Simulating robust far-field coupling to traveling waves in large three-dimensional nanostructured high-Q microresonators
Download: 592次
1 State Key Laboratory of Information Photonics and Optical Communications, Beijing University of Posts and Telecommunications, Beijing 100876, China
2 College of Optical Sciences, University of Arizona, Tucson, Arizona 85721, USA
3 Department of Biomedical Engineering, University of Arizona, Tucson, Arizona 85721, USA
4 e-mail: judy@optics.arizona.edu
5 e-mail: euanmc@optics.arizona.edu
Figures & Tables
Fig. 1. Free-space coupling to a WGM microtoroid optical resonator via a phased array of gold nanorods. (a) Schematic view. (b) Collection of far-field scattering from the grating shows the Fano resonance corresponding to the interference between the grating and WGM resonances. (c) Established 2D axisymmetric simulations identify a resonance of the bare WGM at λ2≈633 nm, corresponding to an azimuthal mode number m=660. (d) The FloWBEM simulation of the same bare toroid as in panel (c). Surfaces S1 and S2 are simulated with Floquet boundary conditions, and S3 is simulated with scattering boundary conditions. (e) For simulating the driven system, a far-field domain (S4) is added at the circumference, replacing the Floquet and scattering boundary conditions for that region. (f) Nanorods are placed in the equatorial plane, between the light source, which is incident at 45°, and the silica toroid. A field continuity condition is applied between the light source and the domain surrounding the toroid.
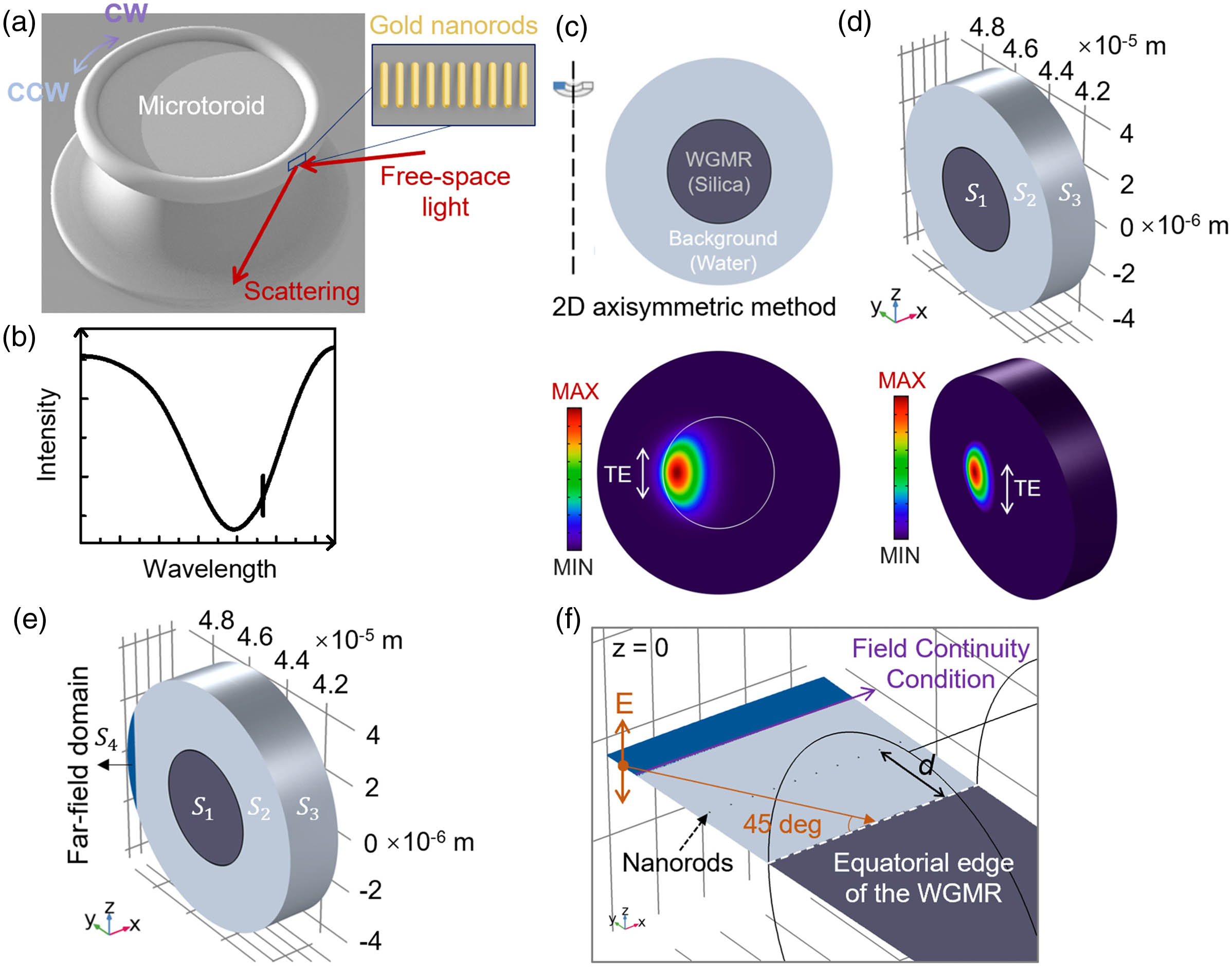
下载图片 查看原文
Fig. 2. Frequency domain simulations of the driven system. (a) Intracavity energy of the coupled TE WGM with a grating spaced d=1000 nm from the toroid. Zoom-in: ΔλFWHM=0.14 pm. (b) Intracavity energy of the coupled TM WGM with a grating-toroid spacing d=1000 nm. Zoom-in: ΔλFWHM=0.22 pm. (c) TE WGM backaction-mediated reflection spectrum corresponding to the same simulation as panel (a). (d) TM WGM backaction mediated reflection spectrum corresponding to the same simulation as panel (b). In all panels, the wavelength step is 0.5 nm for the broad spectrum, and between 0.005 pm and 2 pm in the vicinity of the resonance (insets). The SBR and δλFWHM values for panels (c) and (d) are given in Table 1.
下载图片 查看原文
Fig. 3. Effect of grating-WGM separation on linewidths. Loaded Q factors for both TE and TM polarizations depend on grating-toroid separation. Q factors are evaluated using three methods: eigenfrequency Re{fres}/(2 Im{fres}) (solid circles and lines), eigenfrequency 2πfresW/(Pabs+Prad) (hollow circles), and frequency domain λres/ΔλFWHM (red stars). Owing to the increased computational costs of frequency domain simulations, only four points are shown.
下载图片 查看原文
Fig. 4. Mode field distributions of the driven coupled system. (a) 3D frequency domain simulation of the microtoroid coupled to the grating with various separation distances. The CCW mode is shown, which is selected through appropriate choice of the Floquet boundary conditions. The incident light is s-polarized, which drives a TE WGM. Field distributions are plotted as field amplitude |E|. (b) Same as panel (a), but with a separation of d=1000 nm. (c) Same as panel (b), but where the CW solution has been selected through the choice of Floquet boundary conditions with opposite sign. (d) The superposition of the field distributions in (b) and (c).
下载图片 查看原文
Fig. 5. Reflected spectrum exhibiting a Lorentzian lineshape, corresponding to the third column in Table 1 of the main text (d=700 nm). The SBR is 0.05%.
下载图片 查看原文
Fig. 6. Reflected spectrum exhibiting a Fano lineshape, corresponding to the fifth column in Table 1 of the main text (d=700 nm). The SBR is 1.18%.
下载图片 查看原文
Fig. 7. Far-field scattering from isolated nanorods and nanorod arrays without a WGM resonator. (a) Intensity of the far-field scattering of a single nanorod excited at the LSPR. (b) 3D grating of 10 nanorods with nonphase matched periodicity Λ=228.5 nm, excited at normal incidence and at the LSPR of the individual rods. (c) Same as (b), but at 45° incidence. (d) Same as (c), but with nine nanorods with periodicity Λ=264.8 nm that is perfectly phase-matched for backward scattering when excited at 45° incidence. For the case of finite-length nanorods positioned near the curved surface of the toroid, we expect only partial phase matching (see main text Fig. 4) and not perfect phase matching. (e) Same as (c), but excited with transverse polarization, which would correspond to a TM-polarized WGM. (f) Same as (d), but excited with transverse polarization. The six panels are solved in frequency domain at a wavelength of 630 nm. The dark orange arrows denote the incoming angle of the light.
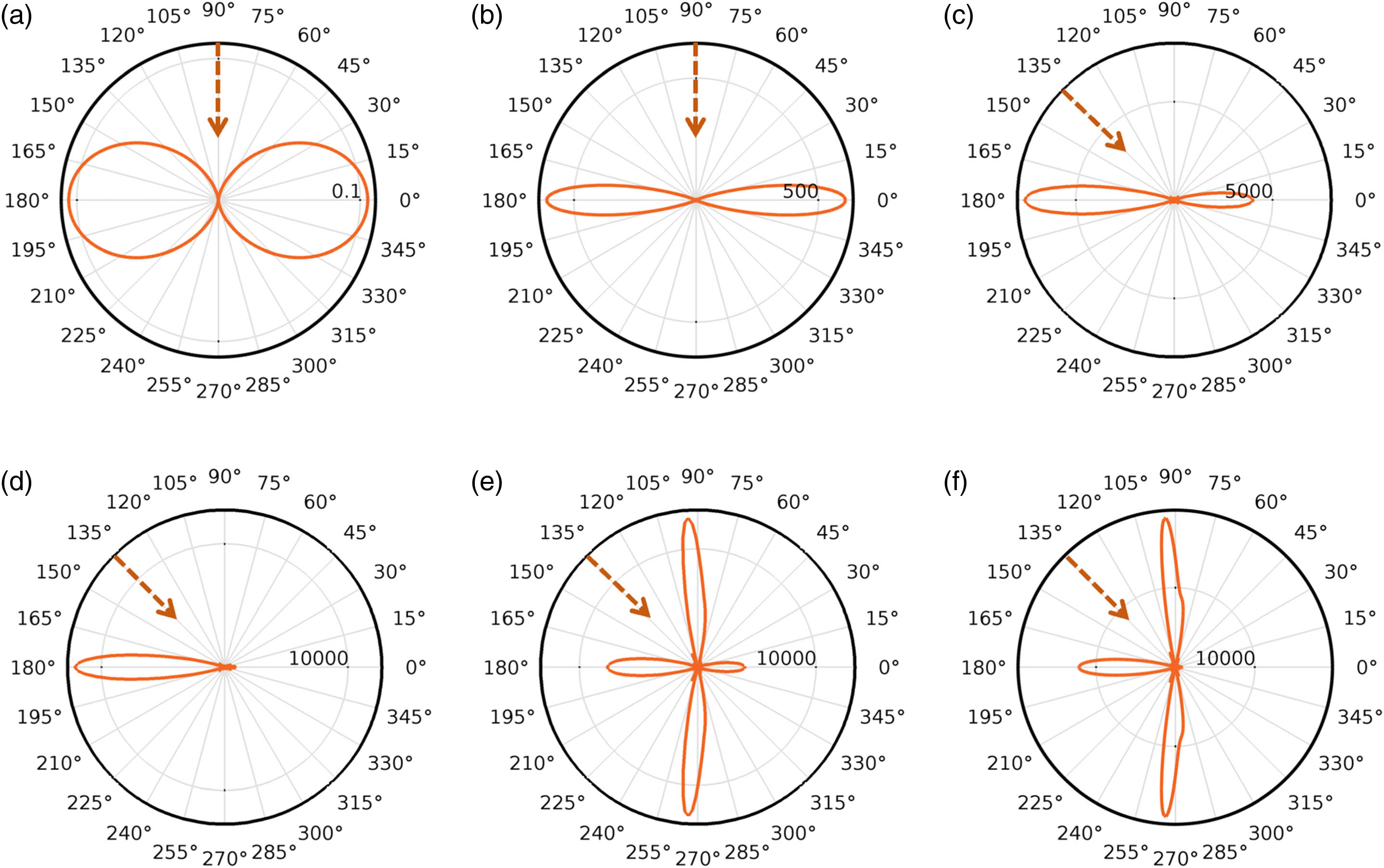
下载图片 查看原文
Fig. 8. Intracavity energy spectra for the TE CCW mode (blue) and the TE CW mode (red). The CCW mode is dominant due to partial phase matching between the incident light, grating periodicity, and WGM mode.
下载图片 查看原文
Fig. 9. WGM frequency shift for a single nanorod as a function of nanorod aspect ratio (AR).
下载图片 查看原文
Table1. Calculation Methods for Loaded of the Driven WGM Coupled to a Gratinga
| Driven TE | Driven TM | Coupling distance | 1000 nm | 700 nm | 1000 nm | 700 nm | Frequency domain driven () | | | | | Eigenfrequency coupled | | | | | Reflected spectrum linewidth () | 0.16 pm | 0.1 pm | 0.28 pm | 0.04 pm | SBR (from reflected spectrum) | 3.86% | 0.05% | 1.33% | 1.18% | Normalized intracavity energy () | | | | |
|
查看原文
Table2. Intracavity Energy of the Frequency Domain Driven TE CCW and CW Modes for Different Coupling Separation
(nm) | Energy of the Driven TE CCW (J) | Energy of the Driven TE CW (J) | Energy Ratio for the TE CCW/CW | 400 | | | 1.15 | 700 | | | 4.18 | 1000 | | | 2.08 |
|
查看原文
Lei Chen, Cheng Li, Yu-Min Liu, Judith Su, Euan McLeod. Simulating robust far-field coupling to traveling waves in large three-dimensional nanostructured high-Q microresonators[J]. Photonics Research, 2019, 7(9): 09000967.