30CrMnSiA和30CrMnSiNi2A高强钢激光熔覆修复后的组织特征与力学性能
下载: 1027次
1 引言
30CrMnSiA钢和30CrMnSiNi2A钢是常用的高强度结构钢,被广泛用于制造重要的承力构件,如轴类、螺栓和飞机起落架等。由于在服役过程中需要承受较高的应力,高强钢构件对冲击、腐蚀、磨损和疲劳等造成的微裂纹具有很高的敏感性,因此,高强钢修复层的韧性对于金属构件的运行成本和安全性至关重要。
30CrMnSiA钢和30CrMnSiNi2A钢的焊接性较差,采用传统方法焊接时需要进行严格的焊前预热和层间温度控制。此外,传统焊接方法的热输入大,易导致高强钢构件发生变形或开裂等问题[1-2]。作为一种新型的表面改性技术,激光熔覆利用激光束快速加热和冷却的特点,在修复件表面熔覆同种或异种金属粉末。该技术具有熔覆组织致密、界面结合强度高、构件变形小和工艺简单等优点[3]。采用激光熔覆技术对高强钢构件进行修复,可以大幅缩短维修周期,延长构件的使用寿命。
近年来,国内外对300M[4-10]、AerMet 100[11-13]、AISI 4340[14-15]、HSLA-100[16]高强钢的激光熔覆修复进行了研究。在高强钢的同种修复方面,Rahman Rashid等[7-8]研究了激光沉积方向对300M修复件力学性能的影响,结果发现:修复件力学性能的各向异性归因于组织中回火马氏体相和马氏体相的存在;激光原位热处理可以减少涂层中硬质马氏体相的含量,使回火马氏体相的分布更均匀。Barr等[9]研究了不连续和连续激光熔覆的热累积对微观组织的影响,得到了不同原位回火机制下熔覆层的硬度与不同状态马氏体相含量之间的关系。Lourenço等[11]和Walker等[12]研究了激光熔覆修复AerMet 100钢的疲劳行为,结果发现,熔覆层和热影响区中存在的残余压应力有利于疲劳寿命的提高,但沿熔覆层内柱状晶晶界断裂的模式降低了修复件的疲劳寿命,而且熔覆后进行热处理不能有效改善疲劳寿命。对于高强钢的异种材料修复,文献中已有较多报道,如在300M钢表面激光熔覆AISI 420粉末[4]、在300M钢表面激光熔覆AerMet 100粉末[5-6]以及在AISI 4340钢表面激光熔覆AerMet 100粉末[14]等。熔覆材料的碳含量(碳的质量分数≤0.25%)小于基板的碳含量(碳的质量分数约为0.4%),低碳含量熔覆层能够提升高强钢修复区的可焊性,增强熔覆层的韧性,但其强度低于基体的强度。因此,对于高强钢的激光熔覆修复,熔覆材料的选择和熔覆层组织的调控对于修复试样强韧性的提升是十分重要的。
对于30CrMnSiA和30CrMnSiNi2A高强钢的激光熔覆修复,目前研究人员主要采用铁基自熔性粉末[17]、18CrMoA[18]、1Cr15Ni4Mo3[19]和GQ001[20]等合金粉末,实现熔覆层和基体的强韧匹配。但30CrMnSiA和30CrMnSiNi2A高强钢中的碳含量不同,在一定温度下,基体中的碳元素会扩散至熔覆层/基体界面,导致界面出现增碳脆化现象,不利于服役性能的稳定[13,21]。因此,选择与这两种钢基体成分相同或相近的合金粉末(如30CrMnSiA合金粉末)进行激光熔覆,将有利于修复件的长期服役性能。但30CrMnSiA合金的焊接裂纹敏感性较强[22-23],而且以30CrMnSiA合金粉末作为熔覆材料进行激光修复的研究还未见公开报道。
本文以30CrMnSiA合金粉末作为熔覆材料,在30CrMnSiA和30CrMnSiNi2A高强钢表面进行了多层多道激光熔覆,通过调控激光熔覆工艺参数,研究了基体、热影响区和熔覆层的微观组织,并对熔覆层试样和基体的力学性能、断口形貌进行了分析,以期为30CrMnSiA合金粉末在30CrMnSiA和30CrMnSiNi2A钢激光熔覆修复中的应用提供参考。
2 实验材料和方法
2.1 材料制备
实验所用合金粉末为30CrMnSiA粉末,其化学成分如
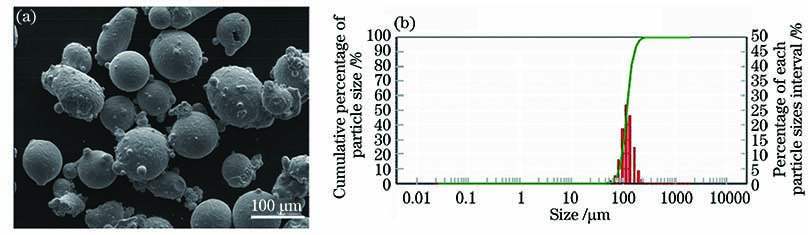
图 1. 30CrMnSiA合金粉末的形貌和尺寸。(a) SEM形貌;(b)粒径分布
Fig. 1. Morphology and size of 30CrMnSiA alloy powders. (a) SEM morphology; (b) particle size distribution
表 1. 30CrMnSiA合金粉末的化学成分
Table 1. Chemical composition of 30CrMnSiA alloy powders
|
实验所用基板分别为30CrMnSiA钢和30CrMnSiNi2A钢,两种钢的尺寸均为120 mm×60 mm×10 mm,在其表面开修复缺口(尺寸为10 mm×60 mm×2.5 mm),如
实验所用激光器为Laserline LDF-8000 型高功率激光器,其最大功率为8 kW,光斑尺寸为4 mm。采用同轴送粉形式进行激光熔覆,整个熔覆过程在高纯氩气保护下进行,熔覆示意图如
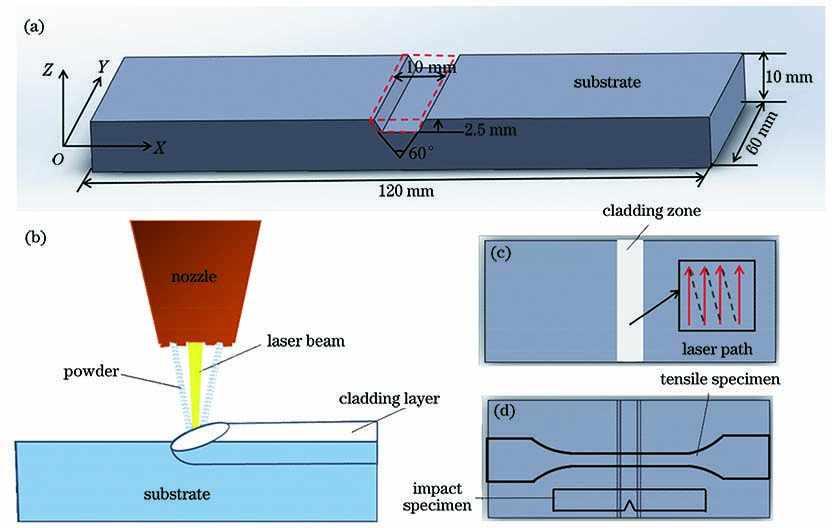
图 2. 激光熔覆及力学性能测试试样的取样示意图。(a)带缺口的熔覆基板;(b)激光熔覆加工示意图;(c)激光扫描方式;(d)力学性能测试试样的取样方式
Fig. 2. Schematics of laser-cladding and sampling of mechanical properties test samples. (a) Substrate with notch for lase cladding; (b) diagram of laser-cladding; (c) laser scanning pattern; (d) sampling method of mechanical properties test samples
单层多道和多层多道熔覆实验过程中采用的工艺参数如下:激光功率为2.1 kW,激光扫描速度为9 mm/s,送粉速率为10 g/min,光斑搭接率为40%,离焦量为0 mm,送粉气流量为10 L/min,保护气流量为20 L/min。在进行多层多道熔覆时,熔覆6层后,将试样空冷至室温。
2.2 组织和性能表征
激光熔覆完成后,垂直于熔覆方向,切割出尺寸为20 mm×10 mm×10 mm的金相试样,依次对金相试样进行砂纸打磨、机械抛光和腐蚀处理,腐蚀剂为4%硝酸酒精。
采用ICAP-7600电感耦合等离子体-原子发射光谱仪对粉末成分进行分析;采用S3500激光粒度仪对粉末的粒径分布进行分析;采用Zeiss-AxioCam MRc5光学显微镜(OM)和TESCAN-LYRA3扫描电子显微镜(SEM)对熔覆层的微观组织进行表征;采用Zwick/Roell Z100维氏显微硬度计对熔覆层的显微硬度进行表征,测试载荷为1.96 N,保载时间为15 s,相邻压痕间距为200 μm;采用Zwick/Roell Z100拉伸试验机进行拉伸测试,采用冲击试验机进行冲击测试,冲击能量为300 J,每组实验进行三次,取三次实验的平均值作为最终的测试值。
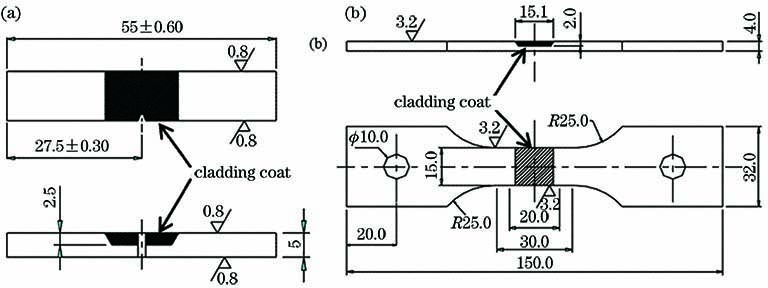
图 3. 拉伸试样和冲击试样示意图。(a)冲击试样;(b)拉伸试样
Fig. 3. Schematics of tensile samples and impact samples. (a) Impact sample; (b) tensile sample
3 分析与讨论
3.1 宏观形貌及微观组织
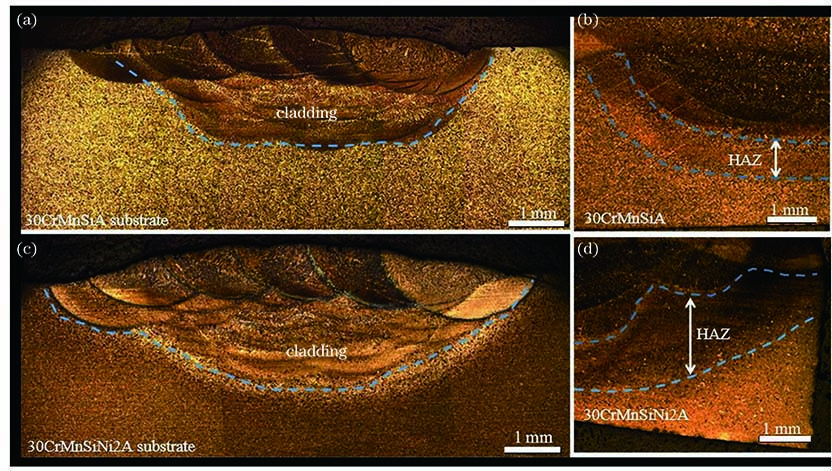
图 4. 两种高强钢经多层多道激光熔覆后的横截面宏观形貌。(a) 30CrMnSiA基体;(b) 30CrMnSiA热影响区;(c) 30CrMnSiNi2A基体;(d) 30CrMnSiNi2A热影响区
Fig. 4. Cross-section macroscopic morphology of two high-strength steels after multi-layer laser-cladding. (a) 30CrMnSiA substrate; (b) HAZ on 30CrMnSiA substrate; (c) 30CrMnSiNi2A substrate; (d) HAZ on 30CrMnSiNi2A substrate
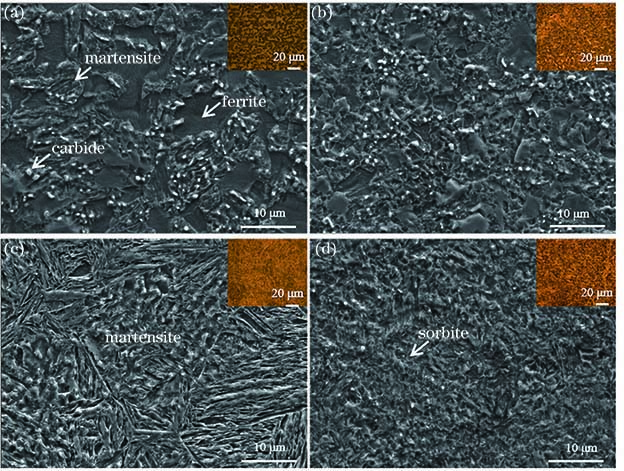
图 5. 两种高强钢基体和热影响区的微观组织。(a) 30CrMnSiA基体;(b) 30CrMnSiA热影响区;(c) 30CrMnSiNi2A基体;(d) 30CrMnSiNi2A热影响区
Fig. 5. Microstructures of two high-strength steel substrates and HAZ. (a) 30CrMnSiA substrate; (b) HAZ on 30CrMnSiA substrate; (c) 30CrMnSiNi2A substrate; (d) HAZ on 30CrMnSiNi2A substrate

图 6. 30CrMnSiA基体上多层熔覆层的微观组织。(a)第1层;(b)第2层;(c)第3层;(d)第4层;(e)第5层;(f)第6层(盖面层)
Fig. 6. Microstructures of different cladding layers on 30CrMnSiA substrate. (a) The first layer; (b) the second layer; (c) the third layer; (d) the fourth layer; (e) the fifth layer; (f) the sixth layer (cap layer)
1) 30CrMnSiNi2A基体上熔覆层的组织为马氏体;进行多层激光熔覆后,熔覆层回火分解成索氏体,索氏体含量明显增加;盖面层组织主要为马氏体和索氏体。
2) 30CrMnSiNi2A热影响区的宽度远大于30CrMnSiA,出现了重新淬硬的粗晶马氏体,大部分马氏体发生回火转变为索氏体;而30CrMnSiA热影响区在激光热源的作用下生成了大量马氏体组织,但未出现粗晶马氏体。

图 7. 30CrMnSiNi2A基体上多层熔覆层的微观组织。(a)第1层;(b)第2层;(c)第3层;(d)第4层;(e)第5层;(f)第6层(盖面层)
Fig. 7. Microstructures of different cladding layers on 30CrMnSiNi2A substrate. (a) The first layer; (b) the second layer; (c) the third layer; (d) the fourth layer; (e) the fifth layer; (f) the sixth layer (cap layer)
出现上述差异的原因可能是30CrMnSiNi2A钢相较于30CrMnSiA钢具有更低的热导率[25]。室温下,30CrMnSiA钢和30CrMnSiNi2A钢的热导率分别为38 W/(m·℃)[26]和25.7 W/(m·℃)[27]。30CrMnSiNi2A钢的热导率更低,因此,在多层激光熔覆过程中的热累积更多,热影响区在更高的温度下重新淬硬生成粗晶马氏体;同时,在多层熔覆的热循环作用下,马氏体经历了多次分解。
3.2 熔覆层的力学性能

图 8. 高强钢基体上熔覆层试样横截面的金相图和显微硬度分布。(a) 30CrMnSiA,金相图;(b) 30CrMnSiNi2A,金相图;(c) 30CrMnSiA,显微硬度分布;(d) 30CrMnSiNi2A,显微硬度分布
Fig. 8. Metallography image and microhardness distribution in cross-section of cladding samples on two high strength steel substrates. (a) 30CrMnSiA, metallography image; (b) 30CrMnSiNi2A, metallography image; (c) 30CrMnSiA, microhardness distribution; (d) 30CrMnSiNi2A, microhardness distribution
此外,与基体的硬度值相比,30CrMnSiA热影响区(HAZ)的软化现象不明显,而30CrMnSiNi2A热影响区存在明显的软化区。这是因为30CrMnSiNi2A基板的热导率较低,导致基板上热量的积累程度较大,热影响区的回火程度较高,故而热影响区的硬度与基板硬度值(约为640 HV)相比,降幅明显。

图 9. 两种基体及熔覆层试样的室温力学性能。(a)冲击韧性;(b)拉伸性能
Fig. 9. Mechanical properties of two substrates and cladding samples at room-temperature. (a) Impact toughness; (b) tensile property
由

图 10. 不同基体上熔覆层试样的冲击断口形貌。(a)(c) 30CrMnSiA基体上熔覆层试样的断口以及裂纹源处的局部放大图;(b)(d) 30CrMnSiNi2A基体上熔覆层试样的断口以及裂纹源处的局部放大图
Fig. 10. Impact fracture morphologies of cladding samples on different substrates. (a)(c) Fracture of cladding sample on 30CrMnSiA substrate and partially enlarged crack source; (c)(d) fracture of cladding sample on 30CrMnSiNi2A substrate and partially enlarged crack source
由30CrMnSiA基体上熔覆层试样拉伸断口的横截面形貌可以看出,拉伸断口上有两处起裂位置,分别如
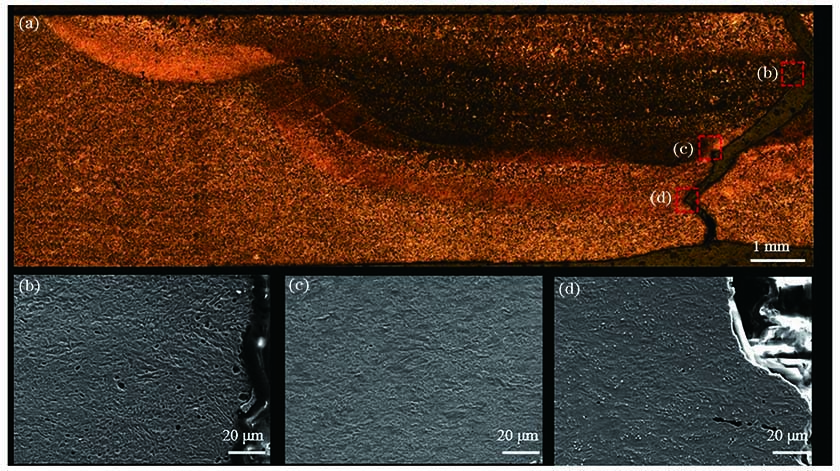
图 11. 30CrMnSiA基体上熔覆层试样拉伸断口的横截面形貌。(a)横截面形貌;(b)~(d)局部放大图
Fig. 11. Tensile fracture in cross-section of cladding sample on 30CrMnSiA substrate. (a) Cross-section image; (b)--(d) partially enlarged images
由30CrMnSiNi2A基体上熔覆层试样拉伸断口的横截面形貌可以看出,熔覆层内含有较多索氏体,如

图 12. 30CrMnSiNi2A基体上熔覆层试样拉伸断口的横截面形貌。(a)横截面;(b)~(d)局部区域放大图
Fig. 12. Tensile fracture in cross-section of cladding sample on 30CrMnSiNi2A substrate. (a) Cross-section image; (b)--(d) partially enlarged images
4 结论
以30CrMnSiA合金粉末作为熔覆材料,对30CrMnSiA钢和30CrMnSiNi2A钢进行了多层多道激光熔覆修复,然后对熔覆层和热影响区的组织、力学性能进行表征,得出以下结论:
1) 30CrMnSiA基体主要为铁素体和马氏体均匀分布的复相组织,热影响区主要为细晶马氏体和少量小块状铁素体;30CrMnSiNi2A基体为马氏体,热影响区为索氏体和粗晶马氏体。
2) 采用相同的熔覆材料和工艺参数进行激光熔覆,两种基体上的多层熔覆层组织存在一定差异,表现为各层中索氏体的含量不同。30CrMnSiA基体上的第1~3层熔覆层主要为索氏体组织,第4~6层熔覆层主要为马氏体和少量回火索氏体,其中盖面层主要为马氏体;30CrMnSiNi2A基体上的熔覆层回火分解成的索氏体含量明显增加。这种组织差异推测是由30CrMnSiNi2A钢的热导率更低,在激光熔覆过程中会导致更多的热累积造成的。
3) 在力学性能上,两种基体上的多层熔覆层存在明显差异:30CrMnSiA基体上熔覆层的硬度要远大于30CrMnSiNi2A基体上熔覆层的硬度;30CrMnSiA热影响区软化现象不明显,而30CrMnSiNi2A热影响区出现了明显的软化现象;30CrMnSiA基体上熔覆层试样的冲击韧性、延伸率均优于基体,抗拉强度为基体的90%以上;30CrMnSiNi2A基体上熔覆层试样的冲击韧性高于基体,抗拉强度约为基体的85%,延伸率约为基体的45%。
4) 30CrMnSiA合金粉末可用于激光熔覆修复30CrMnSiA钢,但修复30CrMnSiNi2A钢则需要进一步调控工艺参数,可采用更小的光斑,以减小热影响区宽度,减少粗晶马氏体的生成以及熔覆层中马氏体的分解。
[1] 杨斯达. 30CrMnSiA钢激光-MAG复合焊接裂纹形成原因与抑制措施[D]. 哈尔滨: 哈尔滨工业大学, 2012: 1- 9.
Yang SD. Formation causes and suppression of cracking in laser-MAG hybrid welding of 30CrMnSiA steel[D]. Harbin: Harbin Institute of Technology, 2012: 1- 9.
[2] 庄明祥, 李小曼, 徐梅, 等. 30CrMnSiNi2A超高强度钢真空电子束焊接工艺应用研究[J]. 航空制造技术, 2017, 60(6): 100-104.
[3] 王华明. 金属材料激光表面改性与高性能金属零件激光快速成形技术研究进展[J]. 航空学报, 2002, 23(5): 473-478.
[4] Da Sun S, Fabijanic D, Barr C, et al. In-situ quench and tempering for microstructure control and enhanced mechanical properties of laser cladded AISI 420 stainless steel powder on 300M steel substrates[J]. Surface and Coatings Technology, 2018, 333: 210-219.
[6] Liu J, Li J, Cheng X, et al. Microstructures and tensile properties of laser cladded AerMet100 steel coating on 300M steel[J]. Journal of Materials Science & Technology, 2018, 34(4): 643-652.
[7] Rahman Rashid R A, Nazari K A, Barr C, et al. Effect of laser reheat post-treatment on the microstructural characteristics of laser-cladded ultra-high strength steel[J]. Surface and Coatings Technology, 2019, 372: 93-102.
[8] Rahman Rashid R A, Barr C J, Palanisamy S, et al. Effect of clad orientation on the mechanical properties of laser-clad repaired ultra-high strength 300M steel[J]. Surface and Coatings Technology, 2019, 380: 125090.
[9] Barr C, Da Sun S, Easton M, et al. Influence of delay strategies and residual heat on in situ tempering in the laser metal deposition of 300M high strength steel[J]. Surface and Coatings Technology, 2020, 383: 125279.
[11] Lourenço J M, Sun S D, Sharp K, et al. Fatigue and fracture behavior of laser clad repair of AerMet ® 100 ultra-high strength steel[J]. International Journal of Fatigue, 2016, 85: 18-30.
[12] Walker K F, Lourenço J M, Sun S, et al. Quantitative fractography and modelling of fatigue crack propagation in high strength AerMet® 100 steel repaired with a laser cladding process[J]. International Journal of Fatigue, 2017, 94: 288-301.
[13] Barr C, Da Sun S, Easton M, et al. Influence of macrosegregation on solidification cracking in laser clad ultra-high strength steels[J]. Surface and Coatings Technology, 2018, 340: 126-136.
[14] Sun S D, Liu Q C, Brandt M, et al. Effect of laser clad repair on the fatigue behaviour of ultra-high strength AISI 4340 steel[J]. Materials Science and Engineering A, 2014, 606: 46-57.
[15] Chew Y. Pang J H L, Bi G J, et al. Effects of laser cladding on fatigue performance of AISI 4340 steel in the as-clad and machine treated conditions[J]. Journal of Materials Processing Technology, 2017, 243: 246-257.
[16] Sun G F, Yao S, Wang Z D, et al. Microstructure and mechanical properties of HSLA-100 steel repaired by laser metal deposition[J]. Surface and Coatings Technology, 2018, 351: 198-211.
[17] 黎文强. 30CrMnSi钢表面激光熔覆强化技术研究[J]. 特种铸造及有色合金, 2019, 39(10): 1058-1061.
Li W Q. Laser cladding intensification on 30CrMnSi surface[J]. Special Casting & Nonferrous Alloys, 2019, 39(10): 1058-1061.
[18] 张志强, 秦仁耀, 孙涛, 等. 30CrMnSiA钢激光熔覆接头的微观组织和性能[J]. 焊接技术, 2018, 47(12): 9-14.
Zhang Z Q, Qin R Y, Sun T, et al. Microstructure and properties of laser cladding joints of 30CrMnSiA steel[J]. Welding Technology, 2018, 47(12): 9-14.
[19] 周可欣, 秦仁耀, 曹强, 等. 30CrMnSiNi2A钢激光熔覆1Cr15Ni4Mo3粉末工艺[J]. 激光与光电子学进展, 2018, 55(7): 071404.
[20] 张志强, 程宗辉, 曹强, 等. 30CrMnSiNi2A超强钢激光熔覆修复试验研究[J]. 装备环境工程, 2016, 13(1): 62-67.
Zhang Z Q, Cheng Z H, Cao Q, et al. Repairing of 30CrMnSiNi2A high strength steel by laser cladding[J]. Equipment Environmental Engineering, 2016, 13(1): 62-67.
[21] 朱红梅, 胡际鹏, 李柏春, 等. 铁基材料表面激光熔覆不锈钢涂层的研究进展[J]. 表面技术, 2020, 49(3): 74-84.
Zhu H M, Hu J P, Li B C, et al. Research progress of laser cladding stainless steel coating on Fe-based substrate[J]. Surface Technology, 2020, 49(3): 74-84.
[22] Lei Z L, Li B W, Ni L C, et al. Mechanism of the crack formation and suppression in laser-MAG hybrid welded 30CrMnSiA joints[J]. Journal of Materials Processing Technology, 2017, 239: 187-194.
[23] 李学军, 黄坚, 潘华, 等. QP1180高强钢薄板激光焊接接头的组织与成形性能[J]. 中国激光, 2019, 46(3): 0302006.
[24] Chipman J. Thermodynamics and phase diagram of the Fe-C system[J]. Metallurgical and Materials Transactions B, 1972, 3(1): 55-64.
[25] 苏铁健, 王富耻, 李树奎, 等. 合金钢的热导率计算[J]. 北京理工大学学报, 2005, 25(1): 91-94.
Su T J, Wang F C, Li S K, et al. Calculation of thermal conductivity for alloy steels[J]. Transactions of Beijing Institute of Technology, 2005, 25(1): 91-94.
[27] Sattar A, Abbas M, Hasham H J, et al. Experimental and analytical investigation of steel bolts failed after isothermal heat treatment[J]. Journal of Failure Analysis and Prevention, 2015, 15(2): 327-333.
[28] Liu BX, Chen CX, Yin FX, et al.Microstructure analysis and weldability investigation of stainless steel clad plate[M] ∥The Minerals, Metals & Materials Series. Cham: Springer International Publishing, 2017: 425- 433.
[29] 朱红梅, 胡文锋, 李勇作, 等. 回火温度对马氏体不锈钢激光熔覆层组织和性能的影响[J]. 中国激光, 2019, 46(12): 1202001.
庞小通, 龚群甫, 王志杰, 李铸国, 姚成武. 30CrMnSiA和30CrMnSiNi2A高强钢激光熔覆修复后的组织特征与力学性能[J]. 中国激光, 2020, 47(11): 1102002. Pang Xiaotong, Gong Qunfu, Wang Zhijie, Li Zhuguo, Yao Chengwu. Microstructures and Mechanical Properties of 30CrMnSiA and 30CrMnSiNi2A High-Strength Steels After Laser-Cladding Repair[J]. Chinese Journal of Lasers, 2020, 47(11): 1102002.