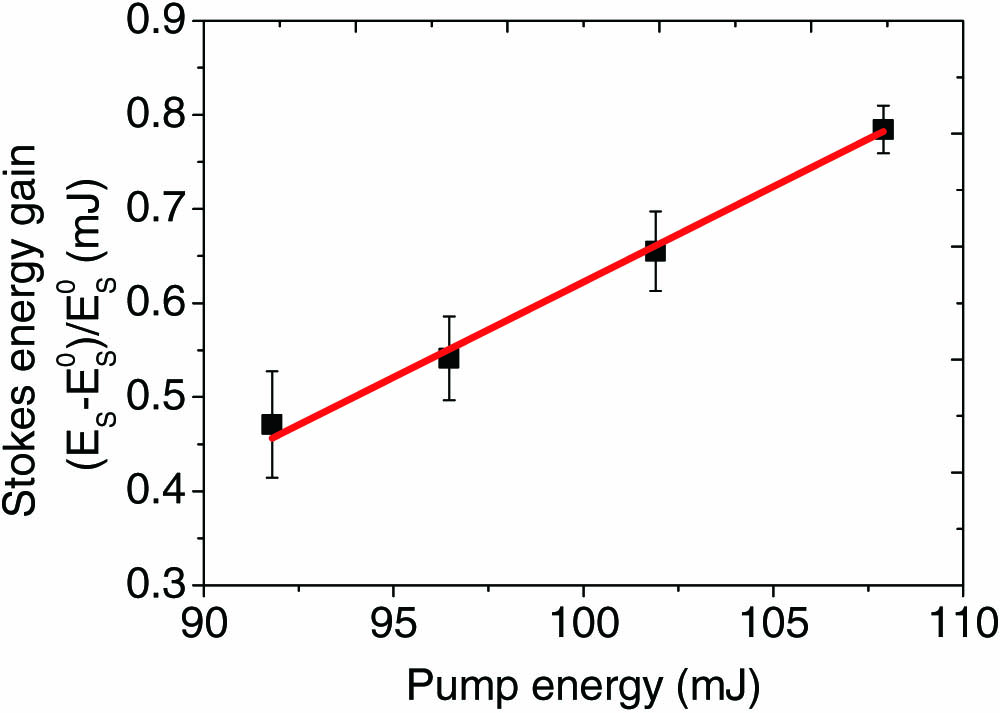
Fig. 2. Typical dependence of Stokes energy gain on pump energy at 532 nm for FC-770. The red line is the linear fit.
The waveforms of the input Stokes light and the pump light are detected by a photodiode (Ultrafast Photodetectors, UPD-40-UVIR-P, rising time 40 ps). Their pulses can be viewed on an oscilloscope (LeCroy Wave Master 806Zi-A) with a bandwidth of 6 GHz and a resolution of 40 GS/s, so

Fig. 3. Typical waveform of the pump light (black) and Stokes light (red) recorded by a photodiode (in FC-770).
According to the theoretical analysis, the Brillouin gain coefficient values are calculated by Eq. (
The measured values of the Brillouin gain coefficients of a few kinds of fluorocarbons are shown in Table
Table 1. Measurement Values of Brillouin Gain Coefficient
|
It is reported that the Brillouin gain coefficient can be calculated by a well-known formula, as follows:
In a previous work, the SBS energy reflectivity of different pump energies in FC-70 was seen to be similar as that in FC-43. However, according to the calculated results of the Brillouin gain coefficient, the value of FC-70 is 0.2 cm/GW, which is much lower than FC-43. On the basis of the SBS characteristic investigation of FC-70[18], we believe that 0.895 cm/GW is much closer to the true Brillouin gain coefficient of FC-70.
The motivation for measuring the Brillouin gain coefficient depends on the needs in designing the Brillouin pulse compressor, which is required by pulse compression from nanoseconds to sub-nanoseconds. Based on the measurement results, the suitable amplification medium can be chosen with a relatively high SBS energy efficiency.
In conclusion, we measure the value of the Brillouin gain coefficient of a few kinds of fluorocarbons (FC-40, FC-770, FC-70, and FC-43) at 532 nm experimentally through the process of Brillouin amplification. The measured values of FC-40, FC-770, and FC-43 are in agreement with the previously calculated values. However, FC-70 is different from the previous work. Our work provides a method of measuring the Brillouin gain coefficient values under varying experiment conditions.
[1]
[2]
[3]
[4]
[5]
[6]
[7]
[8]
[9]
[10]
[11]
[12]
[13]
[14]
[15]
[16]
[17]
[18]
[19]
[20]
[21]
Hang Yuan, Yulei Wang, Zhiwei Lu, Rui Liu, Can Cui. Measurement of Brillouin gain coefficient in fluorocarbon liquid[J]. Chinese Optics Letters, 2016, 14(4): 041902.