Laboratory demonstration of static-mode down-looking synthetic aperture imaging ladar
Download: 1099次
A synthetic aperture imaging ladar (SAIL) can provide fine resolution, two-dimensional (2D) active imaging at a long range with small-diameter optics. It recently became an interesting field of research, and two kinds of SAILs, the side-looking SAIL and the down-looking SAIL, have been reported[1
The down-looking SAIL is based on the key ideas of wavefront transformation and regulation by optical techniques, with a transmitter of two coaxial and orthogonal-polarization beams, and a receiver of self-heterodyne detection. In the orthogonal direction of travel, a linear phase modulation proportional to the lateral distance of the target point is generated by scanning two cylindrical lenses with the same curvatures of the wavefront in reverse. A quadratic phase history centered in the longitudinal position of the target point in the travel direction is produced by using another two cylindrical lenses with different curvatures of the wavefront. The image is focused by the Fourier transform and matched filtering in the two respective directions. The down-looking SAIL has as its inherent feature a controllable and changeable size of the optical footprint, which, together with the associated imaging resolution, can be, manipulated on a large scale. Meanwhile, it effectively reduces the influence of atmospheric turbulence, and simplifies the system structure. Clearly, the down-looking SAIL would be appropriate for practical uses.
In this Letter, we propose the concept of a static-mode down-looking SAIL based on the previous down-looking SAIL[11,12]. We keep the target and carrying platform still. Two cylindrical lenses with the same curvatures of the wavefront are scanned to produce a linear phase modulation. Another cylindrical lens is moved slowly along the direction of travel to produce a quadratic phase history, instead of moving the carrying platform. In the laboratory, a point image and a 2D image are reconstructed under simulated far-field conditions with an optical collimator that has a focal length of 10 m. In particular, the static mode is unique in the down-looking SAIL; it is difficult to implement in the traditional microwave synthetic aperture radar (SAR) and the side-looking SAIL.
The schematic diagram of the static-mode SAIL system is illustrated in Fig.
In our experiment, cylinder lens L2 and cylinder lens L3 scan periodically with a fast velocity. Meanwhile, cylinder lens L4 moves along the
Via an amplified transmission, the inner field is imaged onto the focal plane of the optical collimator with an amplification factor of
During the imaging process, the 2D data was first compressed by the Fourier transform before the target frequency was extracted with a band-pass filter. Then, the 2D data was compressed by a match filter with a conjugate quadratic phase of the phase history in the travel direction.
A static-mode down-looking SAIL demonstration with an optical collimator that has a focal length of 10 m was designed, and the target and carrying platform are relatively static. A Gaussian beam with a wavelength of 532 nm and a power of 100 mW was used in the experiments. A positive lens with a focal length of 100 mm (L1) was used to adjust the orthogonal-polarization beams’ size. The focal lengths of the three cylindrical lenses are all 60 mm, and the size of the lenses are all
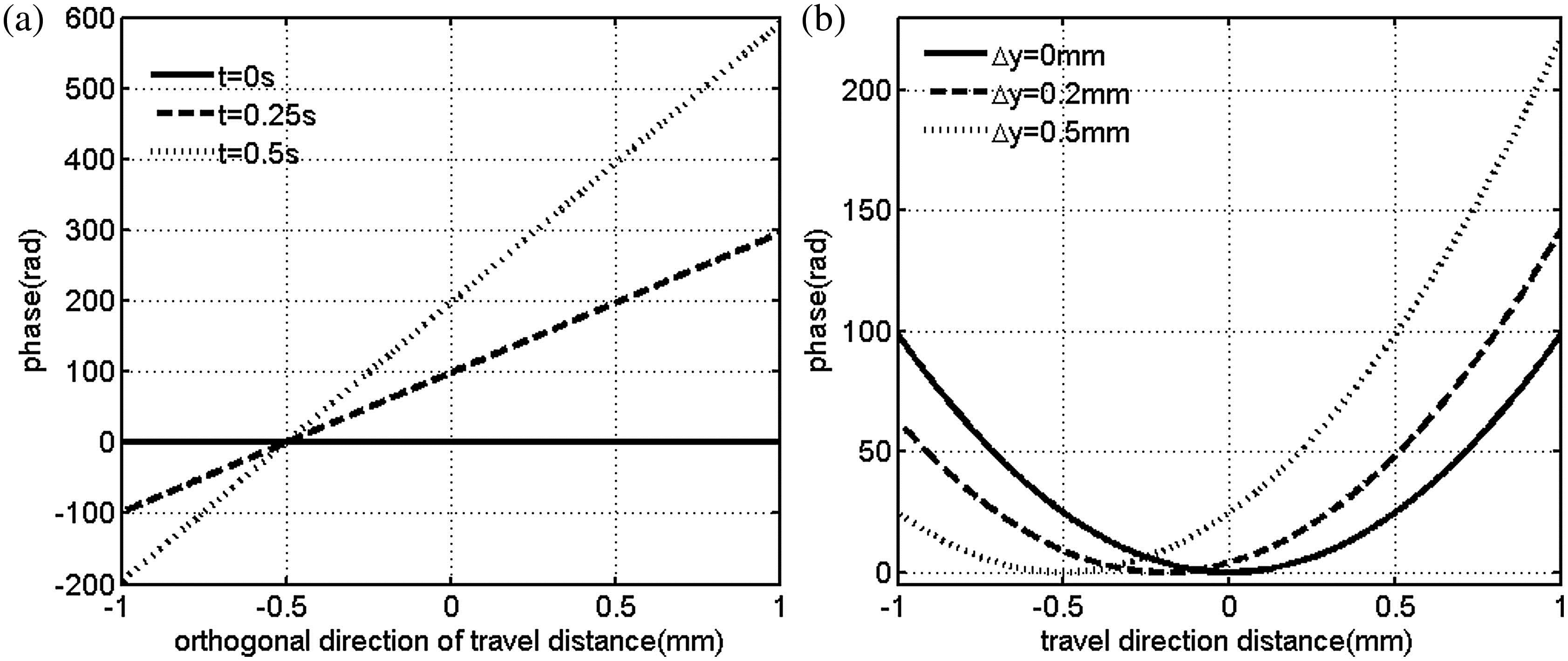
Fig. 2. The phase difference in the inner optical fields. (a) Orthogonal direction of travel. (b) Travel direction.
The diameter of the Gaussian illumination spot on the target plane was about 40 mm. The target was placed on the focal plane of the optical collimator, and the focal length was 10 m. The targets were made of 3M reflective sheets. The scattered light from the target was collected and collimated by a 155.5 mm diameter-receiving telescope. Two mini-lenses were placed after the 180° hybrid to focus the light towards the detectors of the balanced receivers.
The theoretical resolution in the orthogonal direction of travel is inversely proportional to the scanning range of the cylindrical lenses[8]. A scanning range of 1.2 mm was used in experiment, so the corresponding theoretical resolution is 0.53 mm. Similarly, the corresponding theoretical resolution in the travel direction is 0.73 mm under the moving range of 1.5 mm. A
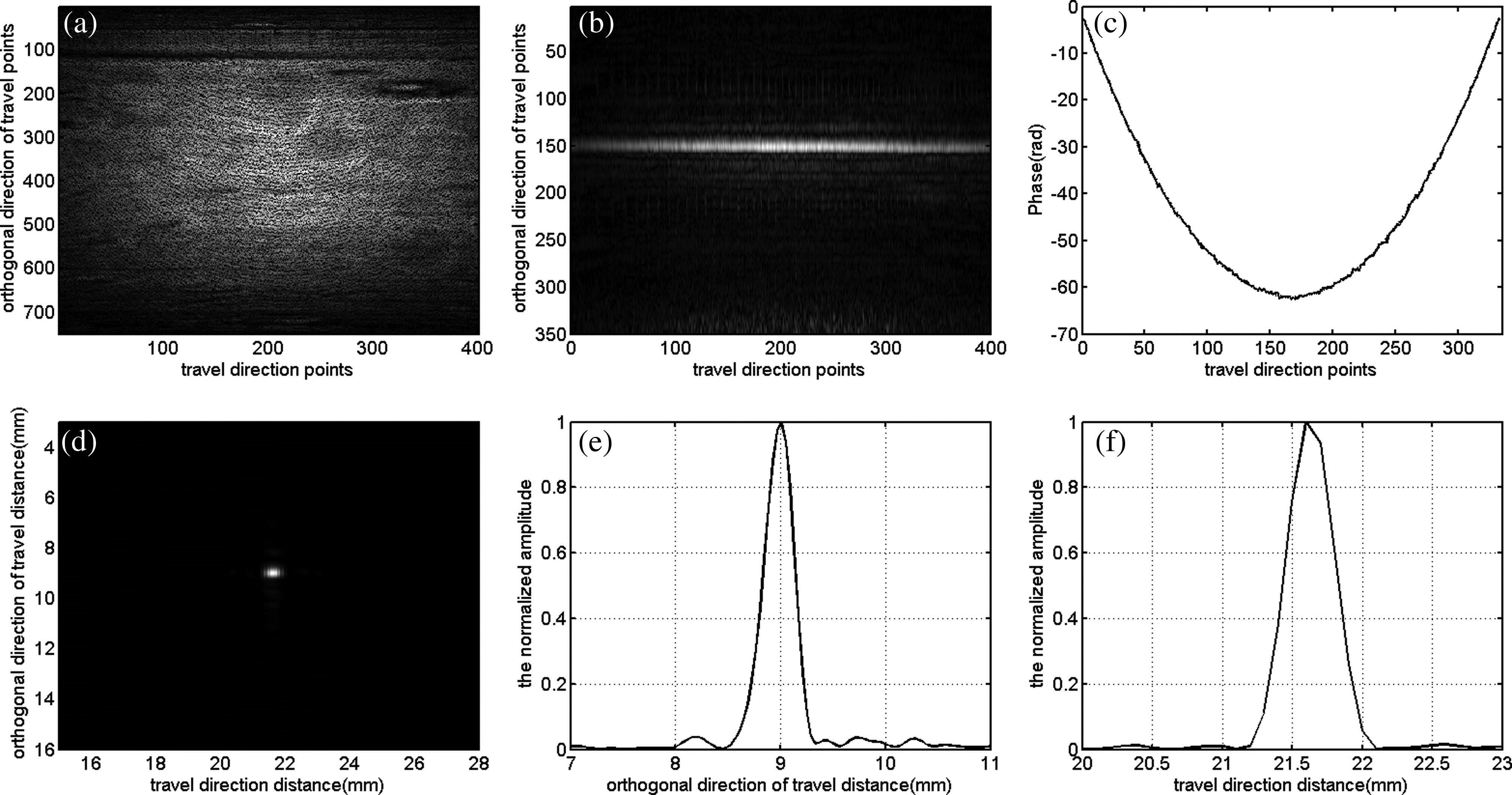
Fig. 3. Reconstructed image process of a point. (a) Return signal data. (b) The image focused by the Fourier transform. (c) Quadratic phase in the travel direction. (d) Reconstructed point image by matching filtering. (e) Point spread functions in the orthogonal direction of travel. (f) Point spread functions in the travel direction.
The imaging experiments of 2D targets were operated under the same conditions, as shown in Fig.
It should be emphasized that the static-mode down-looking SAIL uses an optical collimator to simulate the far-field situation due to the limitations of the laboratory site. The static-mode down-looking SAIL has the ability to automatically compensate for the influence of atmospheric turbulence and unmodeled line-of-sight motion, similar to the previous down-looking SAIL. As a consequence, the static-mode down-looking SAIL could be used outdoors.
In conclusion, we present a static-mode down-looking SAIL and its imaging experiments using an optical collimator with a 10 m focal length to simulate the far-field condition. The resolution is
[1]
[2]
[3]
[6]
[7]
[8]
[9]
[10]
[11]
[12]
[13]
Zhiyong Lu, Ning Zhang, Jianfeng Sun, Yu Zhou, Zhu Luan, Guangyu Cai, Liren Liu. Laboratory demonstration of static-mode down-looking synthetic aperture imaging ladar[J]. Chinese Optics Letters, 2015, 13(4): 042801.