Photonic generation of background-free millimeter-wave ultra-wideband signals (Invited Paper)
Download: 703次
Benefiting from low power consumption, immunity to multipath fading, carrier free, and high data rate, ultra-wideband (UWB) has attracted more and more attention for short-range high-capacity wireless communication and sensor networks[1]. The Federal Communications Commission (FCC) defined the UWB signal as a radio frequency (RF) signal that occupies a spectral bandwidth of more than 500 MHz or more than 20% fractional bandwidth with a power density no more than
In this Review, we review recent progress on the photonic generation of UWB signals in the MMW band with emphasis on the generation of background-free and FCC compliant MMW-UWB pulses.
The electrical spectrum of MMW-UWB signals for outdoor communications spreads from 22 to 29 GHz. Generally, it can be realized by frequency upconversion of a baseband signal to the local oscillator (LO) band since an electrical mixer can cover this frequency range easily. Actually, the electrical mixer-based approach is more mature and easily available for us. Thus, we will first check the possibility of the electrical mixer-based approach for the generation of MMW-UWB signals.
The mixer available in our lab has an intermediate frequency (IF) bandwidth from dc to 8 GHz, an LO bandwidth from 14 to 26 GHz, and an RF bandwidth from 14 to 26 GHz. Due to the limited bandwidth of the mixer, we tried to upconvert the baseband signal to the LO band from 19 to 26 GHz that is 3 GHz lower than the FCC standard. The baseband signal has a 10 dB bandwidth of 7 GHz, while the frequency of the LO signal is 22.5 GHz.
The electrical spectrum of the upconverted signal is shown in Fig.

Fig. 1. Measured (a) electrical spectrum and (b) waveform of the upconverted UWB signal using an electrical mixer.
It is known that the main limitation of the UWB technology is the limited transmission distance, which is around 10 m. In order to extend the transmission distance and to overcome the limitation of the electrical mixer-based approach, photonic generation of the UWB signal as well as UWB-over-fiber has been proposed[3,4]. Photonic frequency upconversion, which is similar to the electrical method, has been widely used for MMW-UWB signal generation. It has been reported that the MMW-UWB signal can be generated based on an optical parametric amplifier (OPA)[8] and four-wave mixing in a highly nonlinear photonic crystal fiber[12]. However, the signals always suffer from the residual LO signal that also exists in the electrical mixer based method.
In order to suppress the residual LO signal, we have proposed a dual-parallel Mach—Zehnder modulator (DPMZM) based a photonic MMW-UWB generator[11], as shown in Fig.
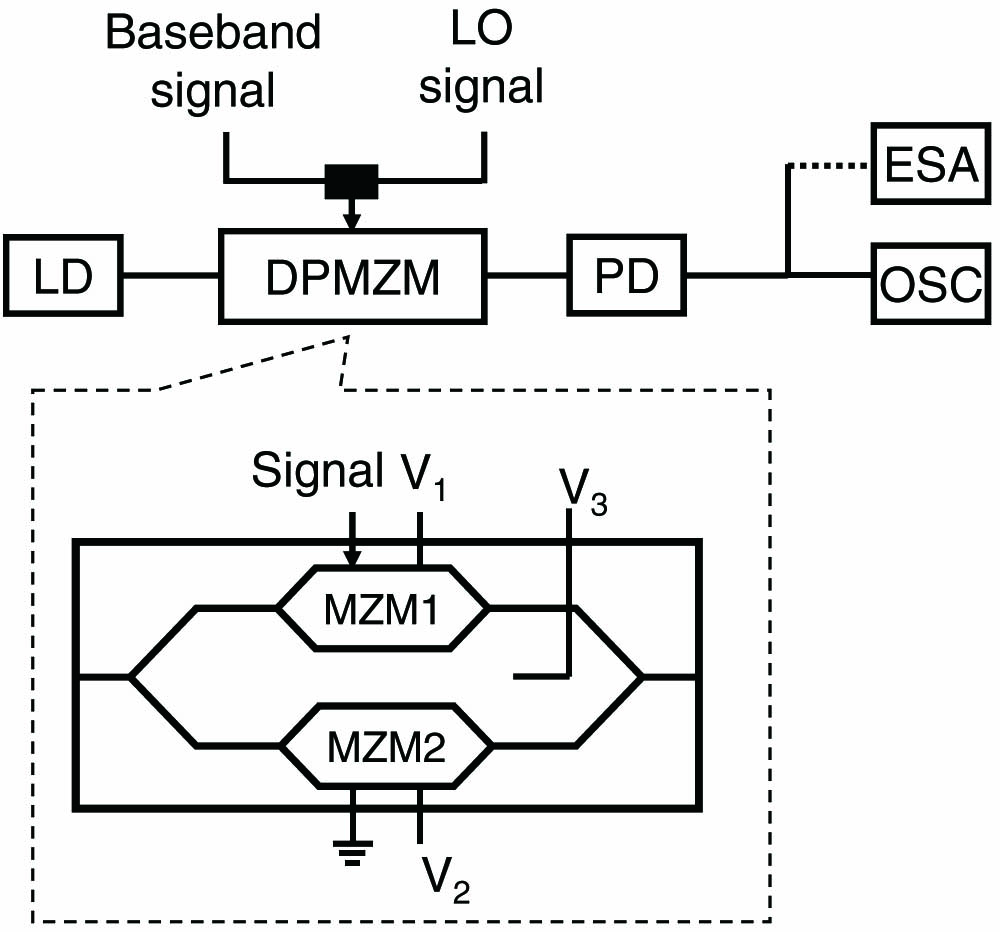
Fig. 2. MMW-UWB generator based on the DPMZM; the layout of the DPMZM is shown in the inset.
In this scheme, MZM1 is biased at the linear transmission point. Thus, the baseband signal can be upconverted to the LO band without distortion. MZM2 has no driven signal to allow the pure optical carrier to pass through. The carrier power at the MZM2 branch can be adjusted by tuning the bias voltage of MZM2. The phase difference between the two sub-MZMs is set to be 180°. by adjusting the bias voltage of the parent MZM. In this way, the optical carriers from MZM1 and MZM2 are destructively interfered with. It means that the power of the optical carrier at the output of the DPMZM can be controlled separately. The optical carrier contributes significantly to the residual LO component. The proposed method provides a new way to control the power of the LO signal.
Figure
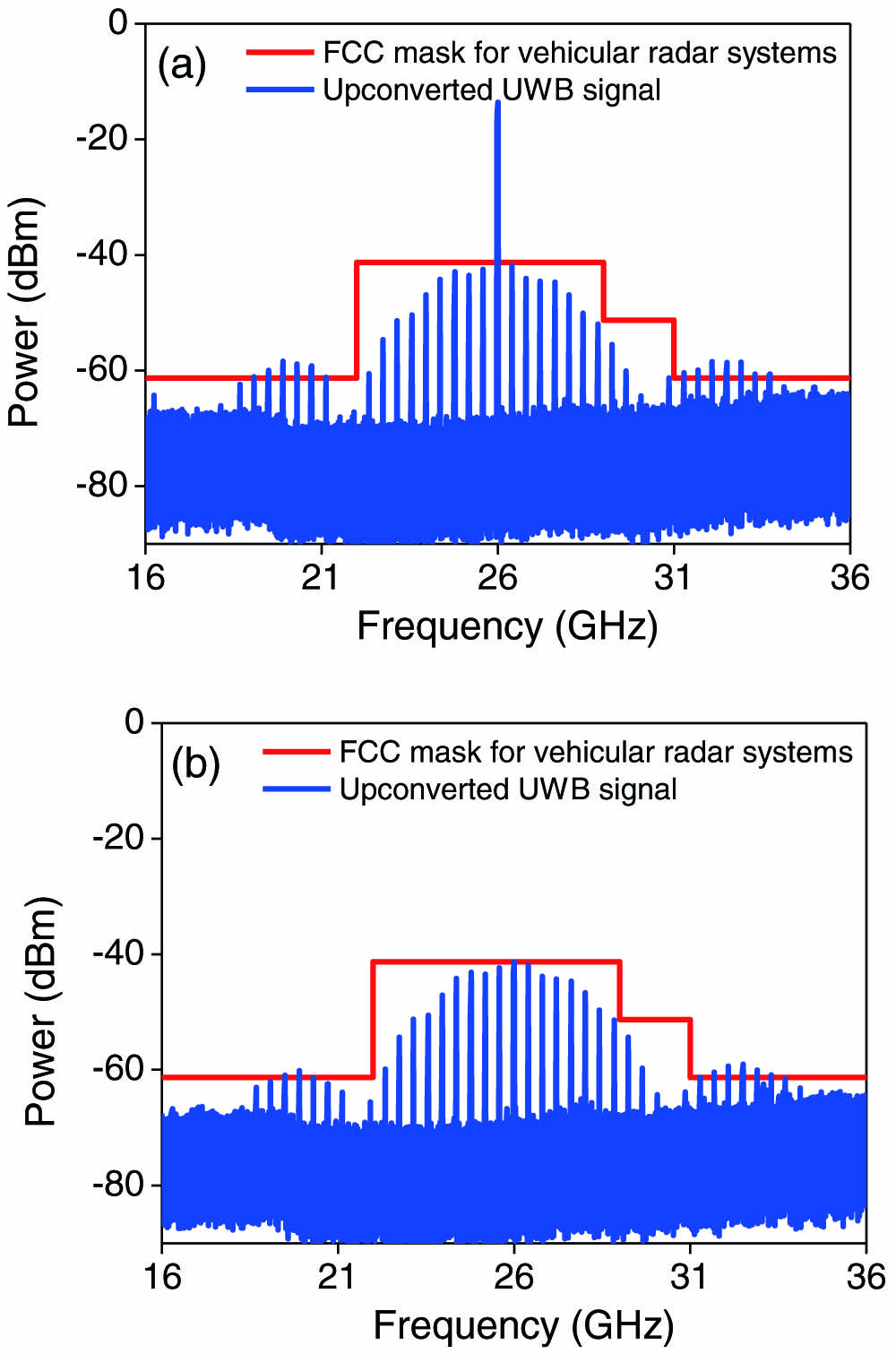
Fig. 3. Measured electrical spectra of the upconverted UWB signals based on the DPMZM approach when the dc bias of MZM2 is set at (a) the minimum transmission point (0.95 V) and (b) , respectively.
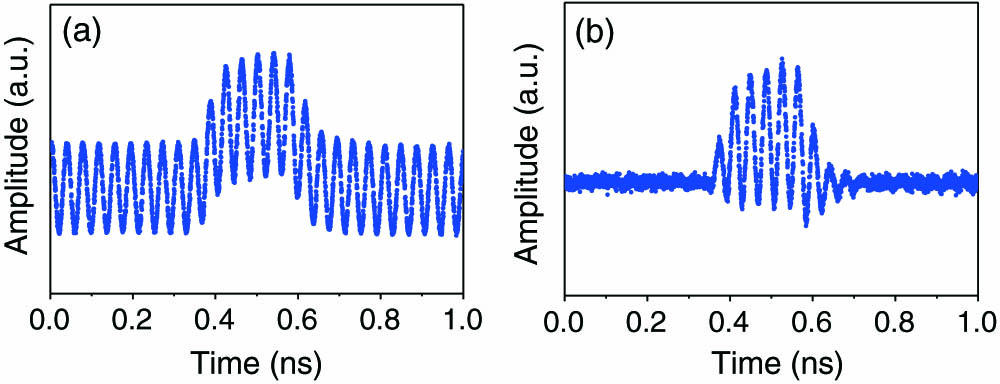
Fig. 4. Measured waveforms of the upconverted UWB signals when the dc bias of MZM2 is set at (a) the minimum transmission point (0.95 V) and (b) , respectively.
It is worth noting that the amplitude of the generated MMW-UWB signal has been modulated using this scheme, as can be clearly seen from Fig.
The DPMZM-based MMW-UWB generator suffers from a background signal. In Ref. [16], we have demonstrated a background-free MMW-UWB generator using two cascaded polarization modulators (PolMs) and an optical bandpass filter (OBPF). The OBPF is added to realize a power balance between the optical carrier and the first-order sideband. The use of the OBPF makes the system unstable. In this section, a similar MMW-UWB generator is discussed without using an OBPF[17]. The schematic diagram of the signal generator is shown in Fig.
The polarization states of the optical carrier and the first-order sidebands are aligned with the two principal axes of PolM2. In this way, an opposite phase shift is introduced to the optical carrier and sidebands. The Pol oriented at an angle of 45° to one principal axis of the PolM2 is added to combine the optical carrier and the sidebands to a fixed polarization state. The optical signal at the output of the Pol is written as
The photocurrent has both dc and ac parts. The dc part is constant all the time, which means that the baseband signal is eliminated. In the ac part, the LO signal is recovered for data “1,” while the LO signal is removed for data “0.” In this way, the residual LO can be well suppressed. A MMW-UWB signal can be generated as shown in the inset of Fig.
In the experiment, the PolM2 was replaced by a phase modulator (PM) since only one PolM was available in our lab. For PM, we have
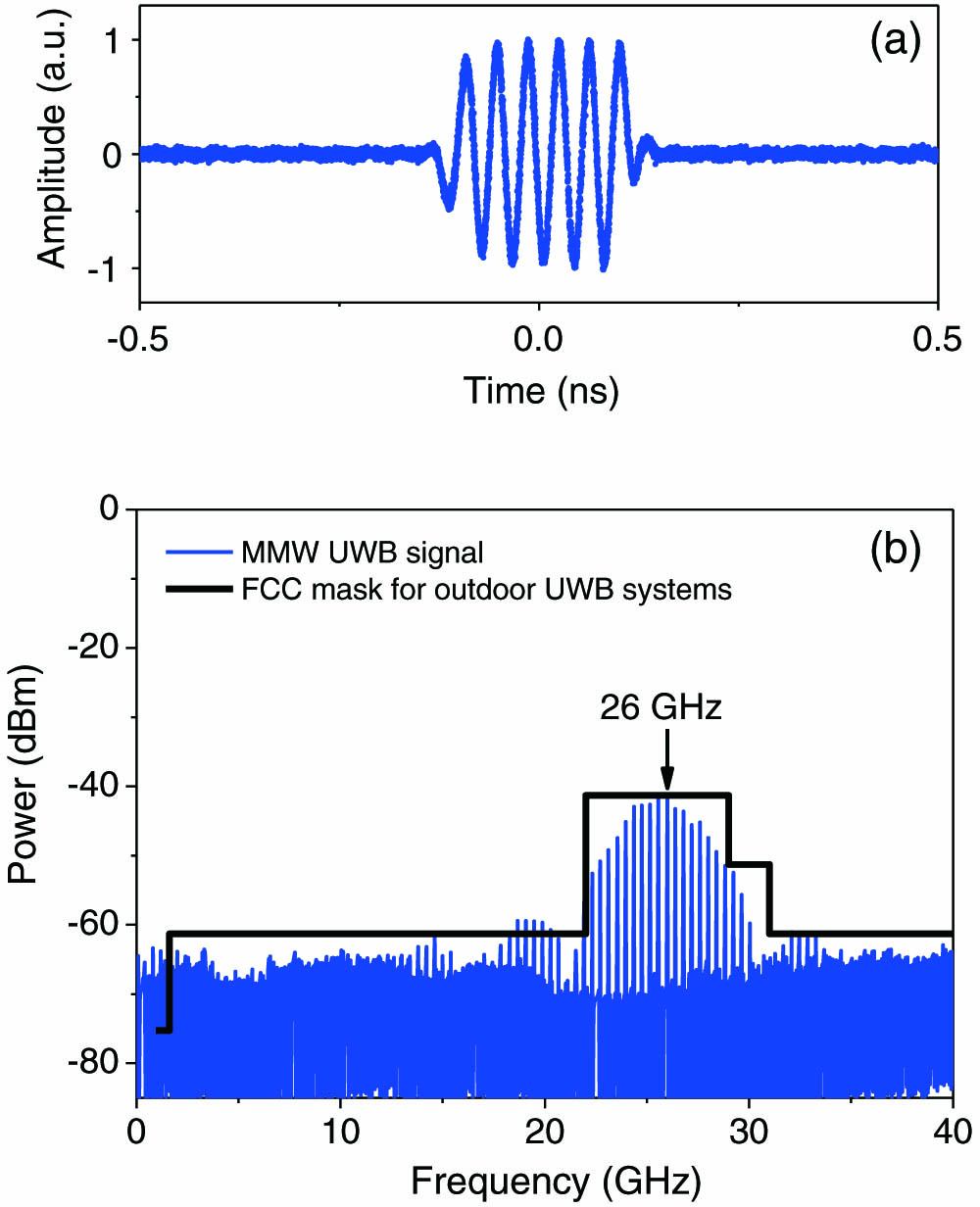
Fig. 6. Measured (a) waveform and (b) electrical spectrum of the MMW-UWB signal based on cascaded polarization modulators.
The proposed method is very promising to fully agree with the FCC standard, as shown in Fig.
In order to enhance the stability of the system, a compact modulator is preferred. In Refs. [10,14], MMW-UWB generators using a DPMZM have been reported. However, sophisticated bias control is required, which is very complicated in practical applications. Here, we introduce an extremely simple MMW-UWB generator, see Fig.
The photocurrent consists of dc and ac signals. The dc part is related to the background signal. A background-free MMW-UWB signal means that the dc part should be constant. Thus,
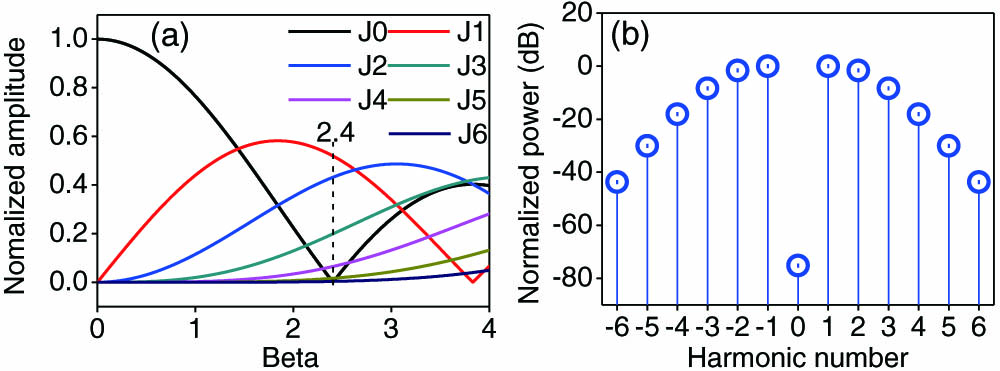
Fig. 8. Normalized (a) amplitude of sidebands versus and (b) power of different sidebands for .
Figure
The principle of this idea is quite simple and an experiment is also easy to carry out to verify the possibility of the UWB generator. One arm (arm1) of the DDMZM was fed by a pulse signal that was set as a 32-bit pattern “1110 0000 … 0000” (three “1’s” every 32 bits) at a speed of 13 Gb/s. The other arm (arm2) was driven by a 26 GHz sinusoidal microwave signal. Its power was optimized to satisfy
The PD used in the experiment has a bandwidth of 40 GHz. Figure
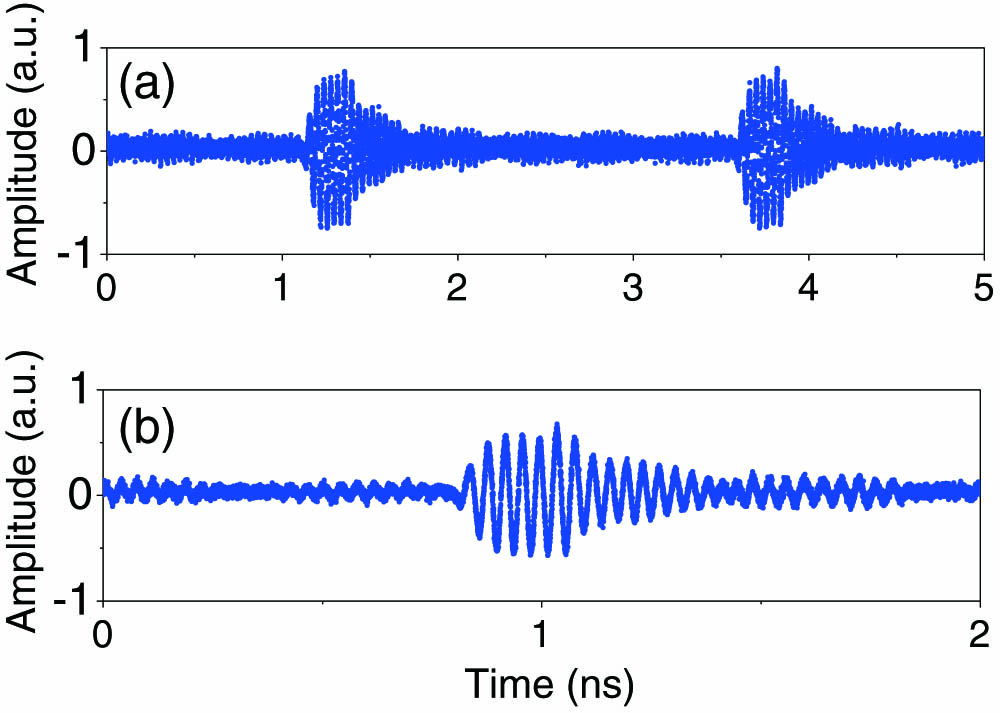
Fig. 9. Generated (a) MMW-UWB pulses and (b) the zoomed-in view based on a single modulator.
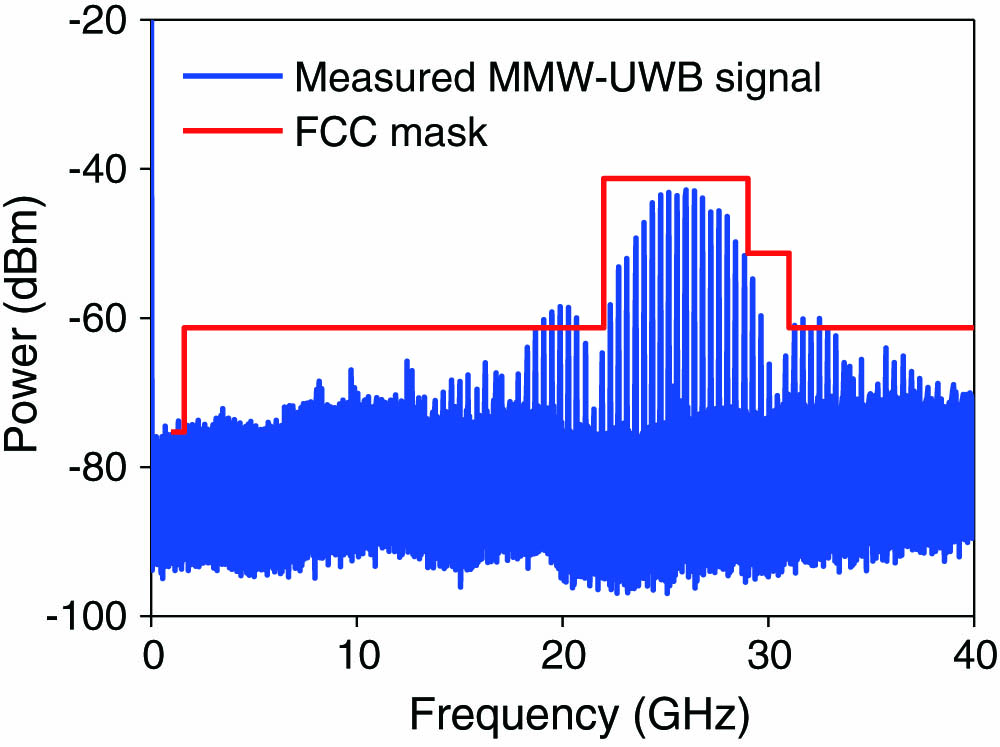
Fig. 10. Electrical spectrum of the MMW-UWB pulse as well as the FCC mask corresponding to the waveform shown in Fig. 9 .
We have discussed several MMW-UWB generators in this Review. The electrical mixer-based UWB generator has strong residual LO signal that is too hard to be suppressed. Many photonic-based approaches have been proposed to overcome the limitations associated with the electrical method. We have also made many efforts to generate background-free MMW-UWB signals. The DPMZM-based approach can suppress the residual LO signal perfectly. However, the baseband signal violates the FCC standard significantly. To eliminate both the baseband signal and the residual LO signal, we used two cascaded modulators to construct a high-speed microwave switch. A sinusoidal microwave signal was truncated into pulses without changing the optical power. In this way, we successfully generated an MMW-UWB pulse that satisfies the FCC requirement. A simpler method has also been developed using a single modulator. This structure has the potential for integration, which could make the UWB generator more compact and stable. For background-free UWB signal generation, the balanced photodetection method is also an alternative way[20]. The baseband signal is eliminated by power cancellation in a balanced PD. For UWB signal transmission, signal modulation is also very important. We have reported binary phase modulation of an MMW-UWB pulse using cascaded modulators[21] or a single modulator[22]. To generate a binary phase modulated MMW-UWB pulse, a three-level electrical pulse has been used.
Similar to an optical switch, the background-free MMW-UWB generator is equivalent to a microwave switch. High-speed switching can be easily realized using a broadband modulator. The microwave switch can also be programmable by programming the data pulse. On the other hand, the MMW-UWB generator is also equivalent to a frequency upconverter. The baseband signal is upconverted to the LO band without leaking of the baseband and LO signals.
[1]
[3]
[4]
[5]
[6]
[7]
[8]
[9]
[10]
[11]
[12]
[13]
[14]
[15]
[16]
[17]
[18]
[19]
[20]
[21]
[22]
Wei Li, Ming Li, Ninghua Zhu. Photonic generation of background-free millimeter-wave ultra-wideband signals (Invited Paper)[J]. Chinese Optics Letters, 2017, 15(1): 010007.