基于三角波调制的免标定气体浓度测量方法研究
下载: 967次
1 引言
可调谐二极管激光吸收光谱(TDLAS)技术中的波长调制光谱法是一种常用的气体参数检测方法,具有信噪比高、灵敏度高、响应速度快、非侵入等优点,适合用于气体的温度、组分浓度等参数的在线测量[1-3]。波长调制技术一般使用低频扫描叠加高频调制信号对分布反馈(DFB)激光器的波长进行周期性调谐,通过提取吸收在较高调制频率分量上的强度信息,降低背景噪声对测量的影响,从而获得高信噪比的吸收信号[4-5]。传统的波长调制法一般采用不同浓度的标准气体标定的方式[6]。由于实际环境中待测气体的组分与标准气体的组分不同,并可能随时变化,因此,完全根据标定方式获取的气体浓度的测量值会存在一定误差。
近些年出现了免标定波长调制技术。Rieker等[7-8]从理论上推导了谐波表达关系式,测量了DFB激光器光强和波长的响应特性,然后将仿真得到的一次谐波归一化二次谐波信号(
本文重点研究了基于三角波调制的免标定波长调制方法的实现,建立了三角波调制方式下DFB激光器的频率响应模型,将三角波调制波形简化为若干正弦波叠加的形式,通过拟合实测的标准具信号来提取模型的参数。选用中心波长为1653 nm的DFB激光器实现了三角波调制方式下CH4气体浓度的免标定测量。
2 基本测量原理
根据Beer-Lambert定律,当一束激光穿过待测气体时,一部分光强被气体吸收,吸收率可表示为
式中
式中
对于不同的气体和温度范围,系数

图 1. 免标定波长调制浓度反演算法流程图
Fig. 1. Flow chart of calibration-free wavelength modulation inversion algorithm for concentration
在测量气体浓度之前需要预先确定
式中
按照同样的方式可得到仿真的
3 三角波调制方式下DFB激光器频率响应模型的建立
由上节可知,实现免标定测量的关键在于预先获得准确的DFB激光器频率响应模型。DFB激光器频率响应特性的本质是对注入电流的响应,注入电流调制机制在于载流子浓度和电流热效应的双重作用[18],上述两种作用均会引起DFB激光器出光波长(频率)的线性和非线性变化[19]。根据该特性,假设正弦波扫描叠加三角波调制方式下的DFB激光器的频率响应模型可用(13)式表示:
式中
式中
表 1. 傅里叶展开式系数Ai及其相对A1的比例Ki
Table 1. Fourier expanding coefficients Ai and ratio (Ki) of Ai to A1
|
设定周期为1 s,
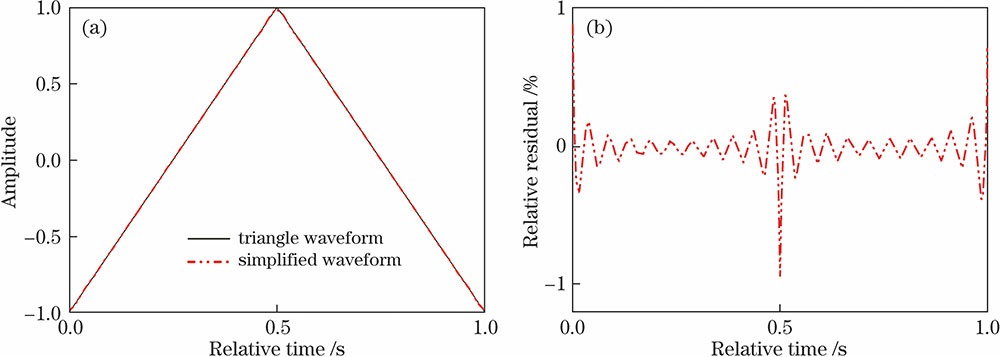
图 2. (a)三角波波形与简化波形的振幅;(b)相对残差
Fig. 2. (a) Amplitudes of triangle waveform and simplified waveform; (b) relative residual
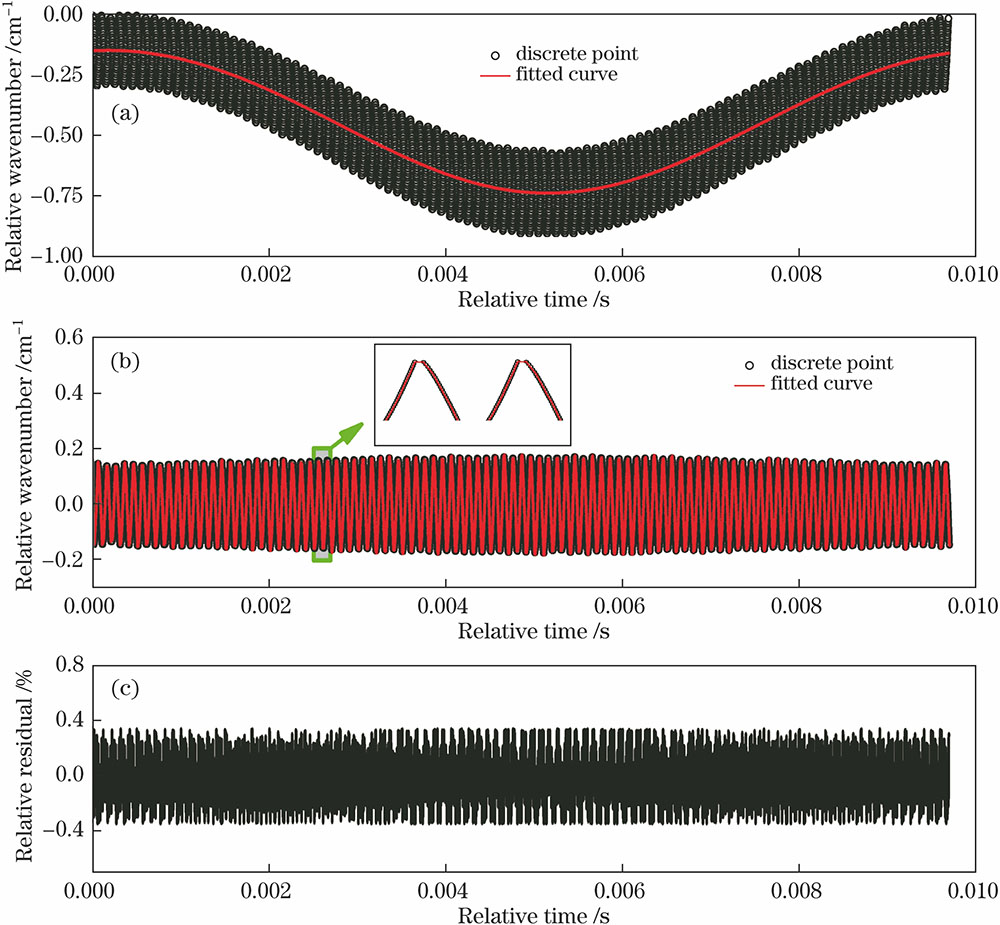
图 3. 正弦波扫描三角波调制的DFB激光器频率响应测量信号和拟合曲线。(a)激光器频率响应的离散点和扫描项的拟合曲线;(b)调制项、耦合项对应的频率响应的离散点和拟合曲线;(c)调制项、耦合项离散点与拟合曲线的相对残差
Fig. 3. Measured and fitted frequency response of sine wave scanning superimposed by triangular wave modulation for DFB laser. (a) discrete points of laser frequency response and fitted curve of scanning term; (b) discrete points and fitted curve of frequency response of modulation term and coupling term; (c) relative residual between discrete points and fitted curves of modulation term and coupling term
表 2. V(t)拟合的参数
Table 2. Parameters of fitting results of V(t)
|
经简化,正弦波扫描叠加三角波调制方式下的DFB激光器频率响应模型可表示为
4 实验结果与分析
为了进一步验证上述模型的正确性,利用上述模型结合免标定算法对CH4气体浓度进行测量。实验装置示意图如
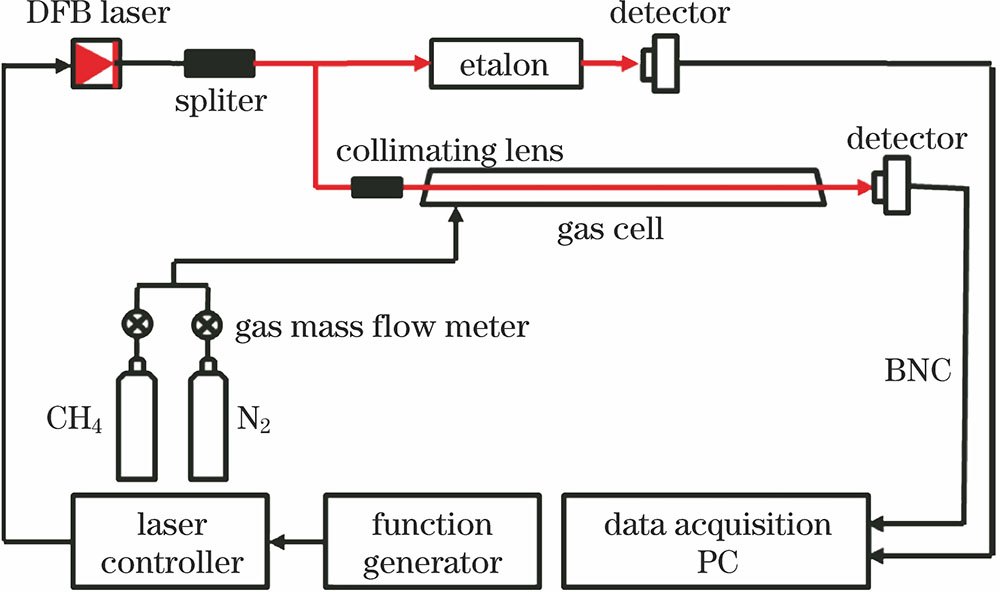
图 4. CH4浓度免标定测量实验装置示意图
Fig. 4. Schematic of experimental apparatus for measuring CH4 concentration without calibration
RIGOL DG1062型函数发生器将扫描叠加调制的电信号输入到LDC501型激光控制器中,通过注入电流调制对中心波长在1653 nm附近的DFB激光器的输出波长进行调谐。DFB激光器输出的激光被一个1×2光纤分束器分为两路:第一路激光经F240APC型准直器准直后穿过长度为20 cm的石英气池,透射光强被Thorlabs PDA10CS-EC型光电探测器接收并转换为电信号;另一路激光通过马赫-曾德尔干涉仪后被光电探测器接收,用于测量标准具信号。两路电信号通过卡扣连接器(BNC)的连接线传输,并被NI PXIe-5170R型高速数据采集卡采集。
所选CH4的吸收线中心位于6046.95 cm-1附近,由3条紧邻的吸收谱线组成,主要参数[20]见
表 3. 所选谱线的主要参数
Table 3. Main parameters of selected spectral lines
|
Iguchi[14]等验证了三角波调制和正弦波调制的最佳调制系数分别为2.8和2.2。实验采用正弦波扫描,扫描频率为100 Hz,三角波调制频率为10 kHz,最佳调制系数下对应的调制深度为0.188 cm-1。此外,使用正弦波调制的信号作为对比,正弦波调制频率为10 kHz,最佳调制系数下对应的调制深度为0.148 cm-1。背景光强信号和气体吸收光强信号的采样率为1 MS·s-1,标准具信号的采样率为125 MS·s-1。
使用物质的量分数为99.999%的高纯N2和4.00%的CH4标准气体(余气为N2,相对不确定度为1.5%、置信因子为2)配制实验所需的不同浓度的CH4气体。通过调节Horiba Z500型质量流量计的出口流量实现配气,相应的配气参数见
表 4. 不同浓度CH4的配气列表(CH4/N2)
Table 4. Distribution list for different mole fractions of CH4(CH4/N2)
|
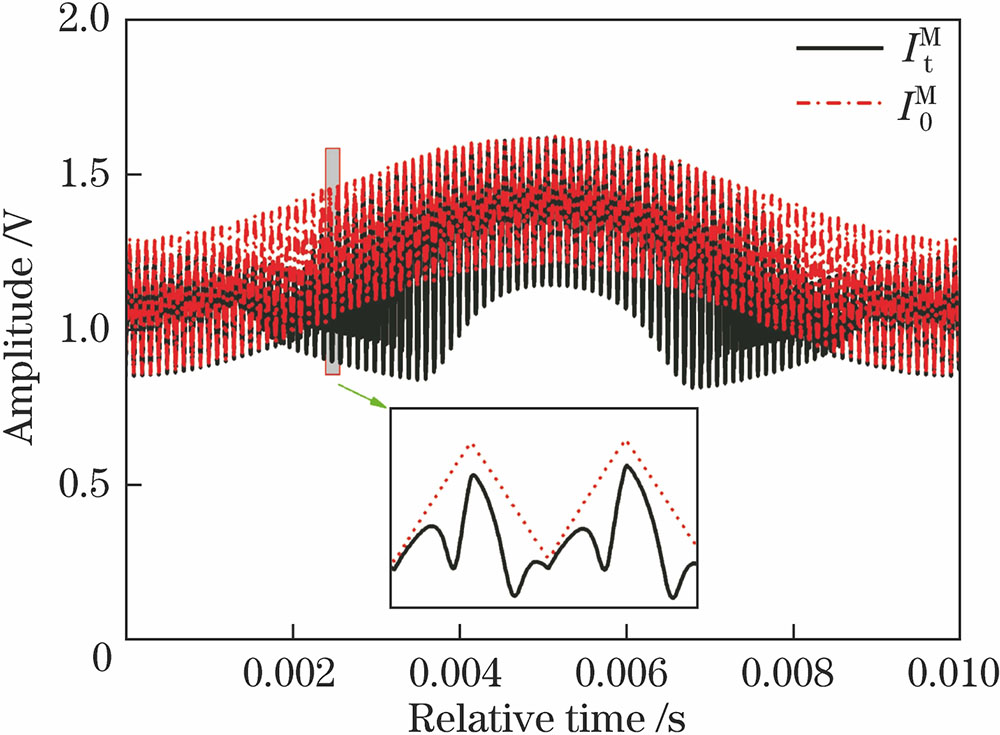
图 5. 背景光强信号和4.00%CH4的吸收信号
Fig. 5. Background light intensity signal and absorption signal of CH4 with mole fraction of 4.00%
最佳调制参数下三角波与正弦波调制方式的二次谐波信号
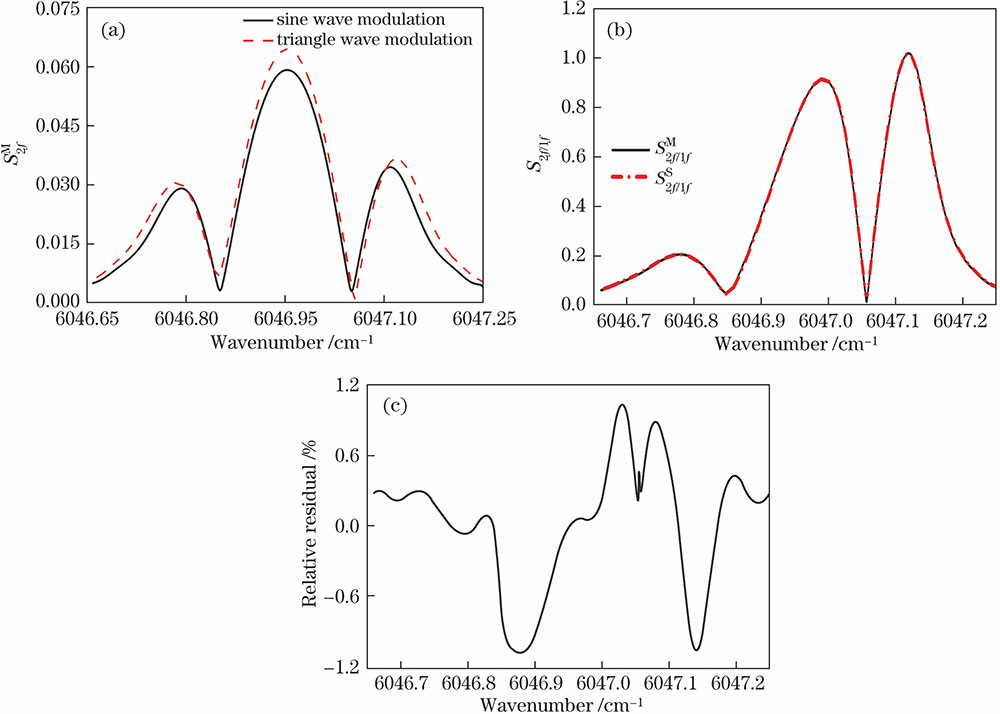
图 6. (a)最佳调制参数下三角波调制与正弦波调制的二次谐波信号SM2f;(b) 4.00%CH4三角波调制实验SM2f/1f信号和仿真SS2f/1f信号;(c)仿真SS2f/1f与实验SM2f/1f信号拟合的相对残差
Fig. 6. (a) Second harmonic signals of SM2f for triangle wave modulation and sine wave modulation at optimal modulation parameters; (b) experimental SM2f/1f and simulated SS2f/1f for CH4 with mole fraction of 4.00%; (c) relative residual between simulated SS2f/1f and experimental S2f/1fM
当配制CH4的物质的量分数为4.00%时,连续测量600 s,测得的结果如

图 7. (a) 4.00%CH4连续600 s的测量浓度;(b) Allan方差
Fig. 7. (a) Measured mole fraction of 4.00%CH4 in 600 s; (b) Allan variance
在同一浓度下进行多组重复实验,测量结果如
为了验证该免标定方法在复杂测量环境中的适用性,使用N2、高纯CO2(纯度不低于99.98%)与物质的量分数为4.00%的CH4标准气体配制不同浓度的CH4,配气参数见
表 5. 不同浓度CH4的配气列表(CH4/N2/CO2)
Table 5. Distribution list for different mole fractions of CH4 (CH4/N2/CO2)
|
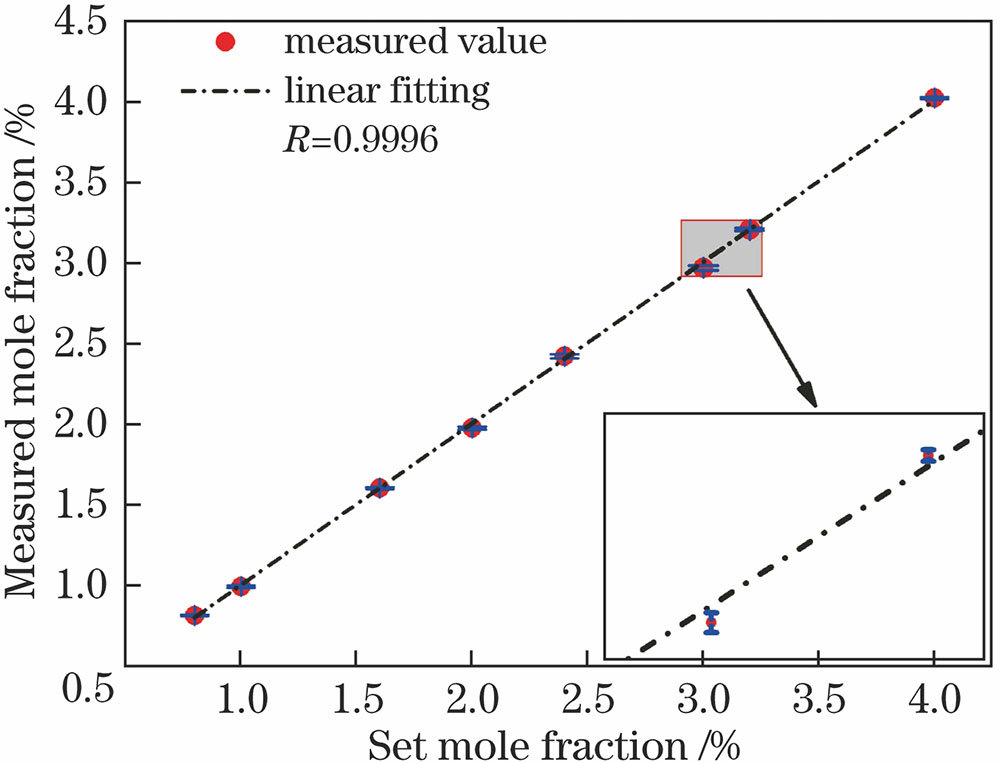
图 8. CH4/N2混合气体中CH4的测量浓度
Fig. 8. Measured mole fraction of CH4 in mixture gas of CH4 and N2
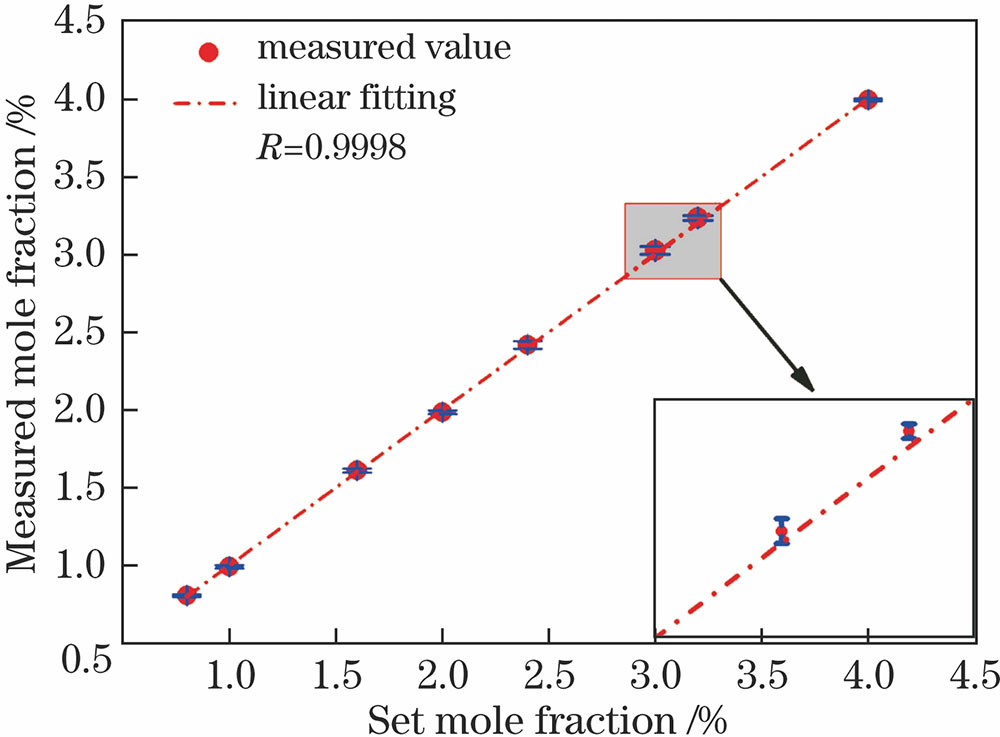
图 9. CH4/N2/CO2混合气体中CH4的测量浓度
Fig. 9. Measured mole fraction of CH4 in mixture gas of CH4, N2 and CO2
5 结论
研究了基于三角波调制的免标定气体浓度测量方法,建立了DFB激光器在三角波调制方式下的频率响应模型,实现了免标定三角波调制方式下CH4气体浓度的准确测量。三角波调制方式比常用的正弦波调制方式具有更高的灵敏度。该方法不仅可用于对气体浓度的测量,稍加改进后可以结合双线测温法对气体的温度和压力等参数进行免标定测量。
[1] 屈东胜, 洪延姬, 王广宇, 等. 基于扫描波长调制光谱的气体质量流量测量方法研究[J]. 中国激光, 2016, 43(9): 0904006.
屈东胜, 洪延姬, 王广宇, 等. 基于扫描波长调制光谱的气体质量流量测量方法研究[J]. 中国激光, 2016, 43(9): 0904006.
Qu Dongsheng, Hong Yanji, Wang Guangyu, et al. Study on mass flux measurement methods of gas based on scanning wavelength modulation spectroscopy[J]. Chinese J Lasers, 2016, 43(9): 0904006.
[2] 高光珍, 蔡廷栋. 1570 nm附近多模二极管激光吸收光谱CO浓度测量[J]. 光学学报, 2016, 36(5): 0530002.
高光珍, 蔡廷栋. 1570 nm附近多模二极管激光吸收光谱CO浓度测量[J]. 光学学报, 2016, 36(5): 0530002.
[3] 王尹秀, 陈东, 贾兆丽, 等. CO近红外高分辨吸收光谱特性的实验研究[J]. 激光与光电子学进展, 2015, 52(11): 113004.
王尹秀, 陈东, 贾兆丽, 等. CO近红外高分辨吸收光谱特性的实验研究[J]. 激光与光电子学进展, 2015, 52(11): 113004.
[6] DongL, LiC, YuY, et al. Compact TDLAS based sensors using interband cascade lasers for CH2O and CH4 trace gas measurements[C]. Optics and Photonics forEnergy and theEnvironment, Suzhou, China, 2015: ETuA. 5.
DongL, LiC, YuY, et al. Compact TDLAS based sensors using interband cascade lasers for CH2O and CH4 trace gas measurements[C]. Optics and Photonics forEnergy and theEnvironment, Suzhou, China, 2015: ETuA. 5.
[13] O'Haver T C. Epstein M S, Zander A T. Waveform effects in wavelength modulation spectrometry[J]. Analytical Chemistry, 1977, 49(49): 458-461.
O'Haver T C. Epstein M S, Zander A T. Waveform effects in wavelength modulation spectrometry[J]. Analytical Chemistry, 1977, 49(49): 458-461.
[16] Gamache RR, KennedyS, HawkinsR, et al. Total internal partition sums for molecules in the terrestrial atmosphere[J]. Journal of Molecular Structure, 2000, 517/518: 407- 425.
Gamache RR, KennedyS, HawkinsR, et al. Total internal partition sums for molecules in the terrestrial atmosphere[J]. Journal of Molecular Structure, 2000, 517/518: 407- 425.
[17] Jannson TP, Aye TM, Hirsh JW, et al. High finesse holographic fabry-perot etalon and method of fabricating:US5293272[P]. 1994-03-08.
Jannson TP, Aye TM, Hirsh JW, et al. High finesse holographic fabry-perot etalon and method of fabricating:US5293272[P]. 1994-03-08.
[18] GhafourishirazH. Distributed feedback laser diodes and optical tunable filters distributed feedback laser diodes and optical tunable filters[M/OL]. [2017-04-18].http:∥download.e-bookshelf.de/download/0000/5799/75/L-G-0000579975-0002360038.pdf.
GhafourishirazH. Distributed feedback laser diodes and optical tunable filters distributed feedback laser diodes and optical tunable filters[M/OL]. [2017-04-18].http:∥download.e-bookshelf.de/download/0000/5799/75/L-G-0000579975-0002360038.pdf.
Article Outline
汪步斌, 周宾, 王浩, 熊涌泉, 王一红, 祝仰坤, 王式民. 基于三角波调制的免标定气体浓度测量方法研究[J]. 光学学报, 2017, 37(9): 0930003. Bubin Wang, Bin Zhou, Hao Wang, Yongquan Xiong, Yihong Wang, Yangkun Zhu, Shimin Wang. Study on Calibration-Free Measurement Method for Gas Concentration Based on Triangular Wave Modulation[J]. Acta Optica Sinica, 2017, 37(9): 0930003.