All-optical logic gates and a half-adder based on lithium niobate photonic crystal micro-cavities
Download: 2686次
Current all-optical logic gates are predominantly fabricated in optical fiber waveguides, which make it difficult to integrate on small integrated chips[1,2], whereas defect waveguides in photonic crystals (PhCs) possess small scale and unique light controlling ability[3] that make it a crucial method to design miniaturized all-optical logic gates. Until now, all-optical logic gates are mostly designed and integrated on silicon-based PhC materials[4,5]. However the two-photon absorption and third-order nonlinearity in silicon may cause nonlinear loss and signal crosstalk, which results in low extinction ratio in silicon logic gates. Consequently, a new optical material integration platform is demanded, such as lithium niobate (
We have experimentally demonstrated polarization-based binary optical logic gates by employing the Pockels effect in periodically poled LN in our previous research works[15,16], where we only get an extinction ratio of about 10 dB. In this Letter, we have designed three basic all-optical logic gates with 23 dB, including AND, XOR, and NOT gates in an LN PhC by employing PhC micro-cavities. We have found that the extinction ratio between the logic 1 and 0 states can be improved by adding PhC-defect micro-cavities in the PhC circuits[17,18]. By utilizing the single-line-defect (W1) waveguides and two-missing-hole (L2) cavities, the extinction ratio of the logic gates we designed can reach a maximum as high as 23 dB in the finite-difference time-domain (FDTD) simulations (the simulated software used is RSOFT). It is the highest extinction ratio obtained, to the best of our knowledge, compared with 20.53 dB in silicon PhC[4]. Finally, an all-optical half-adder was also designed and realized effectively by the combination of these basic logic gates in an LN PhC.
Figure
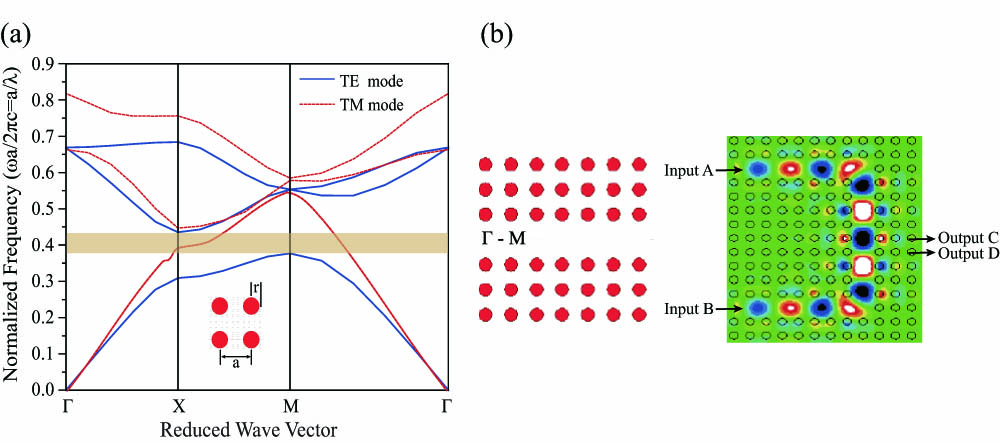
Fig. 1. (a) Band diagrams in a square lattice PhC with LN (MgO-doped) rods for TE and TM modes. (b) The left is the schematic structure of the W1 waveguide in the -M direction; the right is the FDTD simulation result of the W1 waveguide and the sketch of the light beam coherent interference.
The light cannot propagate in the PhC forbidden band. However, the defect waveguides called W1 waveguides[20] in the PhC possess unique light guiding ability due to the existence of the band gap. So the W1 waveguide is designed as the basic element of the all-optical logic gates in LN PhC structures. The PhC line-defect waveguide is fabricated by removing the line rods, as shown in the left of Fig.
The W1 waveguide can guide the light in the PhC structure, and besides, the point-defect micro-cavities located next to the line-defect waveguides in PhC can effectively affect the transmission of the light beams. There are several different micro-cavities in the PhC, one of which is the other basic element called the L2 cavity formed by getting rid of two adjacent rods[20]. Figure
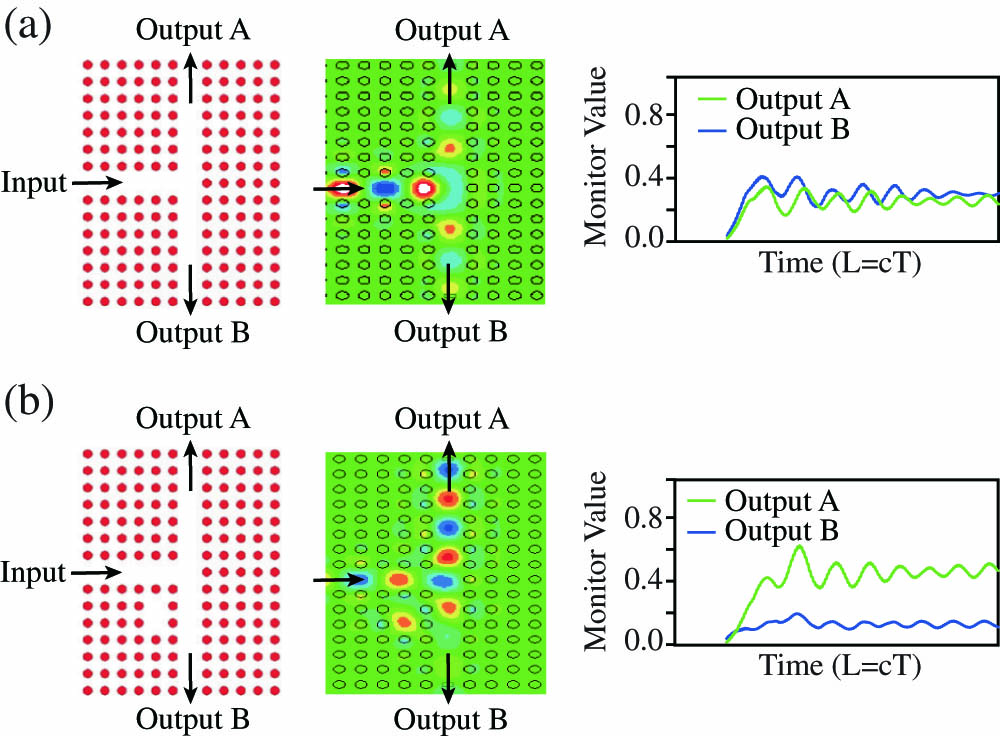
Fig. 2. T-shape W1 waveguides (a) without or (b) with the L2 cavity in an LN PhC and the FDTD simulated different transmission results.
Here, we proposed a complicated LN PhC structure to realize three basic all-optical logic gates and a half-adder with a high extinction ratio by combining the W1 waveguides with L2 cavities. Figure
Table 1. Truth Table for the AND, XOR, and NOT Logic Gates
|

Fig. 3. Three all optical logic gates (a) AND, (b) XOR, and (c) NOT in an LN PhC with different micro-cavities locations.
Figure
In Fig.
Then, we designed an all-optical half-adder by the combination of the AND and XOR gates, as shown in Fig.
Table 2. Truth Table for the All-optical Half-adder
|

Fig. 5. (a) Schematic structure of the all-optical half-adder in an LN PhC. (b) The FDTD simulated transmission of the all-optical half-adder.
In conclusion, assisted by different micro-cavities in LN PhC circuits, we have achieved the AND, XOR, and NOT logic gates and an all-optical half-adder in an LN PhC, which has the highest extinction ratio of 23 dB compared with that in silicon logic gates[4,5]. This logic gate design may benefit complex integrated all-optical functionalities in the LN or LNOI photonics device platform, such as optical quantum computing by combining parametric down-conversion nonlinear processes[22].
[2]
[3]
[4]
[5]
[6]
[7]
[8]
[9]
[10]
[11]
[12]
[13]
[14]
[15]
[16]
[17]
[19]
[20]
[21]
[22]
Chenghao Lu, Bing Zhu, Chuanyi Zhu, Licheng Ge, Yian Liu, Yuping Chen, Xianfeng Chen. All-optical logic gates and a half-adder based on lithium niobate photonic crystal micro-cavities[J]. Chinese Optics Letters, 2019, 17(7): 072301.