双余弦相位调制对超高斯脉冲的频谱压缩
下载: 785次
1 引言
在光子学中,高亮度频谱和高分辨率的光源非常重要,它在非线性显微镜、超快频谱和高速通信等方面具有广泛应用。实现高亮度频谱和高分辨率的方法是将脉冲进行频谱压缩,并将能量重新分布到更窄的频谱范围中。例如,在高非线性光纤中,可基于拉曼效应诱导的自频移机制有效压缩超短脉冲频谱[1-2]。在反常色散渐增光纤中,利用色散渐减光纤中时域绝热压缩的相反过程[3-6],基态孤子也可以获得频谱压缩[7-8]。频谱压缩还可以通过自相位调制效应引起的正啁啾和初始脉冲的负啁啾之间相互补偿来实现[9-11]。这种方法已被应用到标准单模光纤、光学增益光纤、光子晶体光纤和梳型光纤中[12-18]。
一般情况下,光纤中色散引起的初始负啁啾与非线性导致的正啁啾之间的比例失调会引起频谱基座出现,它可能包含了输出频谱一半的能量[8,14]。基座中的频谱旁瓣会使频谱亮度和分辨率降低。一些实验方案和装置可用于实现频谱基座抑制以及频谱压缩,例如:采用抛物线型脉冲作为初始脉冲[14,17];选择传输中脉冲趋向于矩形的区域作为输出频谱[19-20];单模光纤和色散位移光纤串联组合的梳型光纤[18];高非线性光纤和非线性光学环镜互连的结构装置[21]。
Boscolo的研究表明,在外部对高非线性光纤中的脉冲进行余弦相位调制来抑制输出频谱的基座,可以提高频谱的压缩[22]。值得注意的是,具有初始线性负啁啾的高斯脉冲在高非线性光纤中的频谱分裂为规则的频谱旁瓣,可以通过单个余弦进行相位调制来减小频谱旁瓣的强度。但初始为非线性负啁啾的脉冲将会使频谱分裂成更复杂的图样,频谱压缩很难通过单一的余弦进行相位调制。本文以非线性负啁啾的超高斯脉冲作为初始条件,研究其在高非线性光纤中的频谱分裂特征,分析其瞬时相位规律,通过对其进行双相位调制来实现脉冲频谱压缩和优化。
2 研究模型
光纤系统中皮秒量级脉冲频谱压缩的理论研究基于标准的非线性薛定谔方程[23]:
式中
式中
式中
脉冲在光纤中频谱窄化的程度可通过压缩因子
3 超高斯脉冲在非线性占主导的光纤中的频谱演化
在非线性占主导的光纤中,即(1)式中只考虑
其中瞬时相位为
式中
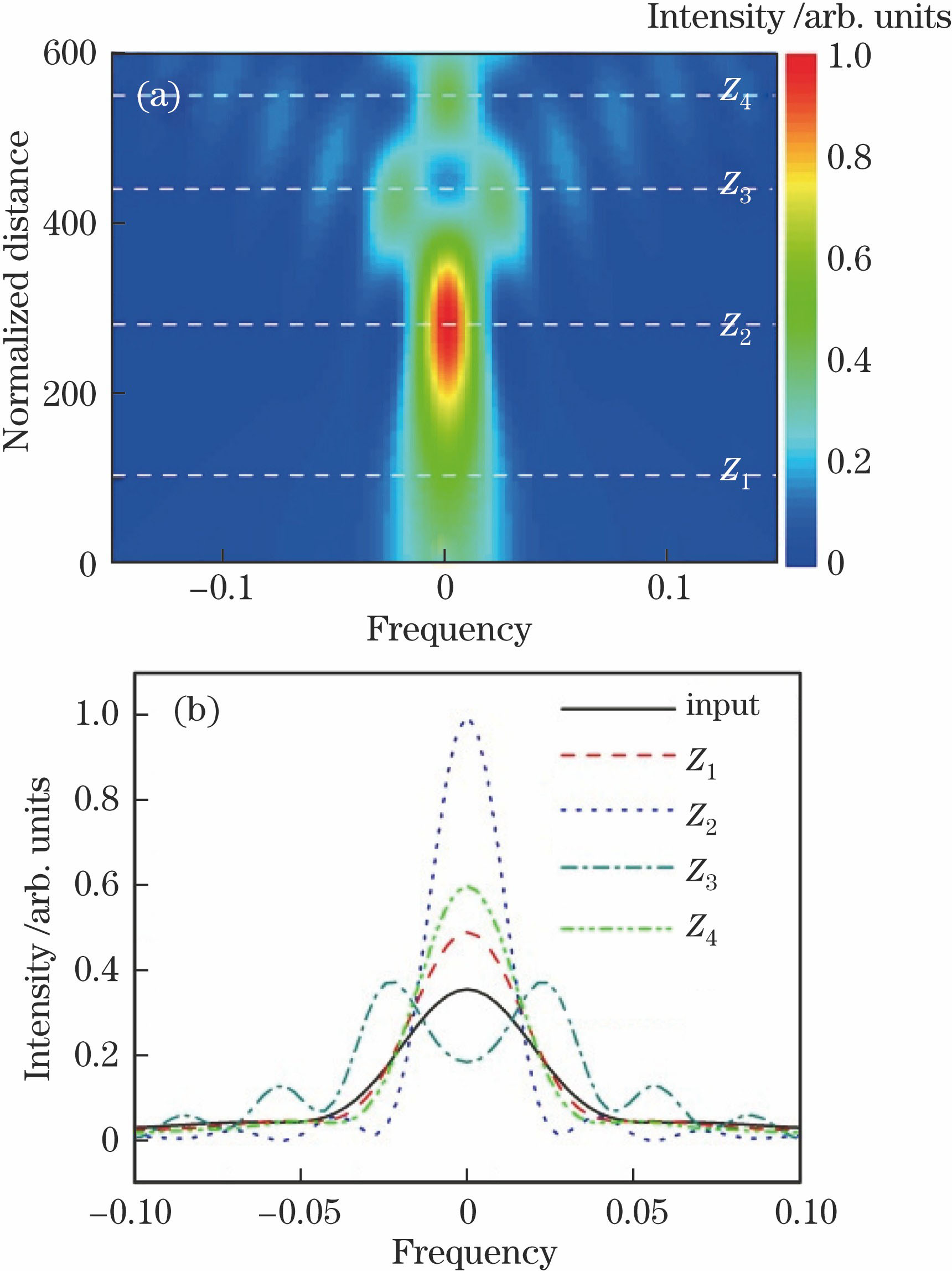
图 1. (a)频谱强度随距离的演化;(b)光纤入口处和不同传输距离处的频谱强度分布
Fig. 1. (a) Evolution of spectral intensity with distance; (b) spectral intensity profiles at the entrance and the different propagation distances
为了分析这些特征,给出了对应的频谱压缩参数
上述结果表明,超高斯脉冲在非线性占主导的光纤中传输时,其频谱只在

图 2. (a) FWHM压缩因子;(b) RMS压缩因子;(c)斯特列比
Fig. 2. (a) FWHM spectral compression factor; (b) RMS spectral compression factor; (c) Strehl ratio
下面从脉冲演化过程来考察时域相位分布与频谱压缩的关联。
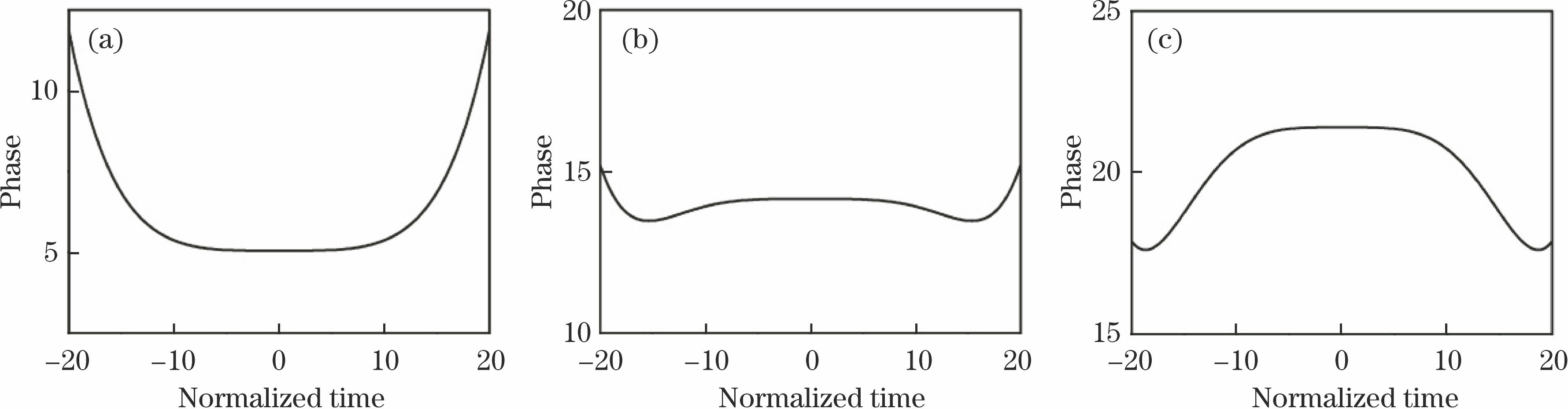
图 3. 在不同传输距离处的脉冲时域相位分布。 (a) Z1处;(b) Z2处;(c) Z3处
Fig. 3. Temporal phase profiles at the different propagation distances. (a) Z1; (b) Z2; (c) Z3
4 外部相位调制对频谱压缩的优化
如前所述,为了获得高质量的频谱压缩,需要消除这个平台,使其相位在脉冲中心区间内变得平坦。研究表明,高斯脉冲在非线性占主导的光纤中传输时,其频谱产生旁瓣是由于初始线性啁啾受自相位调制啁啾作用出现了近似正弦的变化,因此可以通过外部单个余弦相位调制对高斯脉冲进行相位补偿,使其变得平坦[22]。但是对于非正弦的超高斯脉冲啁啾,单个相位调制是比较困难的。为了解决这个问题,考虑到频谱压缩质量降低的相位不平坦主要发生在脉冲中心区间,故而将超高斯脉冲的相位在有限区间[
其中,
式中
通过大量数值实验可以发现,当
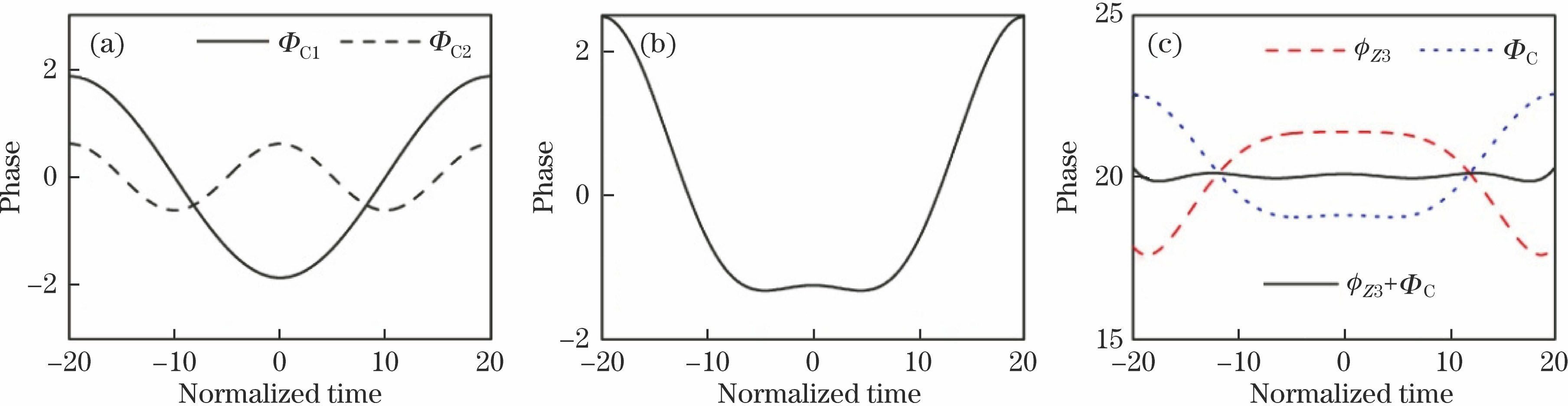
图 4. (a)调制相位ΦC1和ΦC2分布图;(b)双余弦调制相位ΦC分布图;(c)补偿后的相位在Z3处的分布图
Fig. 4. (a) Distribution of the modulation phases ΦC1 and ΦC2; (b) distribution of the dual cosine modulation phase ΦC; (c) distribution of the compensated phase at the propagation distance Z3
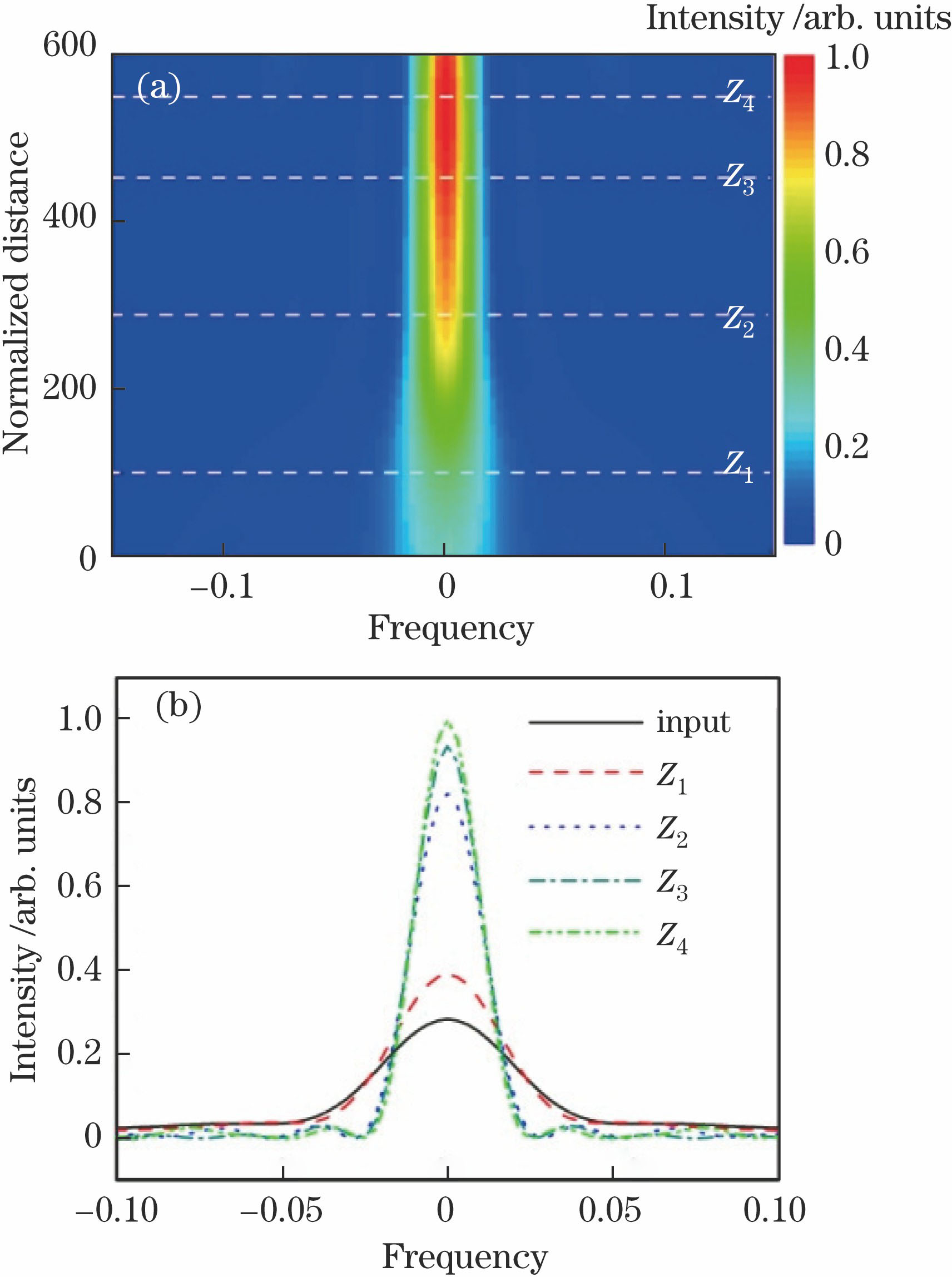
图 5. (a)相位补偿后频谱强度随距离的演化;(b)相位补偿后光纤入口处和不同传输距离处的频谱强度分布
Fig. 5. (a) Evolution of spectral intensity with distance after phase correction; (b) spectral intensity profiles at the entrance and different propagation distances after phase correction
以上给出了用双余弦相位对原脉冲在
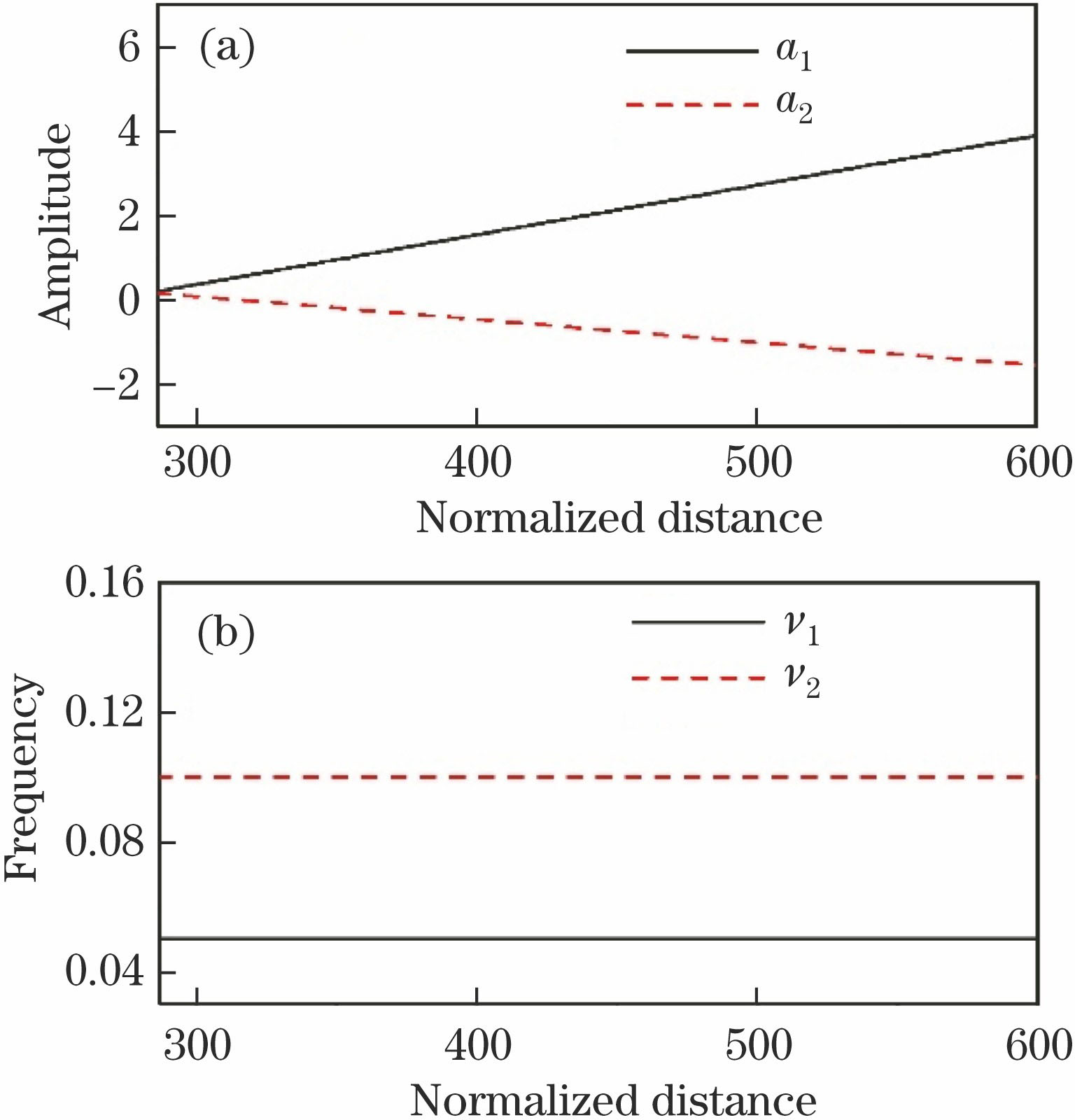
图 6. 调制相位ΦC1和ΦC2的振幅和频率随距离的演化。(a)振幅a1和a2的演化;(b)频率ν1和ν2的演化
Fig. 6. Evolutions of the amplitudes and frequencies of the modulation phases ΦC1 and ΦC2 with distance. (a) Evolutions of the amplitudes a1 and a2; (b) evolutions of the frequencies ν1 and ν2
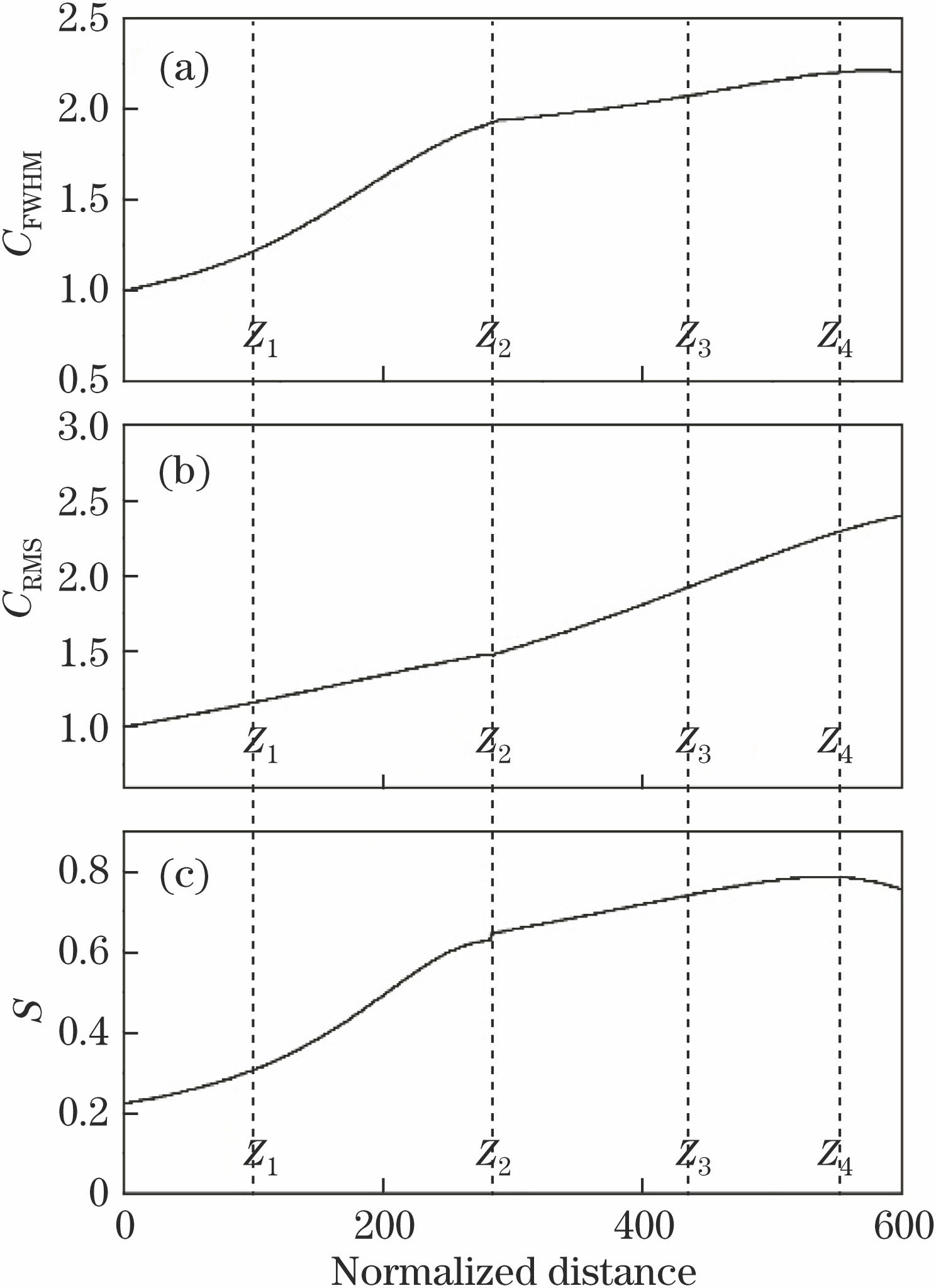
图 7. 相位补偿后各参数随距离的演化。(a) FWHM压缩因子;(b) RMS压缩因子;(c)斯特列比
Fig. 7. Variation of parameter with distance after phase correction. (a) FWHM spectral compression factor; (b) RMS spectral compression factor; (c) Strehl ratio

图 8. 斯特列比S随展宽因子和传输距离的演化。(a)相位补偿前;(b)相位补偿后
Fig. 8. Evolution of Strehl ratio with stretching factor and the propagation distance. (a) Without phase correction; (b) with phase correction
最后,研究了当
5 结论
提出了一种在高非线性光纤中对具有非线性啁啾超高斯脉冲进行频谱压缩的优化方法。在高非线性光纤中,由于脉冲初始非线性啁啾与自相位调制引起的非线性啁啾之间不匹配导致了不平坦的时间相位,脉冲频谱的两翼出现了振荡,使频谱压缩受限。通过外部的双余弦相位调制,可使脉冲中心区间的相位趋于平坦,优化频谱压缩效果。比较相位调制前后各频谱压缩参数可知压缩质量得到了改善。外部的双余弦相位调制可以由受同步微波信号驱动的铌酸锂相位调制器或具有正弦信号的脉冲交叉相位调制器来实现。同时,在更大的展宽因子范围下,超高斯脉冲的压缩质量也得到了明显改善。此外,进一步的研究得出,所提方法对更高陡度的超高斯脉冲同样有效。实验结果对皮秒超高斯脉冲在高非线性光纤中实现频谱压缩优化具有一定的理论指导作用。
[2] Fedotov A B, Voronin A A, Fedotov I V, et al. Spectral compression of frequency-shifting solitons in a photonic-crystal fiber[J]. Optics Letters, 2009, 34(5): 662-664.
Fedotov A B, Voronin A A, Fedotov I V, et al. Spectral compression of frequency-shifting solitons in a photonic-crystal fiber[J]. Optics Letters, 2009, 34(5): 662-664.
[3] 贾东方, 谈斌, 王肇颖, 等. 基于绝热孤子压缩效应的小基座孤子脉冲研究[J]. 光学学报, 2006, 26(2): 166-170.
贾东方, 谈斌, 王肇颖, 等. 基于绝热孤子压缩效应的小基座孤子脉冲研究[J]. 光学学报, 2006, 26(2): 166-170.
[4] 张浩, 谢康, 姜海明. 基于色散渐减、非线性渐增原理的脉冲压缩[J]. 中国激光, 2008, 35(12): 1951-1954.
张浩, 谢康, 姜海明. 基于色散渐减、非线性渐增原理的脉冲压缩[J]. 中国激光, 2008, 35(12): 1951-1954.
[5] 曹文华, 徐平, 刘颂豪. 马赫-曾德尔型色散渐减光纤干涉仪的孤子效应脉冲压缩研究[J]. 光学学报, 2011, 31(4): 0419001.
曹文华, 徐平, 刘颂豪. 马赫-曾德尔型色散渐减光纤干涉仪的孤子效应脉冲压缩研究[J]. 光学学报, 2011, 31(4): 0419001.
[6] 王丽, 杨荣草, 贾鹤萍, 等. 色散渐减光纤链中孤子的周期集总放大和恢复[J]. 光学学报, 2017, 36(6): 0619001.
王丽, 杨荣草, 贾鹤萍, 等. 色散渐减光纤链中孤子的周期集总放大和恢复[J]. 光学学报, 2017, 36(6): 0619001.
[16] Li HP, Zhang XJ, Liao JK, et al. Spectral compression of femtosecond pulses in photonic crystal fibers with anomalous dispersion[C]. SPIE, 2009, 7630: 76301I.
Li HP, Zhang XJ, Liao JK, et al. Spectral compression of femtosecond pulses in photonic crystal fibers with anomalous dispersion[C]. SPIE, 2009, 7630: 76301I.
[23] Agrawal GP, Nonlinear fiber optics[M]. 3rd ed. New York: Academic Press, 2001.
Agrawal GP, Nonlinear fiber optics[M]. 3rd ed. New York: Academic Press, 2001.
[25] Godil A A, Auld B A, Bloom D M. Picosecond time-lenses[J]. IEEE Journal of Quantum Electronics, 1994, 30(3): 827-837.
Godil A A, Auld B A, Bloom D M. Picosecond time-lenses[J]. IEEE Journal of Quantum Electronics, 1994, 30(3): 827-837.
[26] Plansinis B. DonaldsonW R, Agrawal G P. Spectral changes induced by a phase modulator acting as a time lens[J]. Journal of the Optical Society of America B, 2015, 32(8): 1550-1554.
Plansinis B. DonaldsonW R, Agrawal G P. Spectral changes induced by a phase modulator acting as a time lens[J]. Journal of the Optical Society of America B, 2015, 32(8): 1550-1554.
Article Outline
王蕾, 杨光晔, 任晋萍, 罗焕波, 李禄. 双余弦相位调制对超高斯脉冲的频谱压缩[J]. 光学学报, 2018, 38(3): 0319001. Wang Lei, Yang Guangye, Ren Jinping, Luo Huanbo, Li Lu. Spectral Compression of Super-Gaussian Pulse by Dual Cosinoidal Phase Modulation[J]. Acta Optica Sinica, 2018, 38(3): 0319001.