亚波长光栅负折射透镜的柱矢量光束聚焦特性
下载: 1205次
1 引言
柱矢量光束是一种振幅、相位在传播方向横截面上呈柱对称分布的光束,按其在空间上的偏振分布特点可将其分为径向偏振光、旋向偏振光和一般柱矢量光束。其中,若对径向偏振光进行紧聚焦,在焦点附近可得到较强的纵向偏振光场,从而使得径向偏振光在高分辨率光学成像[1]、数据存储[2]、粒子捕获[3-4]、粒子加速[5-6]等方面有广泛的应用。目前实现柱矢量光束聚焦及焦场调控的方式和途径丰富多样,如:利用高数值孔径物镜,通过改变透镜孔径大小,并结合相位、振幅与偏振调制,可突破衍射极限产生亚波长的圆对称光斑[7-8];利用抛物面反射镜聚焦系统,通过反射光线实现聚焦,并能够在近轴条件下获得较好的聚焦效果[9-10];等离激元透镜能够产生突破衍射极限的高质量焦场[11],并能进一步灵活调节焦深及抑制旁瓣[12],有效实现对径向偏振光束的紧聚焦[13-14]。这些方法有各自的优势,但也存在不同的局限性。高数值孔径透镜聚焦效果受到孔径大小、相位、振幅的影响,并存在相差;从几何光学角度来讲,抛物面反射式透镜对光线的会聚能力取决于入射光线与主光轴的距离,只有在近轴条件下能够实现较好的聚焦;等离激元透镜因等离激元激发的偏振依赖性,对径向偏振光束有较好的聚焦效果,形成对聚焦光束的偏振态局限性[15]。
为使柱矢量光束的径向和旋向偏振光均能实现有亚波长聚焦,本研究组已研究了基于负折射效应的微结构透镜,光子晶体平凹透镜能够实现对径向、旋向和任意柱矢量光束的亚波长紧聚焦[16]。然而,光子晶体需要两种以上材料交替排列构成,并且在实现任意柱矢量光束聚焦时,结构设计需要满足横磁和横电偏振具有相同的负折射率。因此,在材料的选取、结构设计和实际制备上均存在一定难度。所幸的是,负折射全介质光栅具有负折射效应,并能够克服这些困难,通过合理选取光栅结构参数获得负折射率,并灵活设计透镜结构,能够实现对柱矢量光束的聚焦[17]。
负折射光栅的介质材料选取、负折射率设定及材料结构参数均会影响柱矢量光束的聚焦效果。研究分析这些参数对焦场调控的影响,利于进一步优化透镜结构,获得更好的焦场调控效果。因此,本文将对影响负折射光栅透镜聚焦效果的因素进行深入探究,包括光栅材料折射率、等效负折射率、结构参数等,分析焦场的焦距、能量效率、焦点的尺寸等受到的影响。
2 负折射光栅及聚焦透镜
利用亚波长介质光栅的-1级衍射波,可实现负折射效应[18-20]。在光栅周期小于工作波长时,可以获得等效的负折射率,并进一步设计出平凹镜结构来实现对光束的聚焦[21-22]。
由 (1) 式可知,当
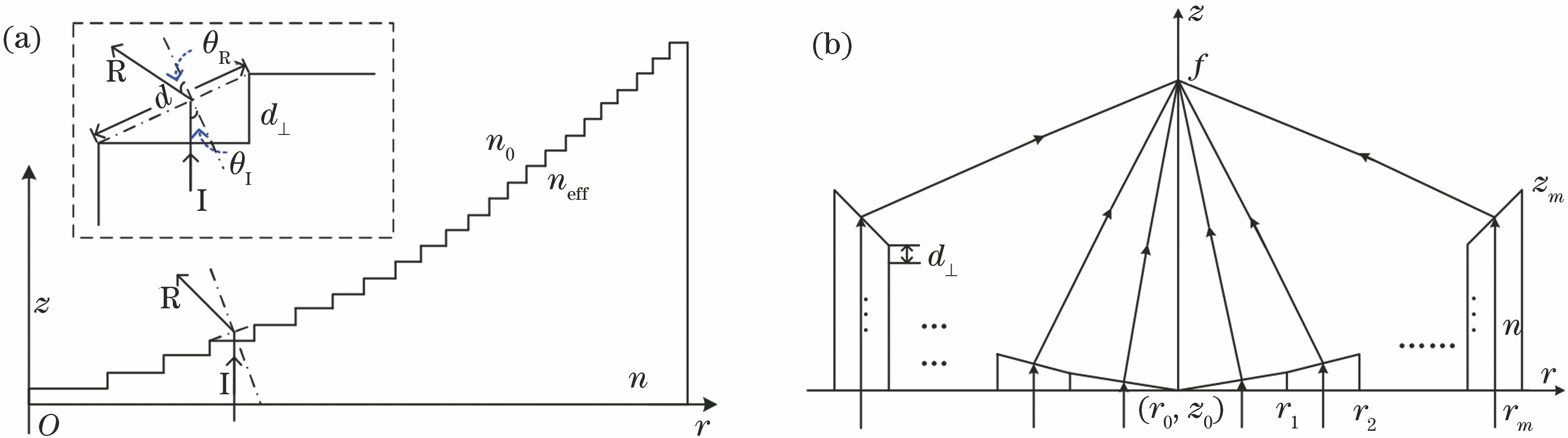
图 1. 平凹镜结构和聚焦示意图。(a)平凹镜r -z 剖面图;(b)聚焦过程示意图
Fig. 1. Profile of plane-concave lens and schematic of focusing process. (a) Cross-section of plane-concave lens in r -z plane; (b) schematic of focusing process
选取恰当的材料折射率和光栅几何参数
式中:
利用基于有限元算法(FEM)的COMSOL Multiphysics软件进行光场聚焦的模拟计算。因透镜结构及入射光束的振幅和偏振均具有柱对称分布特性,所以选择2D轴对称坐标系进行建模,将3D模型降维到2D,可降低计算机运算成本及计算时间。当
3 材料及结构参数对聚焦特性的影响
介质材料的合理选取是构成负折射光栅的必要条件,也是进一步设计光栅平凹镜的前提。文中所研究的负折射光栅平凹透镜由单一介质材料制成,全介质光栅对选取材料的限制较小,选取3种材料折射率值作为数值分析的模型参数,如Si3N4为2, Ag2S为2.20,ZnS为2.36,材料折射率的不同选取,会影响光栅负折射率及平凹镜结构参数之间的关系,进而影响聚焦效果。当设定光栅等效负折射率
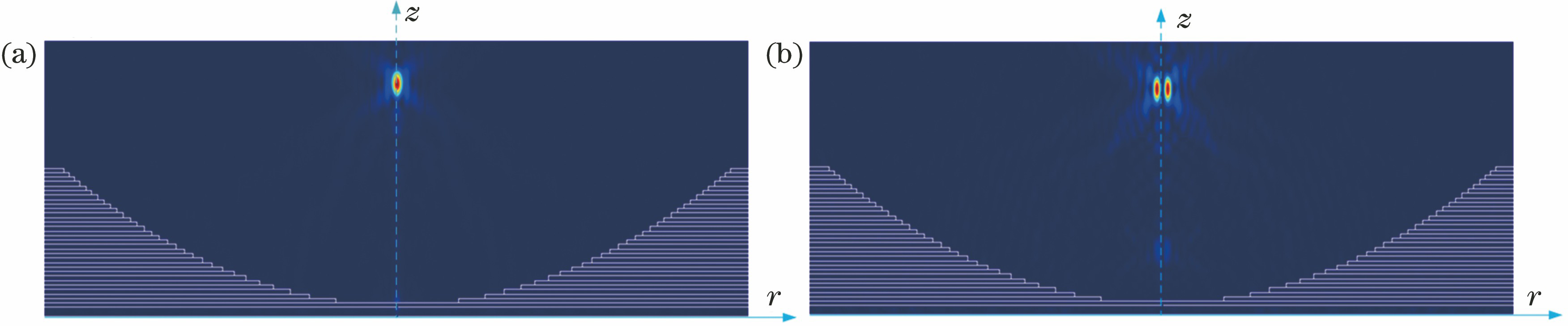
图 2. 预设焦距为f =8 μm的聚焦场分布。(a)径向偏振入射;(b)旋向偏振入射
Fig. 2. Intensity distributions of focal field with predetermined f =8 μm. (a) Radial polarization incidence; (b) azimuthal polarization incidence
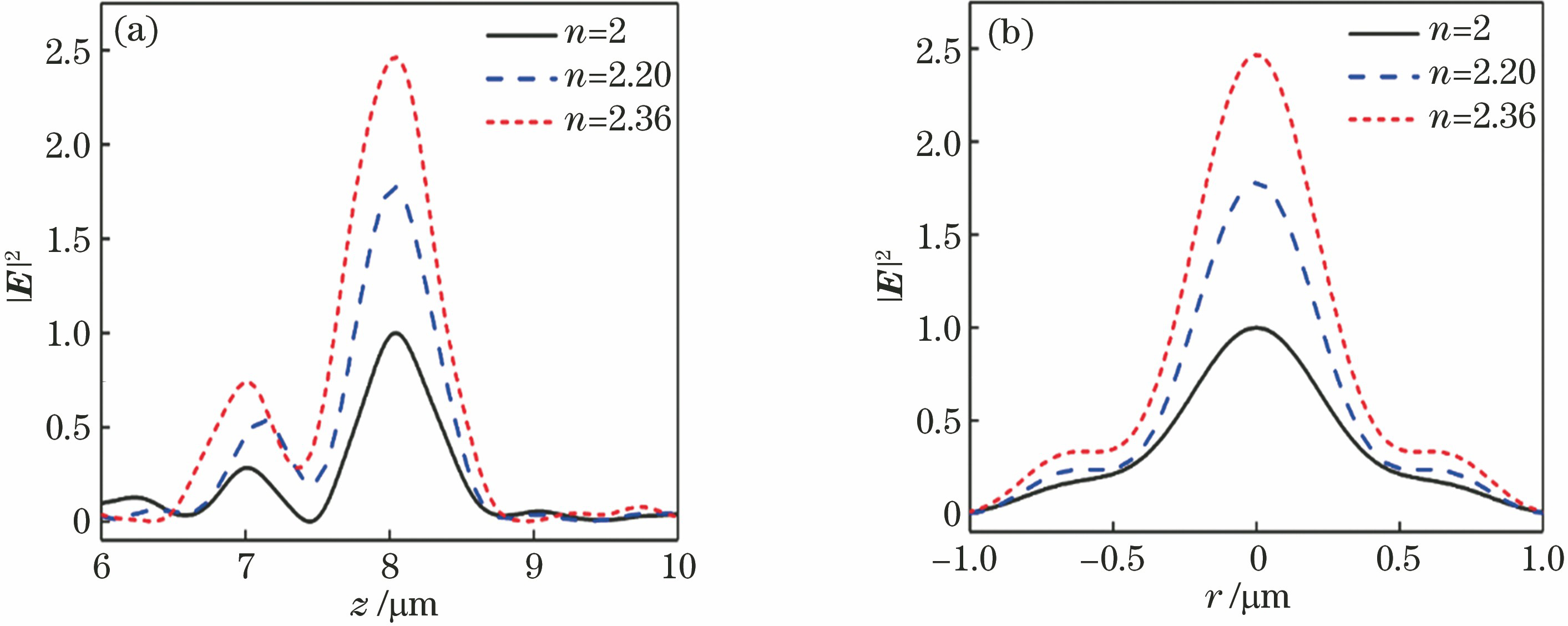
图 3. 预设焦距f = 8 μm,材料折射率n =2,2.20,2.36的聚焦场。(a)沿轴向的场强分布;(b)沿横向(径向)的场强分布
Fig. 3. Intensity distributions of focal field with predetermined f =8 μm and different refractive indices of n =2, 2.20, and 2.36, respectively. (a) Intensity distribution of field along axial direction; (b) intensity distribution of field along lateral direction (radial direction)
选取材料折射率

图 4. 预设焦距为 f =8 μm,等效负折射率为 n eff=-0.8, -0.9, -1.0, -1.1, -1.2的聚焦场。(a)沿轴向的场强分布;(b)沿横向(径向)的场强分布
Fig. 4. Intensity distributions of focal field with predetermined f =8 μm and different equivalent negative refractive indices of n eff=-0.8, -0.9, -1.0, -1.1, and -1.2 respectively. (a) Intensity distribution of field along axial direction; (b) intensity distribution of field along lateral (radial) direction
选择材料折射率为
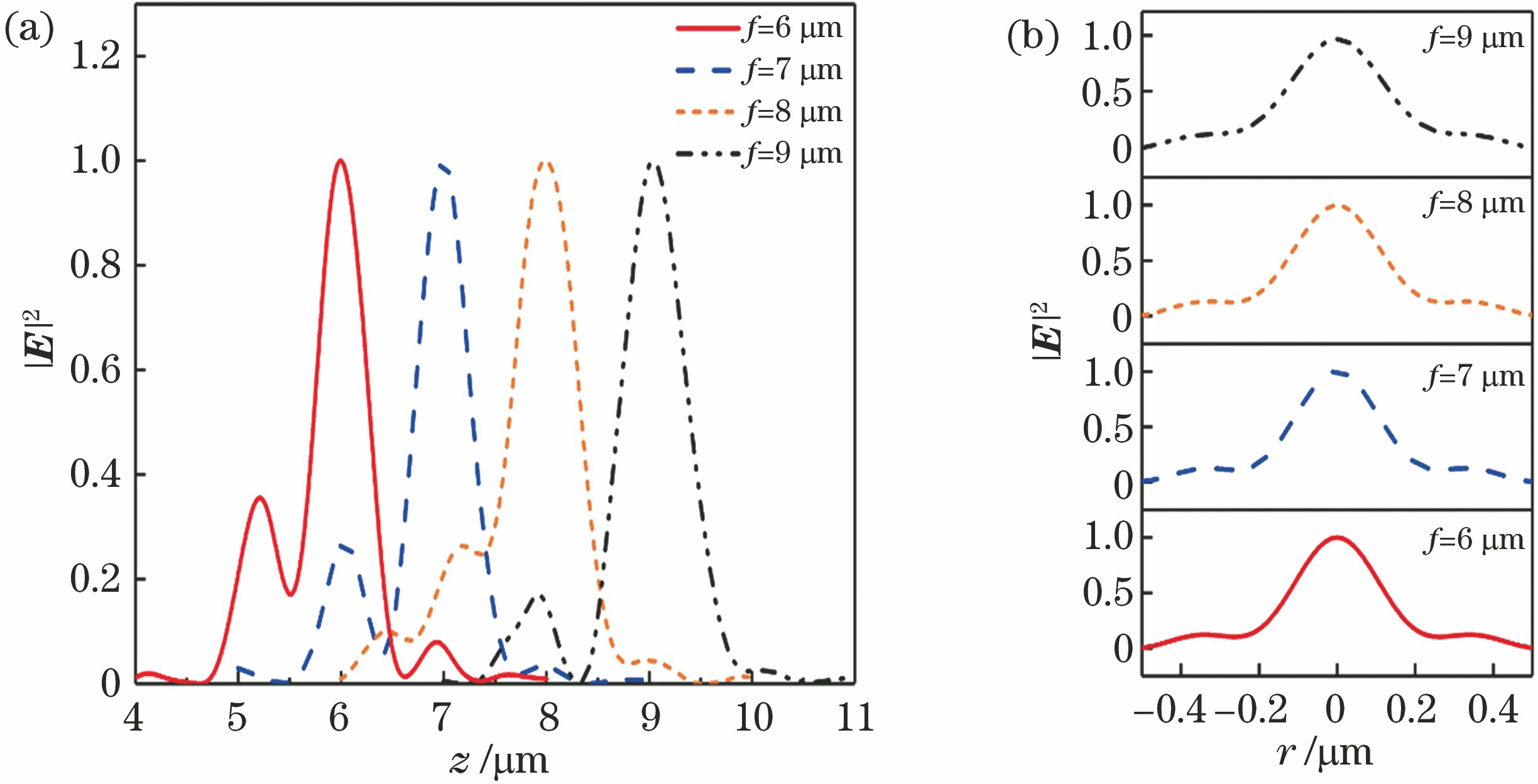
图 5. 材料折射率n =2.36、等效负折射率n eff=-1.0时,预设焦距为f =6、7、8、9 μm的聚焦场。(a)沿轴向的场强分布;(b)沿横向(径向)的场分布
Fig. 5. Intensity distributions of focal field with predetermined f =6, 7, 8, and 9 μm, as n =2.36 and n eff=-1.0. (a) Intensity distribution of field along axial direction; (b) intensity distribution of field along lateral (radial) direction
径向偏振光从透镜出射面出射后,电场横向振动分量将向纵向转化,而聚焦场处纵向场分量越高,焦点尺寸越小[23]。当

图 6. f =6 μm和f =9 μm焦点处的聚焦场分析。(a)(b) |E |2分布的等高线图;(c)(d) |Ez |2分布的等高线图;(e)(f) |E |2、|Ez |2和|Er |2沿横向(径向)的分布曲线
Fig. 6. Analyses of focal field with f =6 μm and f =9 μm. (a)(b) Contour plots of |E |2; (c)(d) contour plots of |Ez |2; (e)(f) distributions of |E |2, |Ez |2, and |Er |2 along lateral (radial) direction
4 结论
利用亚波长全介质光栅-1级衍射的负折射效应,设计等效负折射率聚焦透镜,并以柱矢量光束为入射光,以径向偏振光为例,详细分析各参数对聚焦效应的影响。结果表明,材料的折射率、等效负折射率、预设焦距的选取均对聚焦效果有一定程度的影响。具体表现为:大的材料折射率有较高的聚焦效率,并能获得较小的焦点横向尺寸;等效负折射率会影响聚焦效果,
[1] 李润丰, 施可彬. 基于光场调控的高时空分辨率光学成像[J]. 光学学报, 2019, 39(1): 0126010.
[7] 郑晓, 杨艳芳, 何英, 等. 双环贝塞尔-高斯径向偏振光束经介质分界面的强聚焦[J]. 光学学报, 2016, 36(4): 0426001.
[15] 仲义, 许吉, 陆云清, 等. 基于一维金属光子晶体平凹镜的柱矢量光束亚波长聚焦[J]. 物理学报, 2014, 63(23): 237801.
[20] Shalaev VM, Kildishev AV, Klar TA, et al. Optical negative-index metamaterials: from low to no-loss and from linear to nonlinear optics[C]//LEOS 2006-19th Annual Meeting of the IEEE Lasers and Electro-Optics Society, October 29-November 2, 2006, Montreal, Que., Canada. New York: IEEE, 2006: 246- 247.
[23] Grosjean T, Courjon D. Smallest focal spots[J]. Optics Communications, 2007, 272(2): 314-319.
Article Outline
刘山峰, 袁沭娟, 孙钰淇, 杨茜, 刘扬眉, 刘宁, 陆云清, 许吉. 亚波长光栅负折射透镜的柱矢量光束聚焦特性[J]. 光学学报, 2019, 39(11): 1105001. Shanfeng Liu, Shujuan Yuan, Yuqi Sun, Qian Yang, Yangmei Liu, Ning Liu, Yunqing Lu, Ji Xu. Focusing Properties of Cylindrical Vector Beams Through Subwavelength Grating Lenses with Negative Refractive Indices[J]. Acta Optica Sinica, 2019, 39(11): 1105001.