Compact optoelectronic oscillator based on a Fabry–Perot resonant electro-optic modulator
Download: 957次
Microwave oscillators have been widely used in the field of radar[1], communications[2], navigation[3], electronic warfare[4], and modern physics experiments[5]. However, as wireless communication technology rapidly develops, the traditional microwave oscillators can no longer meet the high performance requirements, such as high output frequency and low phase noise. As an excellent low phase noise radio frequency (RF) source, the optoelectronic oscillator (OEO) has been intensively investigated and has attracted great interest in recent years[6–
In this Letter, we proposed and experimentally demonstrated a novel compact OEO employing an FP resonant EO modulator. A high-quality FP resonator not only has the capability of storing optical energy, but also serves as a mode selector. It is relatively easy to manufacture an FP resonator and to couple the light into it when compared with a WGM resonator[21]. In addition, the integration of an FP resonator and an EO modulator helps to reduce the system complexity significantly, which also makes the oscillator more portable and compatible with a temperature control module. In the proof-of-concept experiment, the characteristics of the novel optical storage link have been theoretically and experimentally investigated. Based on the proposed compact OEO, 10 and 20 GHz signals oscillate respectively with corresponding single-sideband (SSB) phase noise below
Figure
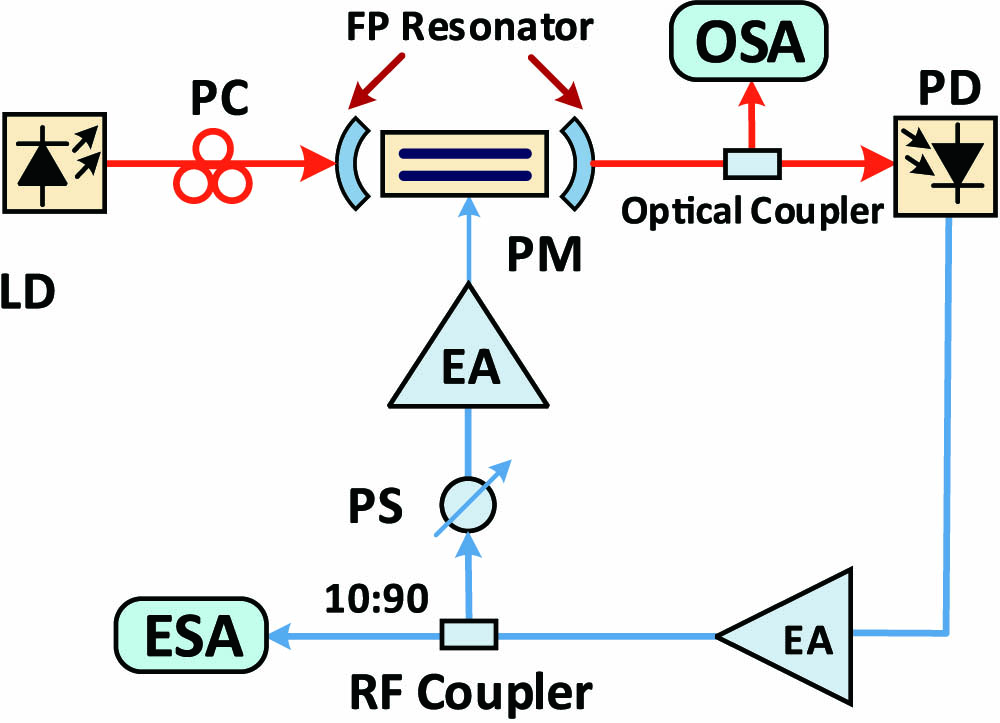
Fig. 1. Schematic diagram of the proposed compact OEO. LD: laser diode; PC: polarization controller; OSA: optical spectrum analyzer; PM: phase modulator; PD: photodiode; EA: electrical amplifier; PS: phase shifter; ESA: electrical spectrum analyzer.
When the modulation frequency is a multiple of the FSR of the FP resonator, the output optical signal after oscillating in the FP–EO modulator for
After several round trips, the total output optical field is
If the laser wavelength was set at a proper position, the gain of the optical segment would achieve maximum value, as shown in
The loop length can be adjusted via the phase shifter in the electrical chain, which would help to match the oscillation frequency with the frequency selection mode of the optical storage element. Assisted by the coupler, part of the oscillating signal can be sent out, while the rest would be fed back into the loop. Thanks to the frequency selection and high quality characteristic of the FP–EO modulator, the ultra-narrow RF BPF and long optical fiber coil are not required. In addition, the proposed OEO can be more compact via the integration of the phase modulator and FP resonator.
As mentioned above, the FP–EO modulator is the key part of our OEO. Both the optical resonance and electrical transmission characteristics play important roles for the energy storage and frequency selection. The corresponding measurement setups for resonance and filtering characteristics are in an open loop configuration. First, for measuring the optical power resonance, the setup consists of a frequency fine-tuning laser source with a narrow linewidth, an electrical function generator, a photodiode, and a high-speed resolution oscilloscope. The light wave generated from a 1550 nm tunable diode laser (New Focus TLB-6728) at a power level of 30 mW is injected into the FP–EO modulator (Opto Comb WTEC-01). The narrow linewidth and wide tuning resolution of the laser are 200 kHz and 0.01 nm, respectively. In addition, the tuning parameter of the laser is about 0.04 nm/V. Then the laser wavelength is scanned by driving the laser current with a 60 Hz triangular signal generated from the function generator (Tektronix AFG3151 C). As the blue line shows in Fig.

Fig. 2. Laser wavelength scanning voltage and corresponding optical resonance spectrum of the FP resonator.
Second, for measuring the electrical transmission response of the optical storage element employing the FP–EO modulator, the measuring setup consists of a tunable laser diode, a photodiode, and a vector network analyzer. A narrow linewidth laser with a 40 kHz linewidth and at a power level of 14 dBm is used as the laser source. The light wave is fed into the FP–EO modulator driven by the RF signal generated from the output port of the vector network analyzer (Agilent N5244A). In addition, both the wavelength of the laser and the bias voltage of the modulator are finely tuned to meet Eq. (
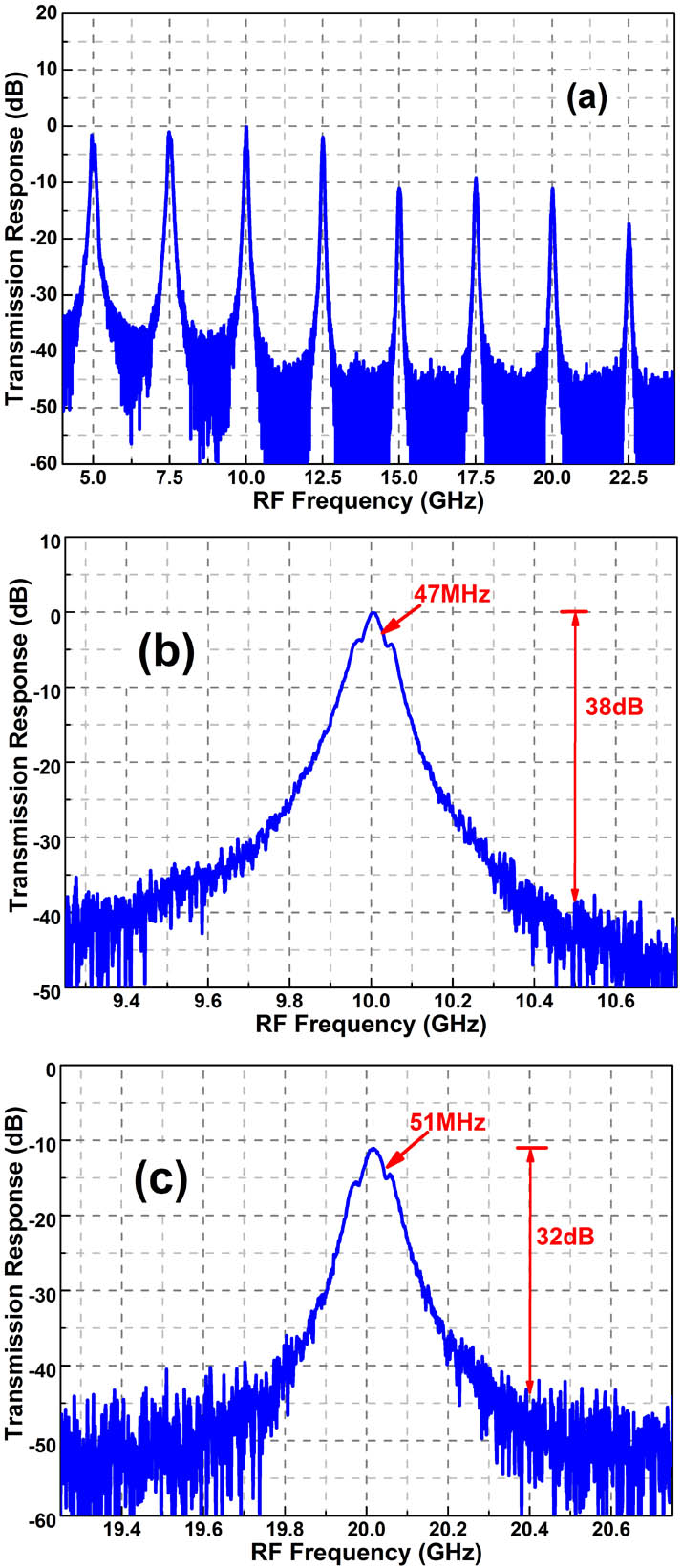
Fig. 3. Measured transmission response of the optical link employing an FP resonant EO modulator. (a) Transmission response of the proposed optical storage link from 4.5 to 23 GHz. (b) Zoomed-in view of the response centered at 10 GHz. (c) Zoomed-in view of the response centered at 20 GHz.
The system operation principle has been theoretically described and the characteristics of the optical segment have been measured earlier. Then a proof-of-concept experiment based on the configuration in Fig.
The amplifiers are used to provide sufficient electrical gain in the loop. The gain of the first stage amplifier is about 30 dB from DC to 26 GHz; while for the second amplifier it depends on the oscillating frequency. For the 10 GHz oscillation frequency, a microwave amplifier from 9 to 12 GHz with a 25 dB gain is used, while it was replaced by another power amplifier from 18 to 22 GHz with a 30 dB gain in the 20 GHz oscillation loop. For the RF phase shifter, it is operated manually with a 900/GHz tuning parameter from DC to 26.5 GHz. By finely tuning the phase shifter and the laser wavelength, 10 and 20 GHz microwave signals can be generated, respectively. Then, for measuring the optical spectra of the proposed OEO, 10% of the optical signal from the 10 dB optical coupler is injected into the optical spectrum analyzer (Yokogawa AQ6370C). Just as shown in Fig.

Fig. 4. Normalized optical power spectrum of the proposed compact OEO. (a) Optical spectra of (a) 10 and (b) 20 GHz oscillation loop.
Finally, the RF signal is produced by the frequency comb on a high-speed photodiode. The corresponding electrical spectrum and phase noise are taken by a 40 GHz RF spectrum analyzer (Agilent 9030A) via a fraction of the generated RF signal output from the 10 dB microwave coupler. Meanwhile, the rest of the microwave signal is fed back into the optoelectronic hybrid oscillating loop. In Fig.
The SSB phase noise performance of the proposed OEO has also been investigated. As shown in Fig.

Fig. 6. SSB phase noise of the oscillation frequencies at (a) 10 and (b) 20 GHz generated by the proposed compact OEO.
The feasibility of the novel compact RF photonic oscillator has been verified. The phase noise performance is mainly limited by the Q value of the FP resonator and the noise figure of the optical storage element. By increasing the resonator’s quality factor, it can help to improve the phase noise performance of the final oscillating signal. The insertion loss of the embedded phase modulator is the main limitation of the FP resonator’s high quality factor. Finally, a stable FP resonator is useful for improving the stability of the OEO, as described in Ref. [18].
In conclusion, we propose and experimentally demonstrate a novel compact OEO employing an FP–EO modulator, which serves as both the energy storage and frequency selection elements. Due to the integration of a modulator and an FP resonator, the novel compact OEO can be realized without a long optical fiber and a large RF narrow BPF. A proof-of-concept experiment is investigated to prove the feasibility of the proposed OEO. Based on the frequency selection characteristics of the optical storage link, the OEO oscillates at 10 and 20 GHz, respectively, and the corresponding SSB noise can reach
[1]
[2]
[3]
[4]
[5]
[6]
[7]
[8]
[9]
[10]
[11]
[12]
[13]
[14]
[15]
[16]
[17]
[18]
[19]
[20]
Jian Dai, Yitang Dai, Feifei Yin, Yue Zhou, Jianqiang Li, Yuting Fan, Kun Xu. Compact optoelectronic oscillator based on a Fabry–Perot resonant electro-optic modulator[J]. Chinese Optics Letters, 2016, 14(11): 110701.