Pd插层NbSe2化合物的制备、晶体结构和电学性质研究
Layered transition metal dichalcogenides (TMDs) with the general chemical formula MX2 (M represents the transition metal and X is the chalcogen) have been widely studied due to their unique physicochemical properties and diverse applications[1,2,3,4,5]. The metallic Group ⅤB TMDs (where M = V, Nb and Ta) are prized for their fascinating electronic properties, such as charge density wave (CDW), superconductivity and Mott transition[6,7]. Among them, 2H-NbSe2 is featured with a high superconducting critical temperature (TC) of ~7.3 K and a quasi-two-dimensional incommensurate charge density wave (ICDW) with a TCDW of ~33 K[8]. Because of the weak van der Waals (vdW) forces connected interlayers in the crystal structure, 2H-NbSe2 can be intercalated by various guests, including atoms, ions, and molecules[9].
Typically, the incorporation of guest metal atoms into the vdW gaps of TMDs could give rise to the crystallographic transformation and change of electronic structure in the intercalated compounds[10]. Magnetic elements (Fe, Co) inserted into the vdW gaps of NbSe2 resulted in the formation of superlattice[11,12]. Alkali metal intercalation was found to remove the CDW instability in NbSe2[13]. Recently, noble metal, such as palladium (Pd), was applied to regulate the electronic structure efficiently for the host TMDs. Our group[14] found that Pd modified the band structure of 2H-TaS2 through Pd-S bonding to strengthen the interaction of adjacent Ta-S layers, which led to the enhanced conductivity in Pd0.10TaS2. Pd intercalation was reported to increase the effective electron- phonon coupling in 2H-TaSe2 and enhance the TC in PdxTaSe2[15]. Considering that the crystal structure of 2H-NbSe2 is identical to that of 2H-TaX2 (X=S, Se), Pd intercalation should be applicable to 2H-NbSe2 and tune the physical properties.
In this work, a series of new compounds PdxNbSe2 (x=0~0.17) were synthesized and the crystal structure of Pd0.17NbSe2 was determined by single X-ray diffraction method in order to investigate the modification of crystal lattice and electrical conductivity in the Pd intercalated NbSe2.
1 Experimental
1.1 Preparation of PdxNbSe2
PdxNbSe2 crystals were prepared by solid-state reaction. Pd (99.99%), Nb (99.5%) and Se (99.99%) powders were mixed according to stoichiometric ratio, and ground. Then the mixtures were compacted into a pellet and heated in the evacuated (< 0.133 Pa) silica tube at 1173 K for 48 h. Subsequently, the as-obtained samples were reground, re-pelletized and held at 1173 K for 72 h. Then the samples were cooled down by quenching in water. High quality single crystal of Pd0.17NbSe2 was obtained by keeping Pd0.17NbSe2 powder with CsI (99.9%) at 1173 K for 1 d and slowly cooling down to 823 K for 3 d.
1.2 Characterization
Single crystal data collections of Pd0.17NbSe2 was conducted on a Bruker D8 QUEST diffractometer equipped with Mo Kα radiation at room temperature. The crystal structure determination and refinement were performed with the APEX3 program. The crystal structure of Pd0.17NbSe2 was drawn by using the VESTA program[16]. The morphology and the composition of the Pd0.17NbSe2 were investigated by a scanning electron microscope (SEM, JSM6510) coupled with energy dispersive X-ray spectroscopy (EDXS, Oxford Instruments). The micro-structure of Pd0.17NbSe2 was uncovered by a high-resolution transmission electron microscope (HRTEM, JEM-2100F) and the selected area electron diffraction (SAED). The valence analysis of the Pd0.17NbSe2 was obtained from X-ray photoelectron spectroscope (XPS) carried out on the RBD upgraded PHI-5000C ESCA system (PerkinElmer) with Mg Kα radiation (hv=51253.6 eV). The binding energy in XPS analysis was corrected by referencing C 1s peak at 284.6 eV. Powder X-ray diffraction (PXRD) data of these PdxNbSe2 samples were collected by using a Bruker D8QUEST diffractometer equipped with Cu Kα radiation (λ=0.15405 nm). Thermogravimetric analysis (TG) and differential thermal analysis (DTA) were carried out on a NETZSCH STA449C thermal analyzer for investigating the thermal stability of Pd0.17NbSe2 and NbSe2 in air. Resistivity of the as-obtained PdxNbSe2 at different temperatures was executed on a Physical Properties Measurement System (PPMS, Quantum Design). A four-probe method was adopted for measurements of the resistance. More specifically, the powders were pressed into a disk. Silver paste and copper wire acted as the contact electrode and conduct wire, respectively. Normalized resistivity (ρ/ρ300 K) versus temperature curves were obtained via dividing the measured resistivity (ρ) by the resistivity value (ρ300 K) at room temperature.
2 Results and discussion
The crystal structure of Pd0.17NbSe2 identified by single crystal X-ray diffraction method is shown in Fig. 1(a-b), where the gray, blue, orange spheres represent Pd, Nb, and Se atoms, respectively. The crystal data and structure refinement of Pd0.17NbSe2 are given in Table 1. The fractional atomic coordinates and equivalent isotropic displacement parameters are summarized in Table S1. The atomic displacement parameters and the geometric pa rameters are shown in Table S2-S3. The space group of Pd0.17NbSe2 is determined to be P63/mmc with lattice parameters of a=0.34611(2) nm, c=1.27004(11) nm. Pd0.17NbSe2 contains one independent Nb site (2b), one independent Se site (4f) and one independent Pd site (2a). Pd0.17NbSe2 consists of Nb-Se layer and Pd-Se layer, which are stacked alternately along c axis. Each Nb atom is coordinated by 6 Se atoms which formed a [NbSe6] triangular prism (Fig. 1(c)). The length of Nb-Se bond in [NbSe6] triangular prism is 0.26006(4) nm which is comparable to 0.25941(5) nm in NbSe2[17]. These [NbSe6] triangular prisms are connected by edge-sharing to form the Nb-Se layer.
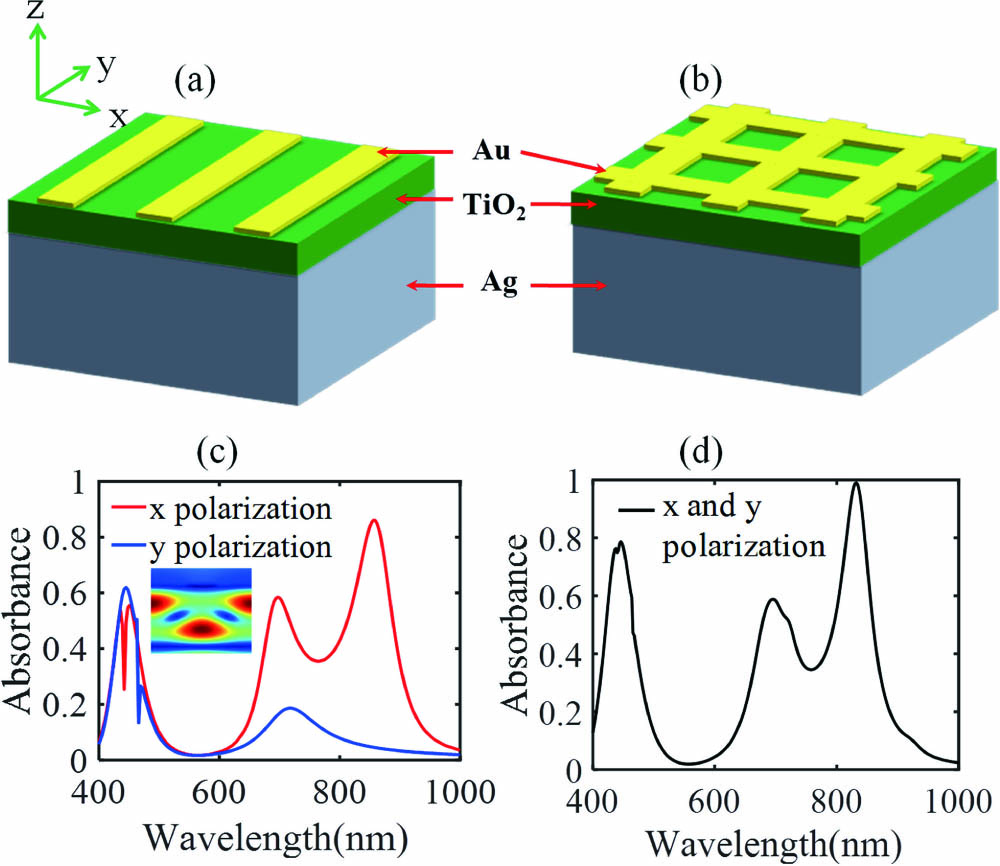
图 1.
Fig. 1. Crystal structure of Pd0.17NbSe2 along (a) the bc -plane and (b) the ab -plane, (c) [NbSe6] triangular prism in Pd0.17NbSe2, (d) [PdSe6] octahedron in Pd0.17NbSe2
表 1.
Fractional atomic coordinates and isotropic or equivalent isotropic displacement parameters of Pd0.17NbSe2
Table 1.
Fractional atomic coordinates and isotropic or equivalent isotropic displacement parameters of Pd0.17NbSe2
|
Each Pd atom is coordinated by 6 Se atoms to form [PdSe6] octahedron (Fig. 1(d)). The average length of Pd-Se bond in [PdSe6] octahedron is 0.25051(4) nm which is comparable to 0.248602(0) nm of PdSe2[18]. These [PdSe6] octahedra partially fill in the vdW gaps of NbSe2, where the occupation of Pd sites is 17%.
The Pd0.17NbSe2 plate with the size about 5 μm was observed by SEM (Fig. 2(a)). The Pd atoms are in a homogenous dispersion in Pd0.17NbSe2, which is confirmed by the elemental mapping analysis of Pd0.17NbSe2. HRTEM image of Pd0.17NbSe2 (Fig. 2(b)) reveals that the lattice fringes with a spacing of 0.301 nm are assigned to (101) plane and (11¯1) plane between which the angle is 60°. This result is also verified by the corresponding SAED.
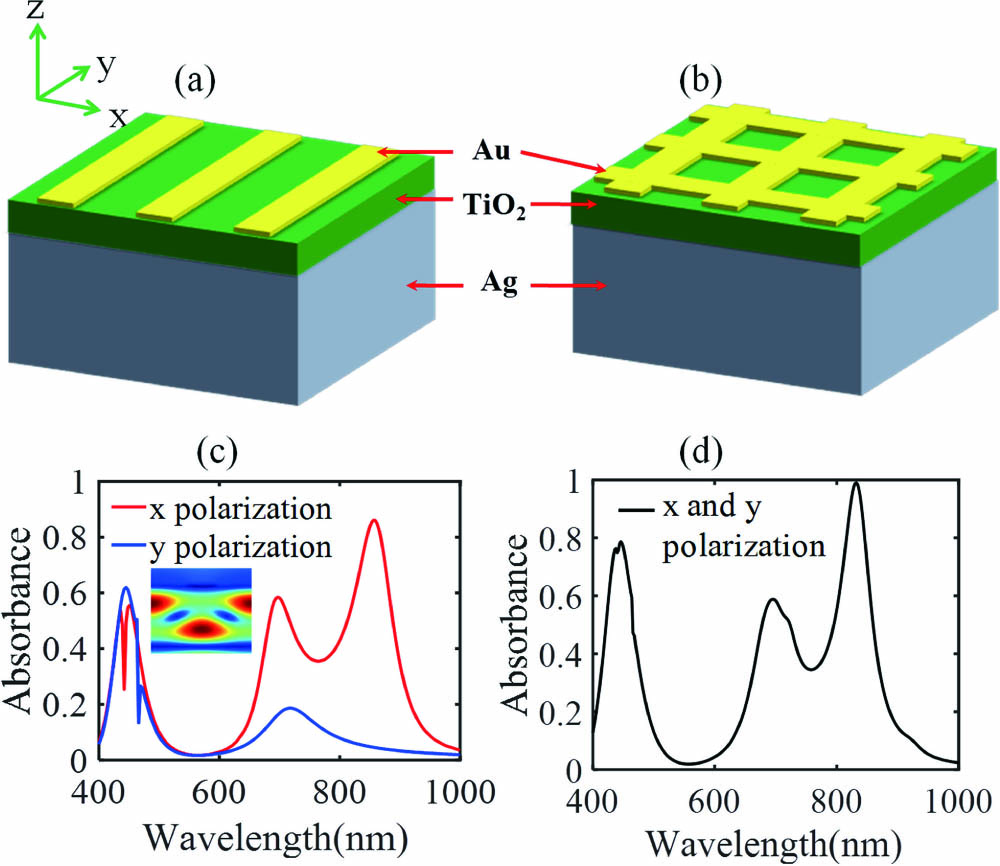
图 2.
Fig. 2. (a) SEM images of Pd0.17NbSe2 and the corresponding elemental mapping analysis, and (b) HRTEM image of Pd0.17NbSe2 along [101¯] zone axis with inset showing the corresponding SAED pattern
XPS data was obtained to confirm the valence state variation of the elements in Pd0.17NbSe2. As displayed in Fig. 3(a), the Pd 3d region is the only difference between Pd0.17NbSe2 and NbSe2. The Pd 3d region of Pd0.17NbSe2 shows two peaks, which locate at the binding energy of 341.95 eV (3d3/2) and 336.70 eV (3d5/2) (Fig. 3(b)). The valance state of Pd in Pd0.17NbSe2 is identified as +2 according to these two peaks[14]. There are two peaks locating at 55.27 (Se 3d3/2) and 54.50 eV (Se 3d5/2) in the Se 3d region of Pd0.17NbSe2, similar to those in the Se 3d region of NbSe2 (Se 3d3/2 at 55.25 eV and Se 3d5/2 at 54.49 eV) (Fig. 3(c)). Therefore, the valance state of Se in Pd0.17NbSe2 is considered as -2. The Nb 3d region shows a mixture of oxidation states because of the slightly oxidation of the samples (Fig. 3(d))[19]. The peaks locating at 206.93 and 204.20 eV are attributed to the Nb-Se bonding in Pd0.17NbSe2. In comparison with these two peaks in pristine NbSe2 (207.01 and 204.25 eV), there is a slight redshift in Pd0.17NbSe2, implying the partial reduction of Nb as a result of Pd intercalation[14].
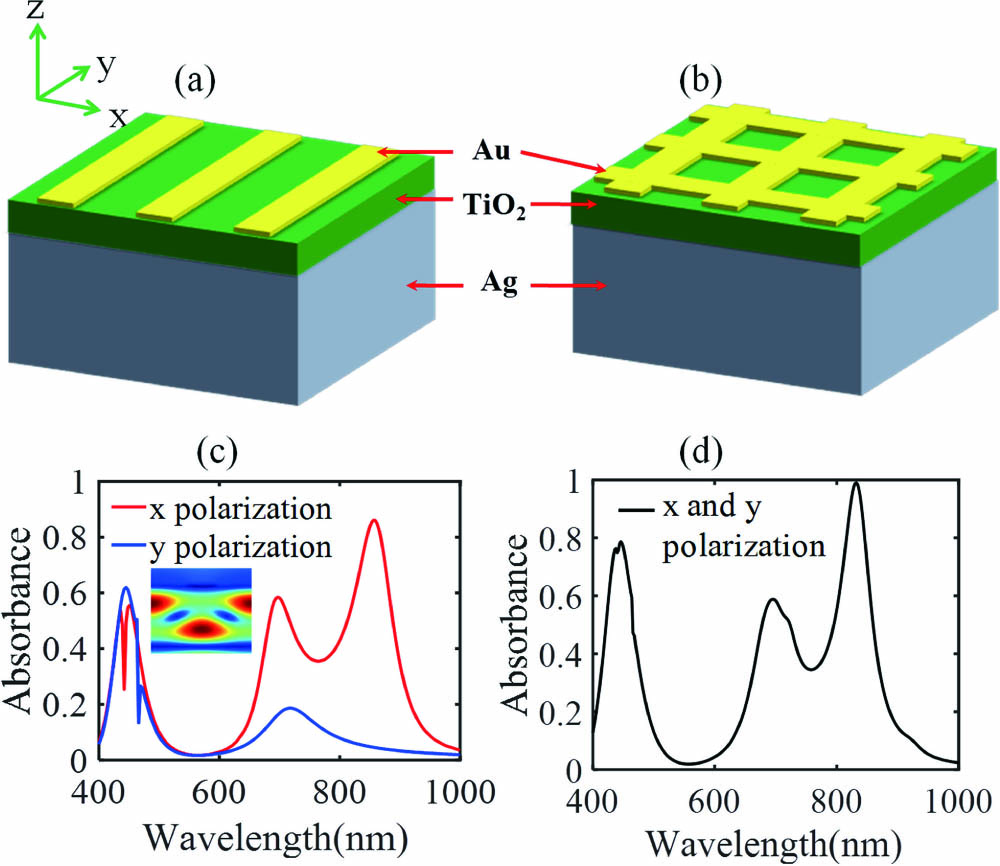
图 3.
Fig. 3. XPS results of Pd0.17NbSe2 and NbSe2(a) Survey spectra, (b) Pd 3d spectrum of Pd0.17NbSe2, (c) Se 3d spectrum, and (d) Nb 3d spectrum
The intercalated amounts of Pd in NbSe2 could be variable, resulting in the formation of a series of PdxNbSe2. The powder XRD patterns of PdxNbSe2 are displayed in Fig. 4(a), with the pristine NbSe2 as reference. The peaks of Pd0.17NbSe2 are well matched to the simulated one obtained from single crystal data, which suggests a high degree of phase purity. The NbSe2 still maintains its space group (P63/mmc) after Pd intercalation. The (004) peak gradually shifts to a lower angle compared with 2H-NbSe2. Furthermore, the lattice parameter a undergoes a negligible change. In a sharp contrast, lattice parameter c increases remarkably because of Pd intercalation enlarging the interlayer space of NbSe2 (Fig. 4(b)).
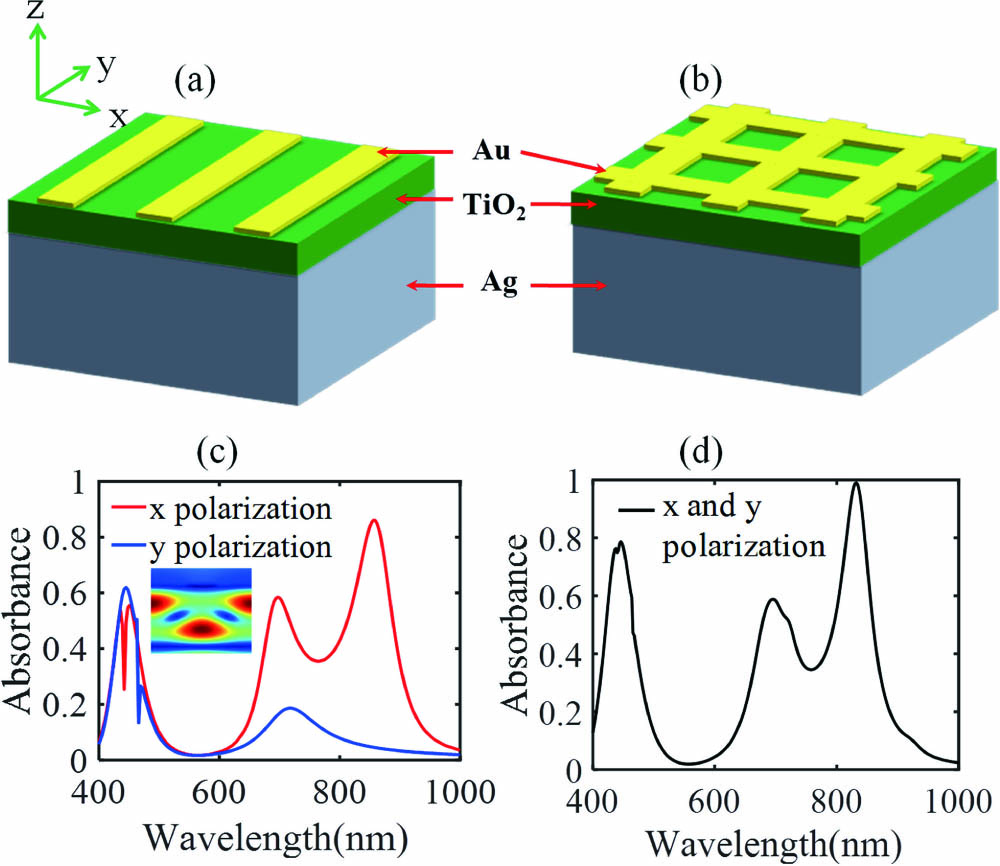
图 4.
Fig. 4. (a) Powder XRD patterns of Pdx NbSe2 (x =0, 0.05, 0.10, 0.15, 0.17), (b) composition dependence of the lattice parameters a and c for Pdx NbSe2 (0≤x ≤0.17)
The influence of Pd intercalation on the thermostability of the samples was investigated. As clearly seen in Fig. 5(a), the weight of NbSe2 begins to increase slightly at 559 K due to the formation of Nb2Se4O13[20]. Subsequently, TG curve of NbSe2 suffers a dramatic decrease because of the complete oxidation of NbSe2 to Nb2O5. However, the process of mass increase could not be found in Pd0.17NbSe2, suggesting that the intercalated Pd enhances the thermostability of NbSe2 with a higher oxidizing temperature. According to DTA curves (Fig. 5(b)), the oxidizing temperature of Pd0.17NbSe2 is 608 K, higher than NbSe2 (544 K). The enhanced thermostability in air could stem from the intercalated Pd which stabilizes the crystal structure of NbSe2 by connecting the adjacent Nb-Se layers[11,21-22].
The electrical conductivity of PdxNbSe2 was measured by PPMS. The resistivity of PdxNbSe2 increases with the rising temperature (Fig. S1) exhibiting metallic behavior. Moreover, the residual resistivity ratio (RRR) [(resistivity at 300 K)/(resistivity just above TC)] for the Pd0.17NbSe2 is ~1.09, extremely lower than NbSe2 (~7.67) (Fig. 6(a)).
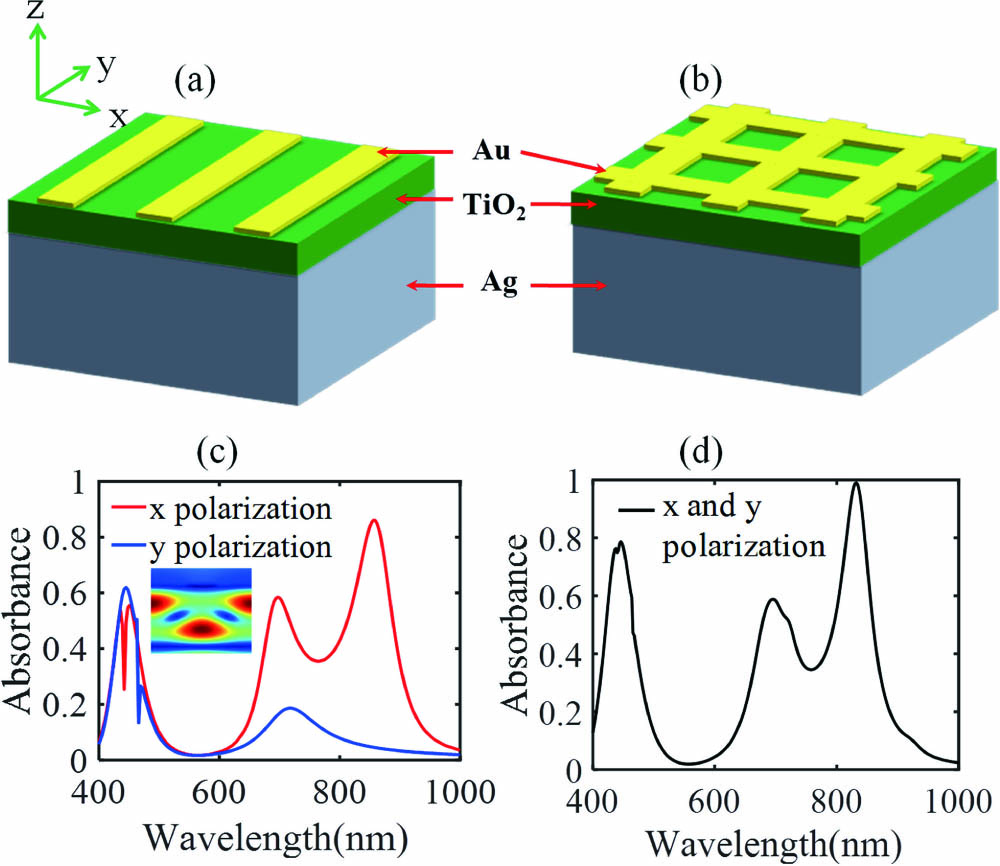
图 6.
Fig. 6. (a) Temperature dependence of the RRR (ρ /ρ 300 K) for Pdx NbSe2 (0≤x ≤0.17) with inset showing enlarged temperature regionof the superconducting transition, (b) composition dependence of T C
The poor RRR in Pd0.17NbSe2 indicates that the intercalated Pd may be an electronically disruptive dopant in NbSe2, which is similar to the copper (Cu) in CuxNbSe2 and the gallium (Ga) in GaxNbSe2[8,23]. All of the PdxNbSe2 samples exhibit a sharp decrease at low temperature region from 8 K to 2K, indicating that the superconductivity occurs in these samples. Fig. 6(b) shows that the TC decreases with a higher intercalated amount of Pd (7.4 K for NbSe2 and 2.7 K for Pd0.17NbSe2). Eventually, the zero resistivity cannot be observed at 2 K in Pd0.17NbSe2. Therefore, it declares that the intercalated Pd has a negative effect on the superconductivity in NbSe2. Similar phenomena are also found in CuxNbSe2, GaxNbSe2, FexNbSe2 and AlxNbSe2[8,23-24]. The reason for this might be that Pd intercalation disrupts the coherence of the CDW, and suppresses the pairing channel which contributes to the higher TC in NbSe2[8].
3 Conclusions
In summary, we introduced noble metal Pd into the vdW gaps of NbSe2, and synthesized a series of new intercalated compounds PdxNbSe2. The Pd0.17NbSe2 crystalizes in hexagonal structure with cell parameter a= 0.34611(2) nm, c=1.27004(11) nm. The intercalated Pd stabilizes the crystal structure of NbSe2 by connecting the adjacent Nb-Se layers with [PdSe6] octahedra leading to the enhanced thermostability in air. PdxNbSe2 remains the metallic character, which is verified by the resistivity measurements. In addition, the incorporation of Pd decreases the TC of NbSe2, implying that Pd is negative for the superconductivity in NbSe2.
7 Supporting information:
表 2.
Atomic displacement parameters of Pd0.17NbSe2
Table 2.
Atomic displacement parameters of Pd0.17NbSe2
|
表 3.
Geometric parameters for Pd0.17NbSe2
Table 3.
Geometric parameters for Pd0.17NbSe2
|
Geometric parameters for Pd0.17NbSe2
Bond | Distance/nm | Bond | Distance/nm |
---|---|---|---|
Nb1—Se2i | 0.26006(4) | Se2—Pd3viii | 0.25051(4) |
Nb1—Se2ii | 0.26006(4) | Se2—Nb1viii | 0.26006(4) |
Nb1—Se2iii | 0.26006(4) | Se2—Nb1vii | 0.26006(4) |
Nb1—Se2iv | 0.26006(4) | Pd3—Se2ix | 0.25051(4) |
Nb1—Se2 | 0.26006(4) | Pd3—Se2iv | 0.25051(4) |
Nb1—Se2v | 0.26006(4) | Pd3—Se2x | 0.25051(4) |
Nb1—Pd3vi | 0.31751(3) | Pd3—Se2i | 0.25051(4) |
Nb1—Pd3 | 0.31751(3) | Pd3—Se2xi | 0.25051(4) |
Se2—Pd3vii | 0.25051(4) | Pd3—Nb1xi | 0.31751(3) |
Se2—Pd3 | 0.25051(4) | ||
Bond | Angle/(°) | Bond | Angle/(°) |
Se2i—Nb1—Se2ii | 134.813 (8) | Pd3viii—Se2—Nb1 | 133.822 (3) |
Se2i—Nb1—Se2iii | 79.58 (2) | Nb1viii—Se2—Nb1 | 83.434 (16) |
Se2ii—Nb1—Se2iii | 83.434 (16) | Pd3vii—Se2—Nb1vii | 76.881 (6) |
Se2i—Nb1—Se2iv | 83.434 (16) | Pd3—Se2—Nb1vii | 133.822 (3) |
Se2ii—Nb1—Se2iv | 79.58 (2) | Pd3viii—Se2—Nb1vii | 133.822 (3) |
Se2iii—Nb1—Se2iv | 134.813 (7) | Nb1viii—Se2—Nb1vii | 83.434 (16) |
Se2i—Nb1—Se2 | 83.433 (16) | Nb1—Se2—Nb1vii | 83.434 (16) |
Se2ii—Nb1—Se2 | 134.812 (7) | Se2—Pd3—Se2ix | 92.612 (17) |
Se2iii—Nb1—Se2 | 134.812 (8) | Se2—Pd3—Se2iv | 87.388 (17) |
Se2iv—Nb1—Se2 | 83.433 (16) | Se2ix—Pd3—Se2iv | 180.0 |
Se2i—Nb1—Se2v | 134.812 (8) | Se2—Pd3—Se2x | 92.612 (17) |
Se2ii—Nb1—Se2v | 83.434 (16) | Se2ix—Pd3—Se2x | 87.388 (17) |
Se2iii—Nb1—Se2v | 83.434 (16) | Se2iv—Pd3—Se2x | 92.612 (17) |
Bond | Angle/(°) | Bond | Angle/(°) |
Se2iv—Nb1—Se2v | 134.812 (8) | Se2—Pd3—Se2i | 87.388 (17) |
Se2—Nb1—Se2v | 79.58 (2) | Se2ix—Pd3—Se2i | 92.612 (17) |
Se2i—Nb1—Pd3vi | 129.790 (11) | Se2iv—Pd3—Se2i | 87.388 (17) |
Se2ii—Nb1—Pd3vi | 50.210 (11) | Se2x—Pd3—Se2i | 180.0 |
Se2iii—Nb1—Pd3vi | 50.210 (11) | Se2—Pd3—Se2xi | 180.0 |
Se2iv—Nb1—Pd3vi | 129.790 (11) | Se2ix—Pd3—Se2xi | 87.388 (17) |
Se2—Nb1—Pd3vi | 129.790 (11) | Se2iv—Pd3—Se2xi | 92.612 (17) |
Se2v—Nb1—Pd3vi | 50.210 (11) | Se2x—Pd3—Se2xi | 87.388 (17) |
Se2i—Nb1—Pd3 | 50.210 (11) | Se2i—Pd3—Se2xi | 92.612 (17) |
Se2ii—Nb1—Pd3 | 129.790 (11) | Se2—Pd3—Nb1 | 52.909 (12) |
Se2iii—Nb1—Pd3 | 129.790 (11) | Se2ix—Pd3—Nb1 | 127.092 (12) |
Se2iv—Nb1—Pd3 | 50.210 (11) | Se2iv—Pd3—Nb1 | 52.908 (12) |
Se2—Nb1—Pd3 | 50.210 (11) | Se2x—Pd3—Nb1 | 127.092 (12) |
Se2v—Nb1—Pd3 | 129.790 (11) | Se2i—Pd3—Nb1 | 52.908 (12) |
Pd3vi—Nb1—Pd3 | 180.0 | Se2xi—Pd3—Nb1 | 127.091 (12) |
Pd3vii—Se2—Pd3 | 87.388 (17) | Se2—Pd3—Nb1xi | 127.091 (12) |
Pd3vii—Se2—Pd3viii | 87.388 (17) | Se2ix—Pd3—Nb1xi | 52.908 (12) |
Pd3—Se2—Pd3viii | 87.388 (17) | Se2iv—Pd3—Nb1xi | 127.092 (12) |
Pd3vii—Se2—Nb1viii | 133.822 (2) | Se2x—Pd3—Nb1xi | 52.908 (12) |
Pd3—Se2—Nb1viii | 133.822 (3) | Se2i—Pd3—Nb1xi | 127.092 (12) |
Pd3viii—Se2—Nb1viii | 76.881 (6) | Se2xi—Pd3—Nb1xi | 52.909 (12) |
Pd3vii—Se2—Nb1 | 133.822 (2) | Nb1—Pd3—Nb1xi | 180.0 |
Pd3—Se2—Nb1 | 76.881 (6) |
Symmetry codes: (i) x, y+1, z; (ii) x-1, y, -z+1/2; (iii) x, y+1, -z+1/2; (iv) x-1, y, z; (v) x, y, -z+1/2; (vi) -x, -y+2, z-1/2; (vii) x+1, y, z; (viii) x, y-1, z; (ix) -x+1, -y+2, -z+1; (x) -x, -y+1, -z+1; (xi) -x, -y+2, -z+1.
Article Outline
黄冲, 赵伟, 王东, 卜克军, 王思顺, 黄富强. Pd插层NbSe2化合物的制备、晶体结构和电学性质研究[J]. 无机材料学报, 2020, 35(4): 505. Chong HUANG, Wei ZHAO, Dong WANG, Kejun BU, Sishun WANG, Fuqiang HUANG.