Mode division multiplexed holography by out-of-plane scattering of plasmon/guided modes
Download: 576次
Controlling light to transfer more information has always been the pursuit of people, not only in optical communications but also in imaging systems. Holography[1] is such a general approach in optical phenomena that enables the recording and reconstruction of the full amplitude and phase information of an object. With the invention of computer-generated holograms[2], people are able to create holograms of virtual objects that do not exist in reality. Compared with conventional holograms (e.g., by a spatial light modulator), the recently developed metasurface[3
In fact, people have developed dielectric-loaded plasmonic waveguides (DLPWs) to optimize the mode confinement and propagation loss[35,36]. When the dielectric layer is thick enough, a DLPW is able to carry more guided modes, including the transverse electric (TE) field ones, which have less mode field inside the metal part and exhibit a much lower loss. Such a kind of mode demultiplexer has been demonstrated in in-plane mode focusing[35], which indicates a possible application of the out-of-plane hologram like the SPP does[31,34]. In this Letter, we extend the multiplexed hologram by SPP scattering to the DLPW in which both TM and TE and their higher-ordered modes can be used for scattering holography. Unlike the previous SPP propagation-dependent multiplexing[34], this new proposed mode division multiplexed hologram (MDMH) can generate multiple free-space images in the same input direction as the guided modes. Here, holographic images multiplexed both in TM/TE and
First, it is necessary to introduce the diffraction process to manipulate the guided-mode holograms in the DLPW. A novel phase modulation method for propagating plane waves by in-plane diffractions from nonperiodic nanoarrays was first introduced in SPP systems[26]. By defining nanoscatterers at proper locations, the phase of the propagating field can be selected for the diffracted beam engineering, e.g., Airy SPP[26], collimated SPP[29,37], and winding SPP[30]. This in-plane diffraction method was further extended to out-of-plane beam engineering[31,38] and holography[34] on one hand and to mode division multiplexing (MDM) focusing in DLPW systems[35] on the other hand. Here, we incorporate the DLPW modes into multiplexed holograms by defining the corresponding phase evolution of the diffracted guided modes, which can be written as
Before getting into the experimental details, we need to analyze the mode properties in the DLPW. Figure
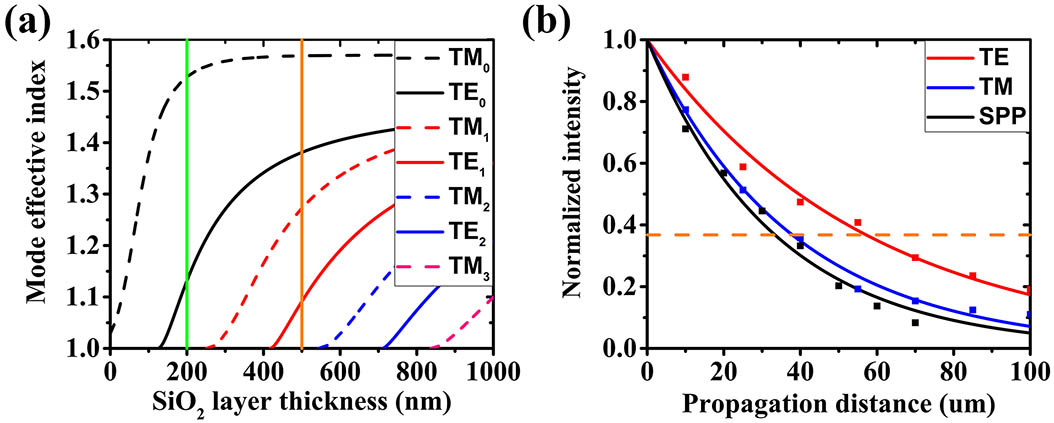
Fig. 1. (a) Calculated mode effective index of the DLPW with respect to different layer thickness including all TE and TM modes. (b) The normalized loss measurement of SPP, TE, and TM modes. The symbols are the experimental data and the curves are the exponential fittings. The orange horizontal dotted line means the guided wave’s intensity attenuated to .
The experimental setup is shown in Fig.

Fig. 2. (a) Illustration of the experimental setup; (b) a schematic of the DLPW where multiple modes are launched by a grating in the silver film from an incident laser beam and image over our sample; (c) a top-view scanning electron microscope (SEM) image of the couple-in grating and nanoscatterers in which the inset figure is the zoom-in image showing the details of the hologram units.
According to the MDM method introduced above, the designed object imaging could be reconstructed by a proper guided wave illumination on a well-arranged nanoscatterers hologram. It is necessary to mention that holography has a broadband effect that might lead to crosstalk when we use different modes for multiplexing. Fortunately, such a reconstruction cannot be totally fulfilled by other guided modes for their unsatisfied phase evolutions. Here, taking the focusing case and a 200 nm thickness single-mode waveguide for example, the effective mode indices of
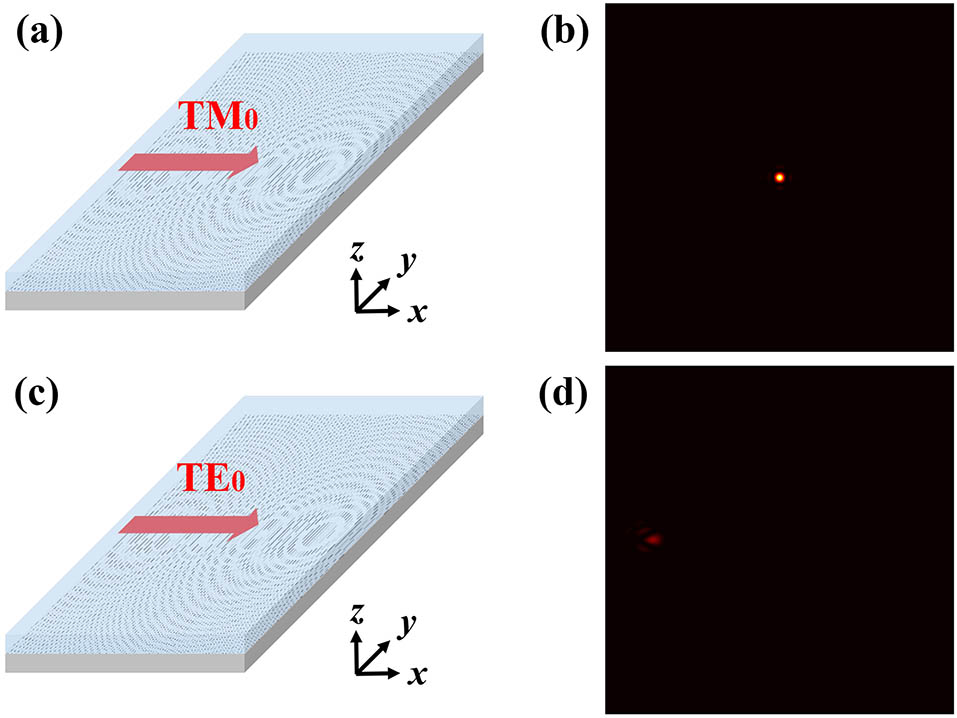
Fig. 3. Schematic of the guided-mode propagating through a well-designed hologram for (a) and (c) along the direction; (b) and (d) are the calculation results by the diffraction Huygens–Fresnel principle with respect to the hologram structures under and illumination, respectively. The size is .
For a good MDMH, a suitable design of the nanoscatterers distribution is important. Figures

Fig. 4. (a) and (b) The hologram patterns of a single object letter ‘E’ with respect to the mode as the reference wave and letter ‘M’ with respect to the mode as the reference wave, respectively; (c) the overlapped hologram pattern for two holographic images, and (d) the zoom-in figure; (e) shared scatterers between two hologram patterns; (f) the final designed hologram structure with a well-selected nanoscatterers array.
Due to the different losses and polarizations of TM and TE modes, two kinds of MDM holography are carried out in experiments. One is the TM/TE-based MDMH, in which the guided modes can be launched by the same grating (according to a certain bandwidth) coupling by different polarization incidences. The sample was fabricated by FIB etching on silver film with PECVD depositing a 200 nm thick

Fig. 5. (a) and (c) The SEM images of a couple-in grating and a multiplexed hologram by and . The size of the nanoscatterers array is due to the loss of TM mode. The red arrow in the upper-right corner represents the polarization of the incident lights and the red arrow in the center-left with the letters ‘TE’ or ‘TM’ represents the excited guided mode. (b) and (d) The experimental measurements of the capital letters ‘E’ and ‘M’ images decoded from the multiplexed hologram according to TE and TM mode excitation, respectively.
The other approach is to multiplex multimodes in the same polarization and take the
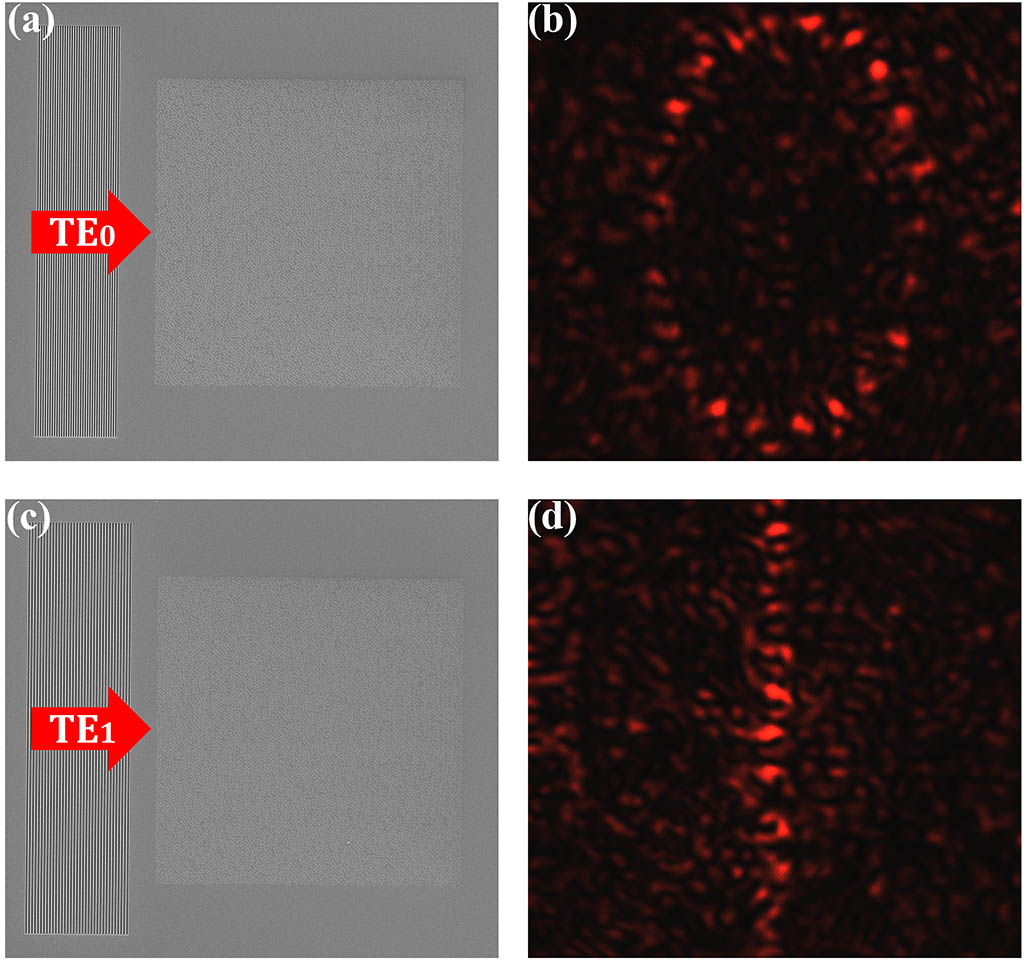
Fig. 6. (a) and (c) The SEM images of the totally same multiplexed hologram by and but different couple-in gratings with different periods. The size of the nanoscatterers arrays is due to the low loss of the TE mode. The periods of the gratings in (a) and (c) are equal to the effective wavelengths of and , respectively. (b) and (d) The experimental measurements of the number ‘0’ and ‘1’ images decoded from the multiplexed hologram according to and mode excitation, respectively.
In conclusion, we theoretically proposed and experimentally demonstrated mode division multiplexed holograms based on DLPW. Both TM/TE and
[1]
[2]
[3]
[4]
[5]
[6]
[7]
[8]
[9]
[10]
[11]
[12]
[13]
[14]
[15]
[16]
[17]
[18]
[19]
[20]
[21]
[22]
[23]
[24]
[25]
[26]
[27]
[28]
[29]
[30]
[31]
[32]
[33]
[34]
[35]
[36]
[37]
[38]
Chenchen Zhao, Ji Chen, Hanmeng Li, Tao Li, Shining Zhu. Mode division multiplexed holography by out-of-plane scattering of plasmon/guided modes[J]. Chinese Optics Letters, 2018, 16(7): 070901.