Photoacoustic characteristics of lipid-rich plaques under ultra-low temperature and formaldehyde treatment
Download: 714次
Acute cardiovascular events, caused by atherosclerotic plaque rupture and subsequent thrombosis, are still the leading cause of mortality and morbidity worldwide. These rupture-prone plaques are deemed as vulnerable plaques, characterized by a large lipid core and thin fibrous cap[1,2]. Clinically available imaging techniques with high spatial resolution
To date, most reports involve samples stored in formaldehyde or under ultra-low temperature. As we know, formaldehyde fixation may lead to lipid loss[10,11], and the freeze–thaw cycle can degenerate proteins and biological molecules. These could alter the photoacoustic (PA) characteristics of the tissues, which might, in turn, cause bias on the results explanation and pre-clinical translational research on PAI. In this Letter, we aim to compare the PAI results before and after these two treatments with histology and the golden standard to validate their effects on artery PA features.
The scheme of the PAI system is shown in Fig.
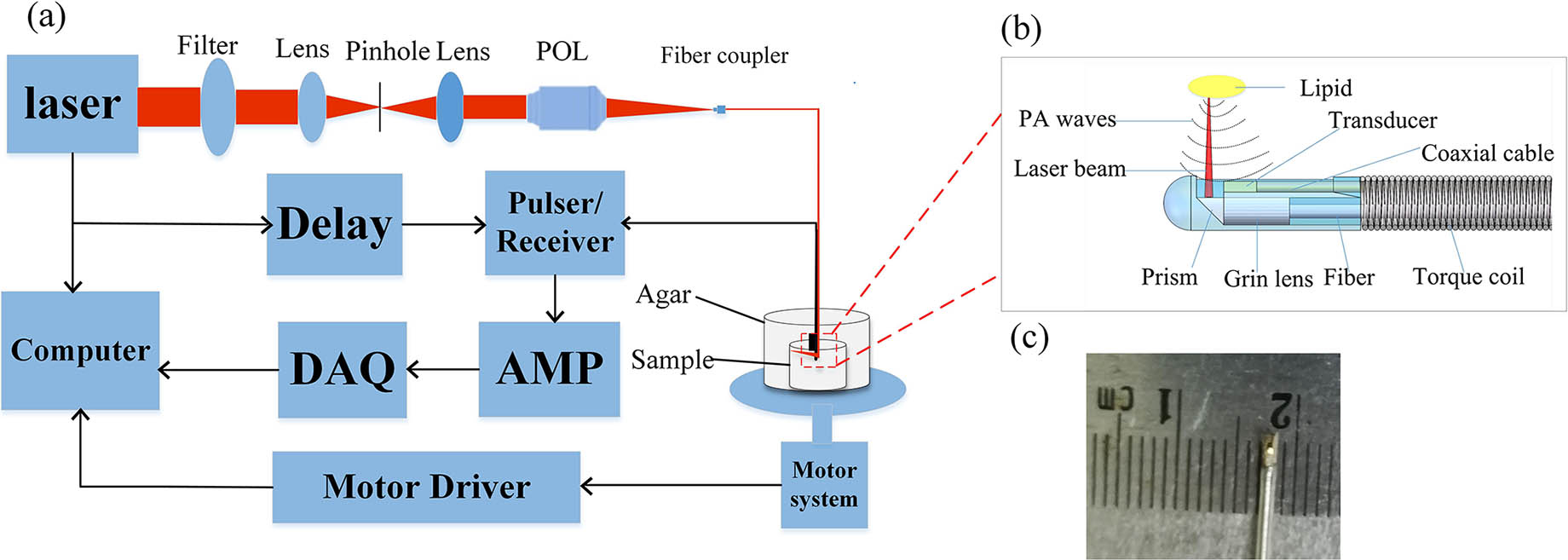
Fig. 1. Schematic of the system and photograph of the devices. (a) Schematic of the system: POL, plan objective lens; AMP, amplifier; DAQ, data acquisition system. (b) Schematic of the laser emission and PA signals reception. (c) Photograph of the integrated transducer.
The atherosclerotic rabbit model was established according to our previous reports[12,13]. Briefly, male New Zealand white rabbits with 2 kg in weight were fed with atherogenic diet (1% cholesterol) and underwent balloon-induced abdomen aorta endothelial denudation by a 4 Fr balloon catheter through the right femoral artery. The high cholesterol diet was conducted for 20 weeks successively. After sacrificed by euthanasia, the abdomen aorta was excised and divided into six equal 1-cm-long segments and numbered from 1–6. All animal care and experimental protocols complied with the Animal Management Rule of the Ministry of Public Health, People’s Republic of China and were approved by the Animal Care Committee of Shandong University.
As is well-known, the blood shows strong optical absorption in the range 400–600 nm, and water-rich tissue greatly absorbs the energy of light from 900 nm to the far-infrared range. Light in a narrow range of 600–900 nm can effectively avoid the strong absorption, which is known as an optical window for biomedical imaging. A pulsed laser at 760 and 780 nm wavelengths was used for vascular sample imaging[14
The vessel-mimic agar phantom was fixed in an electric displacement platform for supporting the artery segments placed within it. The high-frequency ultrasound transducer was used to collect PA signals. The performance of the transducer is shown in Fig.
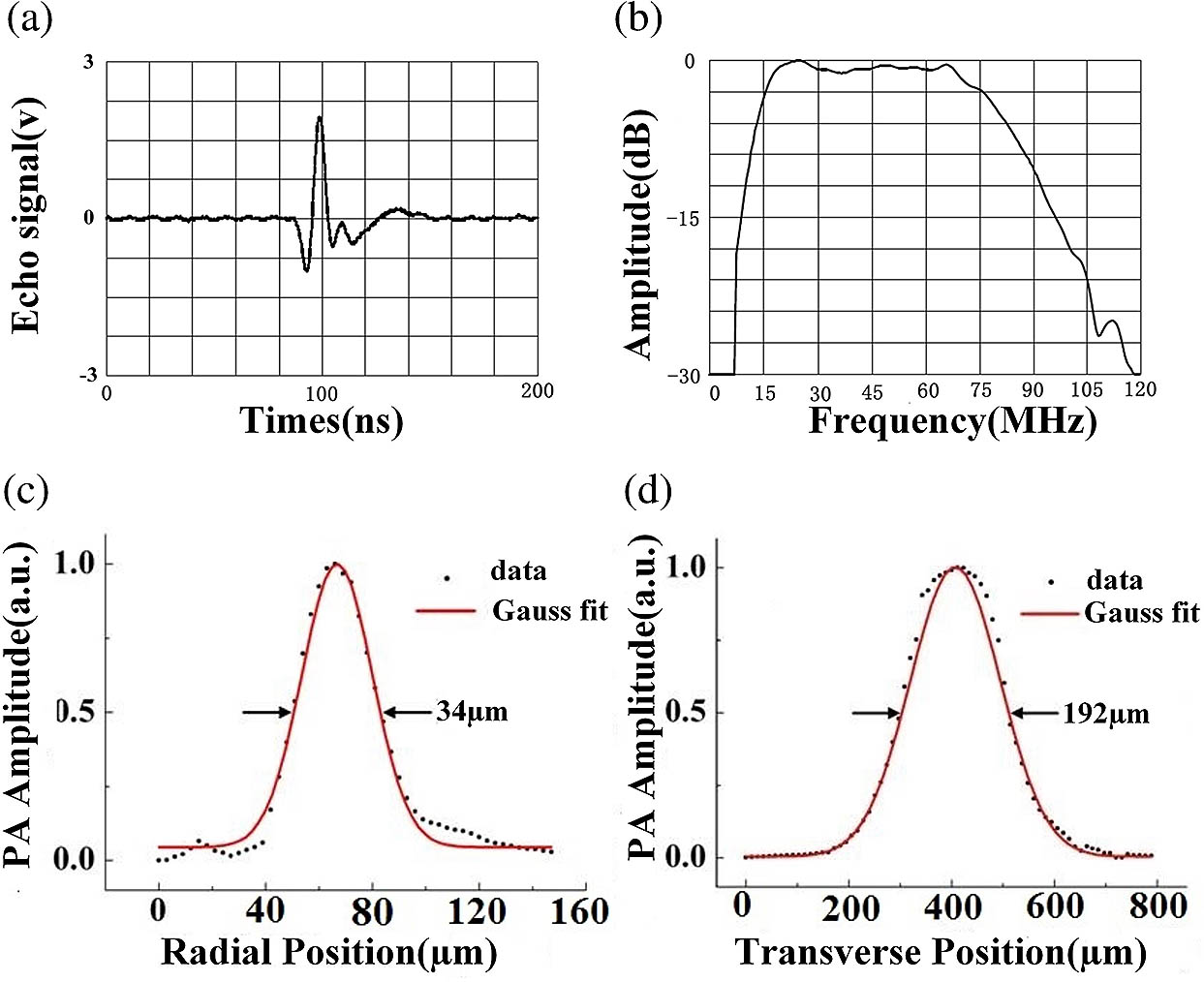
Fig. 2. Performance of the transducer and system. (a) The echo signal detected by the transducer. (b) Bandwidth of the transducer elements with a bandwidth of 95%. (c) The axial resolution of the system. (d) The lateral resolution of the system.
To prove the spatial resolution of the system, a 20 μm diameter carbon fiber was immersed in the inner surface of a gelatin phantom, the PAI of the carbon fiber has been done, and, according to the PA image, the experimental data of the imaged carbon fiber was plotted along its axial and lateral directions, respectively. The dotted lines were then fitted with Gaussian functions to estimate the radial and transverse resolutions of PAI. Based on the full width at half-maximum (FWHM) of the fitted functions, the axial resolution is estimated to be 34 μm, while the lateral resolution is 192 μm, as shown in Figs.
Artery segments were frozen and histological slices were prepared with the thickness of 5 μm for oil-red O staining. Slides were scanned by microscope (Olympus BX53; Olympus, Japan). The positive staining areas, which indicate the lipid components, were measured and quantified by the index lipid ratio (LR), expressed as the percentage of the stained area divided by the plaque area with the Image-Pro Plus (IPP) software (Image-Pro Plus 6.0, Media Cybernetics)[22,23].
PA images were also analyzed by the IPP software to calculate the LR index. Pixels of the lipid signal (
The lipid component can be clearly identified on PA images, which correlated very well with histology. Numbers 1–3 artery segments were treated by an ultra-low temperature. Although the lipid contents quantified by the PAI tended to decrease after such treatment, the LR index did not differ from pre- to post-treatment significantly (
Table 1. Difference and Correlation of Lipid Ratio Derived by PAI Pre- and Post-Treatments
|
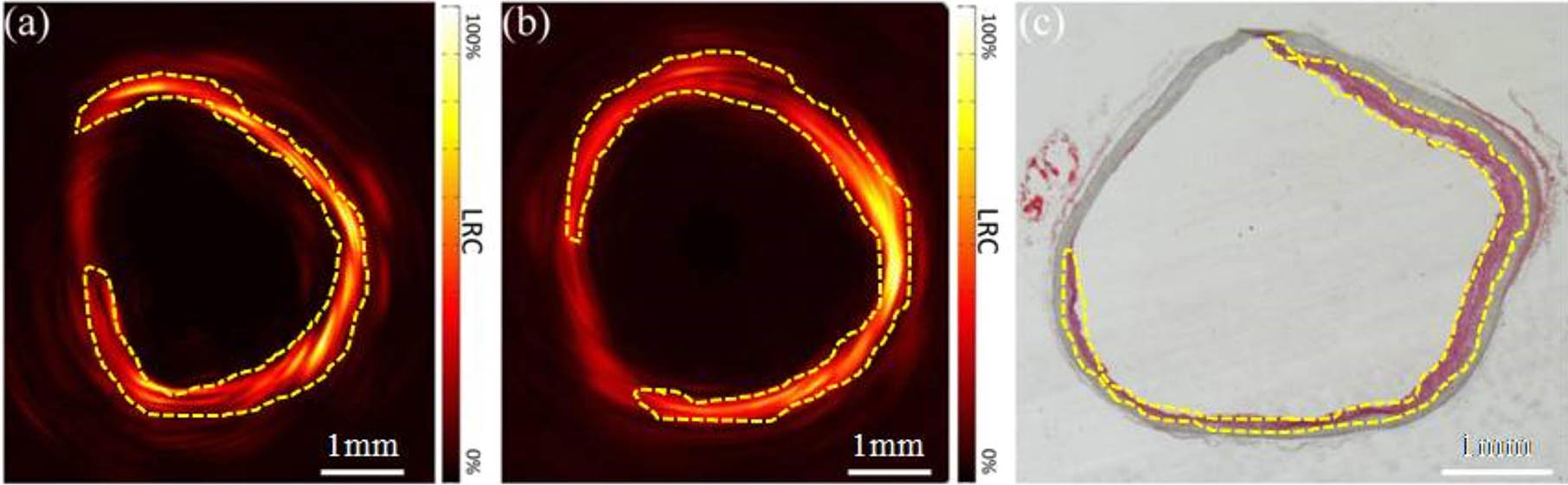
Fig. 3. Comparison of PA images experiencing pre- and post-frozen treatment with histological images. (a) The PA cross-section image of No. 2 vessel before frozen treatment. (b) The PA cross-section image of No. 2 vessel as shown in (a) after frozen treatment. (c) The matched histological cross-section image of No. 2 vessel in (a) and (b). LRC, lipid relative concentration.
Numbers 4–6 artery segments were treated with formaldehyde. As illustrated in Fig.

Fig. 4. Comparison of PA images experiencing pre- and post-formaldehyde treatment with histological images. (a) The PA cross-section image of No. 5 vessel before formaldehyde treatment. (b) The PA cross-section image of No. 5 vessel as shown in (a) after formaldehyde treatment. (c) The matched histological cross-section image of No. 5 vessel in (a) and (b).

Fig. 5. Statistical analysis results. (a) Correlation between LR by histology and PAI post-frozen treatment, . (b) Correlation between LR by histology and PAI post-formaldehyde treatment, .
Although lipid contents quantified by the PAI tended to decrease after such treatment, the LR index did not differ from pre- to post-treatment significantly (
Table 2. Correlation of Lipid Ratio Derived by PAI and Histology Pre- and Post-Treatments
|
Moreover, differences and correlations of LR derived by PAI and histology after different treatments were further explored in Table
Table 3. Difference and Correlation of Lipid Ratio Derived by PAI and Histology Post-Frozen and Formaldehyde Treatment
|
Although the LR indices from pre- and post-treatment PAI agreed with each other, the correlation coefficient after the formaldehyde treatment is reduced to 0.658 (Table
In this study, we found that ultra-low temperature or formaldehyde treatment has few effects on lipid characterization by PAI. As we know, formaldehyde fixation may lead to lipid loss[24]. However, Donato
We validate that neither ultra-low temperature nor formaldehyde treatment has an effect on the PA characteristics of arteries acquired from the atherosclerotic rabbit model. PAI holds high accuracy of lipid identification compared to histology.
[1]
[2]
[3]
[4]
[5]
[6]
[7]
[8]
[9]
[10]
[11]
[12]
[13]
[14]
[15]
[16]
[17]
[18]
[19]
[20]
[21]
[22]
[23]
[24]
[25]
[26]
[27]
[28]
Mingjun Xu, Peng Lei, Jianqin Feng, Fangfang Liu, Sihua Yang, Pengfei Zhang. Photoacoustic characteristics of lipid-rich plaques under ultra-low temperature and formaldehyde treatment[J]. Chinese Optics Letters, 2018, 16(3): 031702.