20.231 Gbit/s tricolor red/green/blue laser diode based bidirectional signal remodulation visible-light communication system
1. INTRODUCTION
Wireless communication is becoming more and more crucial in our daily lives. As the traditional radio-frequency communication band is highly congested, visible-light communication (VLC) has been proposed as a supplementary means of wireless communication providing high data rate (
In the literatures, mainly single direction high speed LD-based VLC systems are reported. It is important to have a bidirectional transmission for practical VLC deployment. In this work, we propose and experimentally demonstrate a recorded bidirectional tricolor RGB LD-based VLC system supporting signal remodulation. In the signal remodulation system, an LD source is not needed at the client side. The client can reuse the downstream wavelength sent from the central office (CO) and remodulate it to produce the upstream signal. As the LD sources are located at the CO, the laser wavelength and temperature managements at the cost-sensitive client side are not needed; and the system could be cost effective and energy efficient. Here, the downstream is the tricolor RGB signal. A high spectral efficiency OFDM format with bit-loading is employed. A total data rate of 20.231 Gbit/s (R: 11.023 Gbit/s, G: 4.618 Gbit/s, B: 4.59 Gbit/s) OFDM downstream and 2 Mbit/s signal remodulated OOK upstream can be achieved in 1 m free-space transmission. This is the first demonstration, to our knowledge, of a
2. EXPERIMENTAL SETUP
Figure
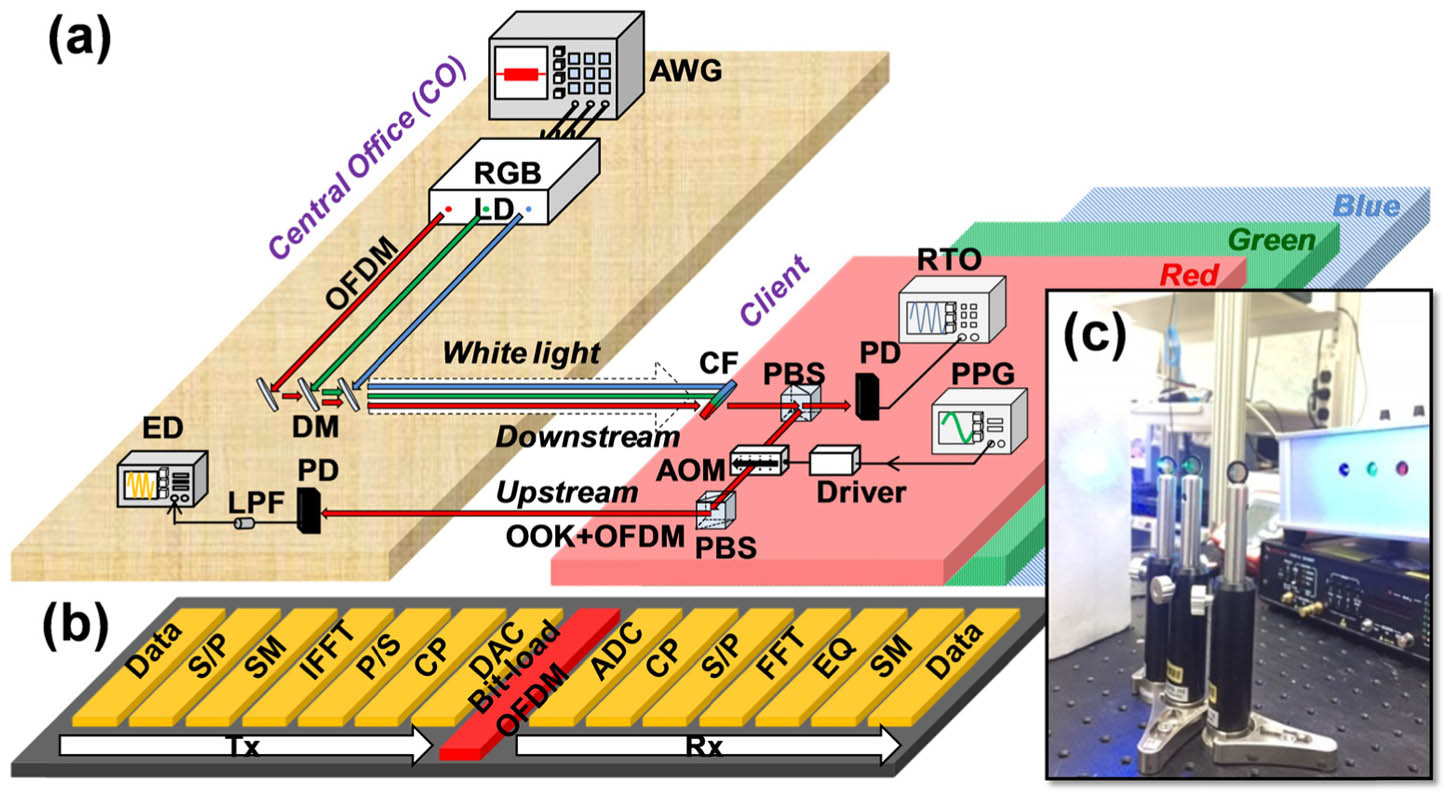
Fig. 1. (a) Experimental setup of tricolor RGB LD-based bidirectional VLC system. (b) OFDM signal encoding and decoding processes. (c) Photography of tricolor RGB LD to produce white light. AWG, arbitrary waveform generator; PBS, polarization beamsplitter; DM, dichroic mirror; PD, photodiode; RTO, real-time oscilloscope; CF, color filter; AOM, acousto-optic modulator; ED, error detector; PPG, pulse-pattern generator; LPF, low pass filter.
The three LDs are in TO packages with wavelengths of the red, green, and blue LDs of 676.6, 514.8, and 449.3 nm, respectively. Figure
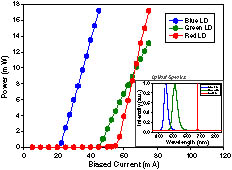
Fig. 2. Experimental output optical power and biased current responses of the RGB LDs. Inset: measured optical spectra.
Encoding and decoding of the OFDM signal are implemented by using offline MATLAB program. Figure
Then the RGB signals are wavelength division demultiplexed by using three different color filters (red: 680 nm; green: 520 nm; blue: 450 nm) to select the red, green, and blue wavelength channels. In this VLC system, only the optical intensity of the LD is modulated, and the optical detection mechanism is based on intensity modulation/direct detection. The optical receiver used in the LD-based VLC is a silicon-based amplified positive–intrinsic–negative (PIN) photodiode (PD) (EOT, ET-2030A), which utilizes the photovoltaic effect to convert optical power into an electrical current, and a fixed gain trans-impedance amplifier for signal amplification. It has an optical-to-electrical bandwidth of 1.2 GHz, and active area diameter of 400 μm. A real-time oscilloscope (RTO, Tektronix DPO7354C) is used for OFDM demodulation. The RTO provided the function of an analog-to-digital converter for signal decoding and analysis. The OFDM decoding process is also shown in Fig.
In order to produce the wavelength remodulated upstream signal, one part of the downstream signal will be taped out via a polarization beamsplitter (PBS). In this proof-of-concept demonstration, we use the red color channel to generate the upstream wavelength remodulation signal. The signal is launched into an acousto-optic modulator (AOM) connected to an electrical pulse-pattern generator (PPG) to produce the upstream OOK signal. Since there was only one AOM available in the laboratory, only the red upstream remodulated channel is demonstrated. The produced upstream remodulated signal is then received by another PIN PD connected to an error detector (ED) at the CO to measure the BER of the upstream signal.
At the CO, an electrical low pass filter (LPF) with 3-dB bandwidth of 20 MHz is connected after the PD to erase the high frequency downstream OFDM signal so that high quality of upstream remodulated OOK signal can be received. As the available AOM has a modulation bandwidth of only 1.8 MHz, we demonstrate the upstream remodulation operated at 2 and 1 Mbit/s. The upstream remodulated OOK signal is limited by the modulation bandwidth of the AOM, and higher data rates are possible if higher bandwidth AOMs are available.
3. RESULTS AND DISCUSSION
Figures

Fig. 3. (a) Measured SNRs and bit-loading of different OFDM subcarriers. (b) Constellation diagrams of 16, 32, 64, 128, 256-QAM of red LD.
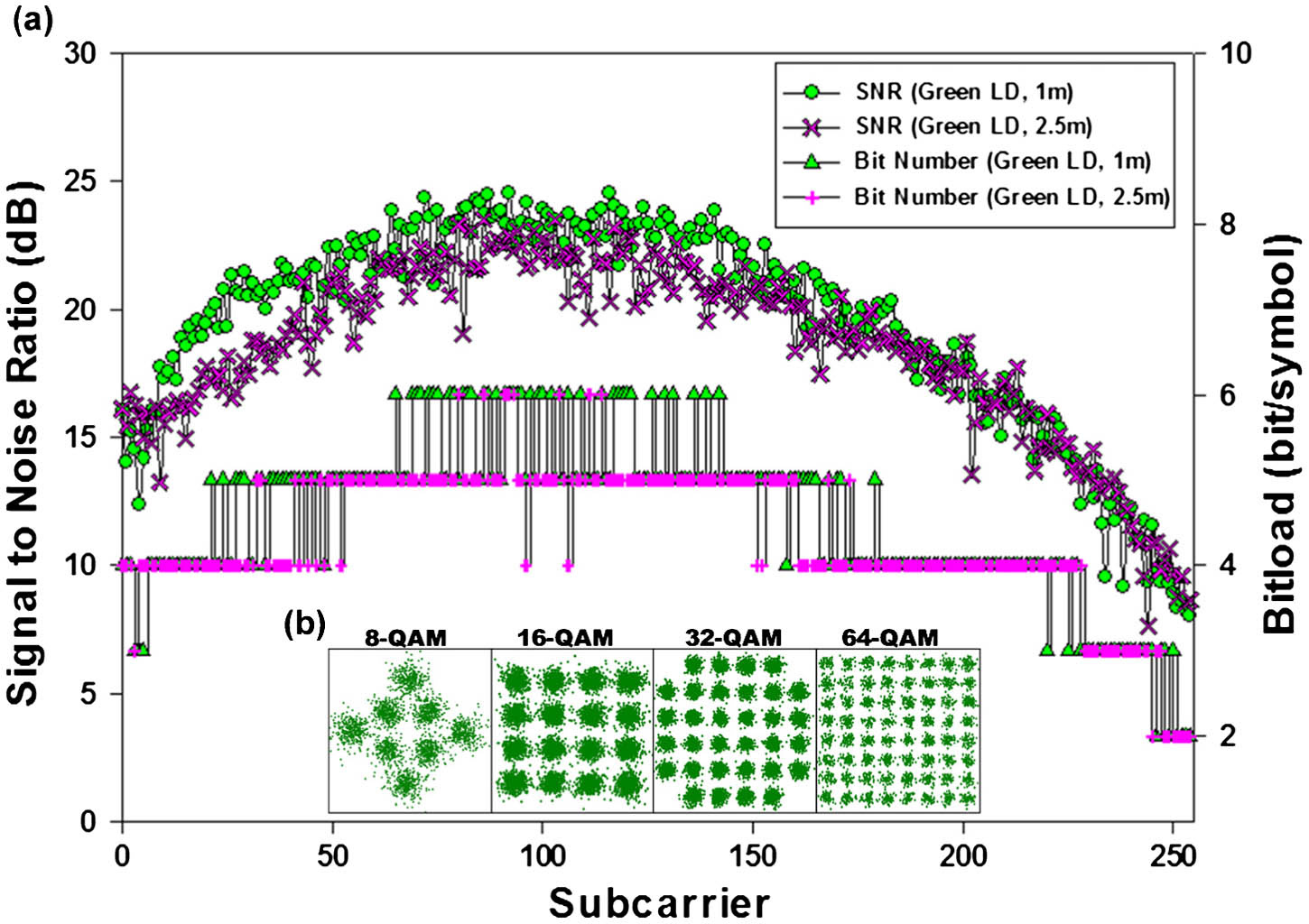
Fig. 4. (a) Measured SNRs and bit-loading of different OFDM subcarriers. (b) Constellation diagrams of 8, 16, 32, 64-QAM of green LD.
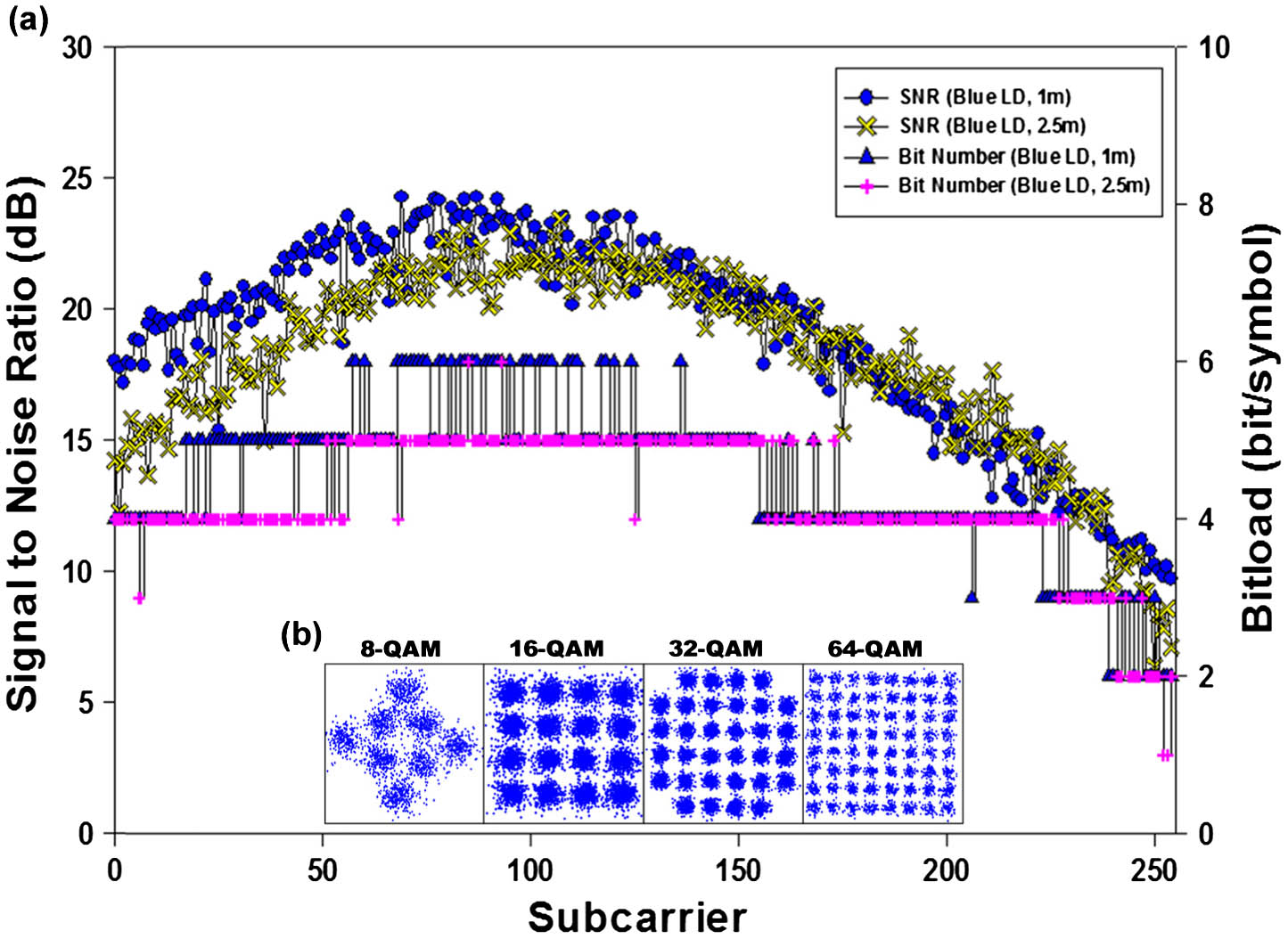
Fig. 5. (a) Measured SNRs and bit-loading of different OFDM subcarriers. (b) Constellation diagrams of 8, 16, 32, 64-QAM of blue LD.
Then, we evaluate the cross talk of the tricolor RGB channel. Figures
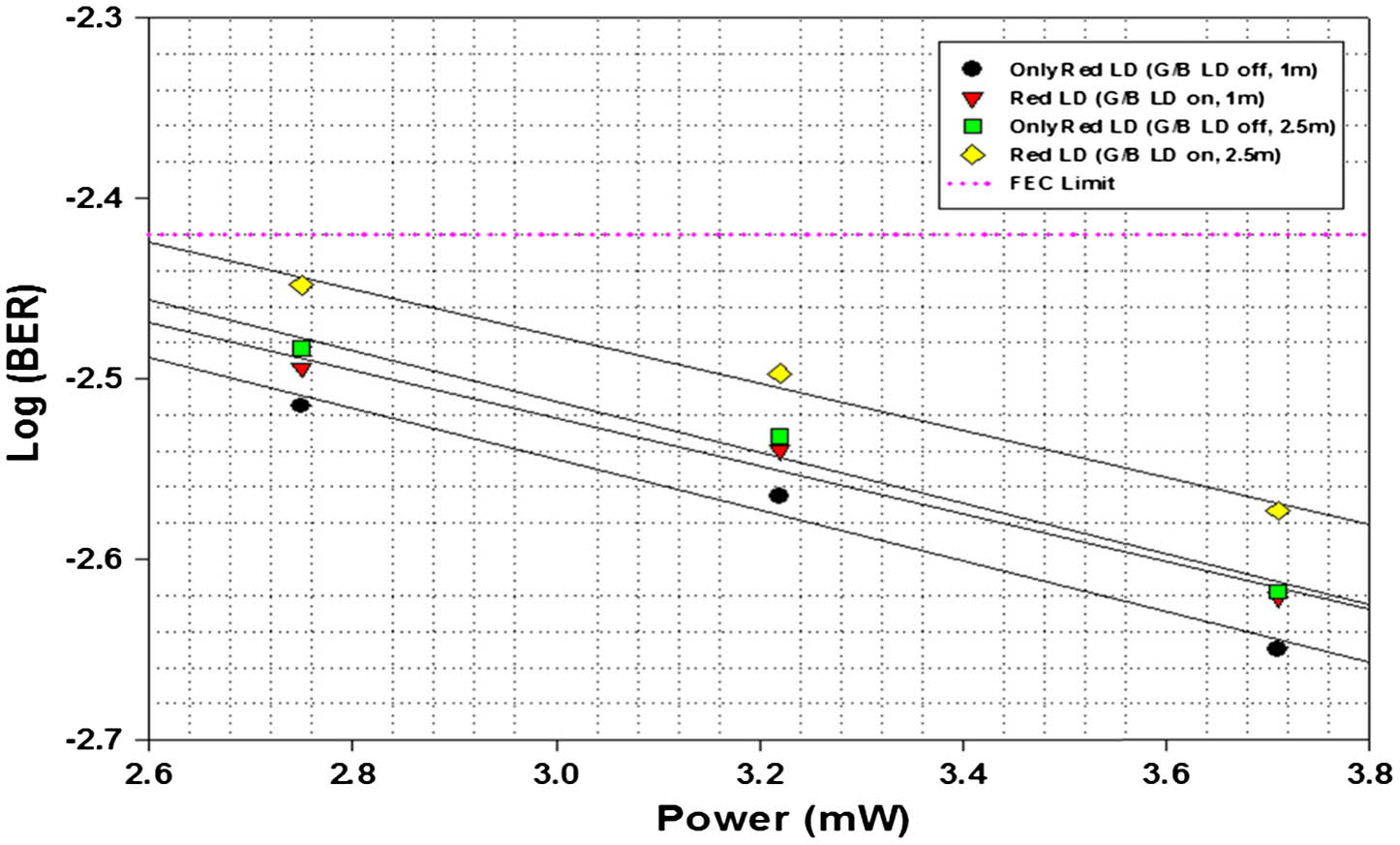
Fig. 6. Measured log(BER) of red LD at different received powers and influence on red LD with G/B LDs off and G/B LDs on.

Fig. 7. Measured log(BER) of green LD at different received powers and influence on green LD with R/B LDs off and R/B LDs on.

Fig. 8. Measured log(BER) of blue LD at different received powers and influence on blue LD with R/G LDs off and R/G LDs on.
Figure
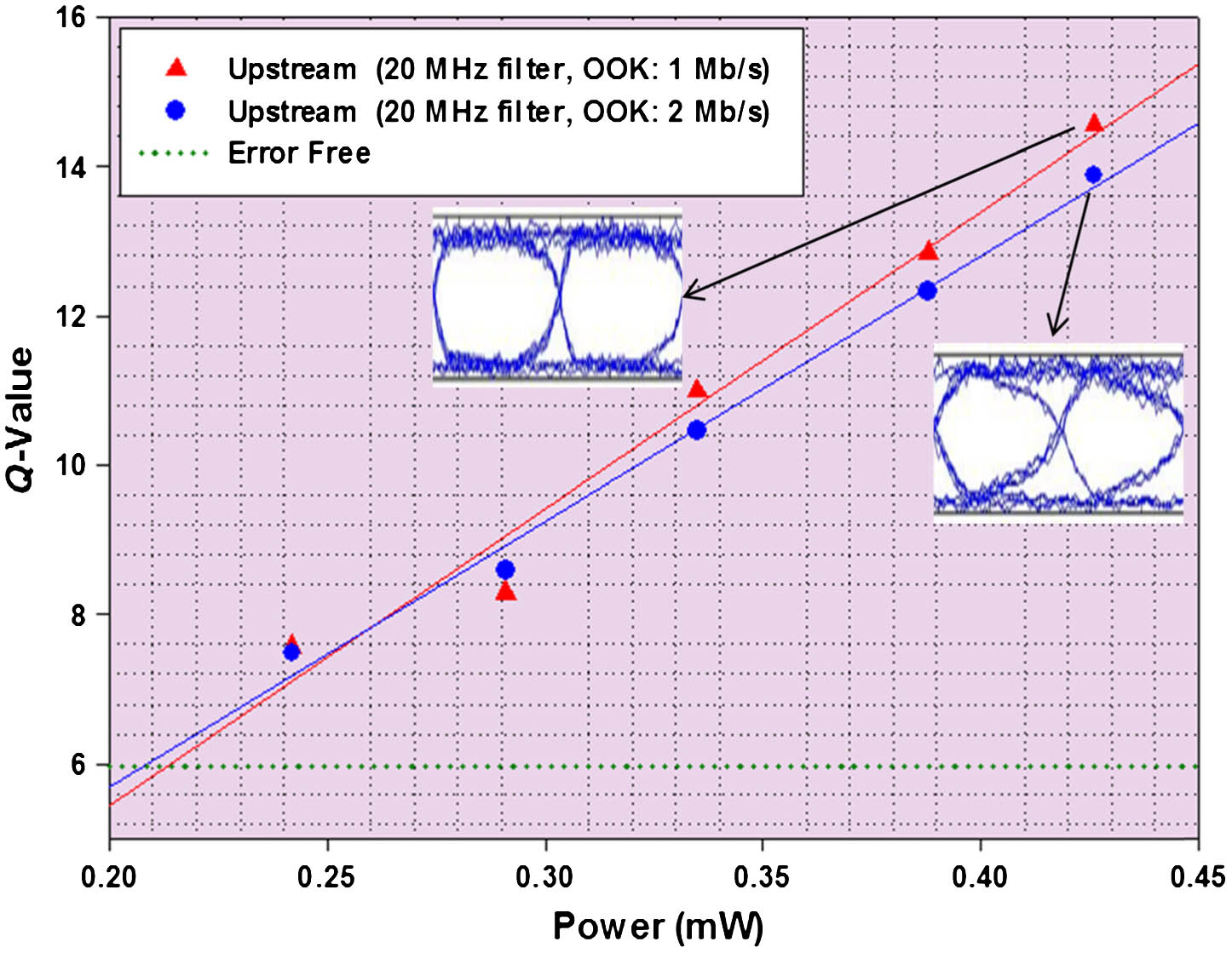
Fig. 9. Measured -value of the remodulated upstream OOK signal (red points: 1 Mbit/s, blue points: 2 Mbit/s) against different received optical powers when different LPFs are used.
4. CONCLUSION
In this work, we proposed and experimentally demonstrated a recorded 20.231 Gbit/s (R: 11.023 Gbit/s; G: 4.618 Gbit/s; B: 4.59 Gbit/s) 1-m bidirectional tricolor RGB LD-based VLC system supporting signal remodulation. As the LD sources are located at the CO, the laser wavelength and temperature managements at the cost-sensitive client side are not needed. The cross talks introduced by adjacent color channels in the RGB LD-based transmissions were evaluated. 2 Mbit/s remodulated error-free OOK upstream signal was achieved. The data rate was limited by the AOM available; higher upstream data rates are possible. This work is the first demonstration of
[2] C. W. Chow, C. H. Yeh, Y. Liu, Y. F. Liu. Digital signal processing for light emitting diode based visible light communication. IEEE Photon. Soc. Newsl., 2012, 26: 9-13.
Liang-Yu Wei, Chin-Wei Hsu, Chi-Wai Chow, Chien-Hung Yeh. 20.231 Gbit/s tricolor red/green/blue laser diode based bidirectional signal remodulation visible-light communication system[J]. Photonics Research, 2018, 6(5): 05000422.