基于APD自适应增益控制的近地无线激光通信信道大气湍流抑制方法研究
下载: 626次
Owing to 5G, free-space optical communication (FSO) has high communication rate, long communication distance, strong anti-interference and low power consumption. It does not require laying fibre optic cables and has become the current research hot spot in wireless communication. For wireless free-space laser communication, the most severe problem is the impact of the atmospheric channel. The light intensity scintillation effect caused by atmospheric turbulence causes the communication data received by the receiver to be superimposed with jitter and fading, inevitably leading to degradation in communication quality. The front-end avalanche photodiode avalanche photodiode (APD) method is used in this study. In this technique, the reverse bias voltage is changed to control the multiplication factor of APD, which is essential in suppressing atmospheric turbulence and extending the dynamic range.
In order to improve the communication performance of laser communication systems under atmospheric channels, this study proposes a laser transmission terminal atmospheric turbulence suppression algorithm based on APD adaptive gain control (AGC). Additionally, we design an APD current feedback and bias control circuit and write a set of closed-loop regulation schemes. Furthermore, a wireless laser communication experiment with an adaptive gain control algorithm under medium and weak turbulence is developed.
A laser communication test with a 500 m communication distance was conducted at Huawei’s Songshan Lake base in Dongguan, Guangdong Province. The average temperature was 25 ℃ and weather was cloudy and breezy weather on the experiment day. The experimental system consists of an APD receiver and laser diode (LD) transmitter (Fig. 5). The probability distributions and power spectra were tested under 500 m of weak turbulence, with fixed APD gain and adaptive APD gain control, respectively. The results show that the optical power distribution ranges from 1000 to 40, 000 nA (Fig. 6) under weak turbulence conditions, with a log-normal probability density curve. In a typical Gaussian distribution, the light intensity is concentrated at 10, 00020, 000 nA (Fig. 6), with a maximum range of less than 20, 000 nA, when APD gain adaptive control is added. The optical intensity flicker variance is reduced from 0.057 to 0.023 (Fig. 7). The power spectrum shows that the slope of the curve with gain control is -1.056, and the slope of the curve without gain control is -0.364. The flatter the slope, the lower is the noise suppression effect. Thus, the power spectrum with automatic gain control is more consistent with the 5/3 power-law spectrum (Fig.8), and the system bit error rate (RBE) is reduced by two orders of magnitude (Fig.9). Furthermore, the flicker variance and BER were tested at different times of the day to verify the effect of APD adaptive gain control on the communication performance of the detection system. The flicker variance reaches its maximum at around 1: 00(PM) hours when the atmospheric turbulence is strong, and the average RBE of the system is 10-4 orders of magnitude when uncontrolled (Fig. 10). The experimental results show that the APD gain closed-loop regulation method can effectively reduce the RBE of the system and improve the communication quality. It can also increase the reliability of the laser communication performance under the atmospheric channel.
This study investigated the effect of atmospheric turbulence on laser communication systems and proposed a communication detection and reception method based on closed-loop regulation of the APD gain factor. Building a wireless laser communication experiment verifies that the APD gain closed-loop regulation method significantly improves the system performance under the turbulence fading channel. The above experiments show that the APD gain control can effectively suppress the optical power fluctuations generated by atmospheric turbulence jitter; thus, significantly improving the communication performance. Under weak turbulence conditions, the power spectrum curve with AGC is more consistent with the 5/3 power-law spectrum. The variance of light intensity flicker is reduced from 0.057 to 0.023; the system RBE is reduced from 1.75×10-8 to 3.99×10-10, a reduction of two orders of magnitude. Finally, the turbulence intensity and corresponding system average RBE variation curves under different periods are calculated. This shows that the adaptive gain control algorithm effectively suppresses turbulence-induced signal power fluctuations and achieves high interference immunity for wireless laser communication.
1 引言
以激光作为传输载体的无线激光通信技术结合了微波通信及光纤通信的优势,自由空间光通信(FSO)具有通信速率高、通信距离长、抗干扰性强、功耗低等优点,并且不需要铺设光纤线缆,已成为当前无线通信技术领域的研究热点[1-5]。对于无线自由空间激光通信,最严重的问题是大气信道的影响,其中大气湍流引起的光强闪烁效应使接收机接收到的通信数据叠加了抖动和衰落,这必然会导致通信质量下降。
近年来,针对大气湍流引起的光强闪烁现象,人们提出了众多解决方案,如Yuksel等[6-7]提出了一种孔径平均的方法,即利用多帧图像分析接收的强度闪烁模式以优化设计和配置自由空间光学通信链路。Lee等[8]研究了分集相干和非相干接收机在衰落、背景噪声等各种干扰条件下的性能并发现分集相干检测比分集直接检测的功率增益明显增多。Li等[9]阐述了采用正交阵列相移键控(QPSK)调制的海上大气相干自由空间光学的性能,并利用自适应光学(AO)技术补偿大气湍流。Zhang等[10]研究了一种基于低密度奇偶校验(LDPC)编码轨道角动量(OAM)的均匀圆形阵列自由空间光学系统在大气湍流衰落信道下的信道估计。尽管这些方法都在抑制大气湍流方面具有独特的作用,但这些方法都有较大的成本,如体积过于庞大、软硬件较为复杂、信息传输过程中容易损失数据等。
在大气湍流信道中,探测器探测后进行光电转化得出的电信号可直接表征光信号的强度起伏[11-12]。Yao等[12]提出了对自由空间链路上基于雪崩光电二极管(APD)探测器的多电平脉冲幅度调制(M-PAM)光信号传播模型的性能进行表征,表明通过增加APD的增益来降低APD的噪声是提高传输速率的有效方法,分析了在相同大气湍流条件下,APD增益越大对降低平均误码率(ABER)性能效果越明显,但并未对大气湍流闪烁效应提出改善方法。从通信的角度来讲,解决信号抖动衰落等问题最直接的方法是自动增益控制,通常采用的方法是放大器自动增益控制(AMP-AGC),即在后端放大器电路上进行自动增益控制。但是这种方法抑制抖动和衰落的能力十分有限,会受到前端放大器的限制,且动态范围没有明显改变,因此本文采用的方法是对前端雪崩光电二极管进行自动增益控制(APD-AGC),该项技术是更改反偏电压以控制APD的倍增因子,总倍增因子范围可从2倍调整至20倍,因此从理论上来讲,动态范围可扩大3~13 dB,对抑制大气湍流和扩大动态范围起到了重要作用。
本文介绍了APD-AGC技术,对该技术建立了理论模型,设计了APD电流反馈和偏置控制电路,编制一套比例、积分微分(PID)闭环调节算法,并在湍流信道条件下开展了距离为500 m的弱湍流抑制FSO实验。
2 大气湍流对空间激光通信影响机理分析
大气湍流是由地球表面和大气之间的温度差所引起的,在这种温度梯度存在的情况下,吹过地球表面空气动力学粗糙区域的风会造成大气折射率的随机波动,从而形成光学湍流。通过大气湍流传播所接收到的光强起伏称作“闪烁”。当湍流经过半径远大于湍流自身的光束时,许多尺寸不一的涡旋会对经过其中的光束进行散射、衍射和反射等,多光束相互干涉从而造成光强在时间及空间内随机起伏,这种现象称为光强闪烁。
闪烁指数(
光波传播的理论研究传统上分为弱涨落理论和强涨落理论。当使用Kolmogorov谱研究沿长度为L的路径传播的平面波或球面波时,通常用Rytov方差(
光强闪烁会造成接收机的功率损失和起伏,并最终导致接收信号衰减至低于可检测阈值。在这样的环境下,可以从概率密度函数(PDF)的数学模型推导出随机光强衰落信号。
弱湍流情况下的光强概率密度函数pI(I)服从对数正态分布[15]
由于大气湍流具有一定的不确定性,采用随机场理论对其进行分析。对于时间的确定且可积函数f(t)来说,该时间函数的频率可由傅里叶变换表示
而对于复杂平稳随机过程来说,可以用黎曼积分来进行表述[16]
大气湍流是随时间和空间不断变化的,因此时间和空间统计之间的关系是大气测量的中心问题。同时,大气湍流具有各向同性及各态历经性,结合泰勒“冰冻”假说的相关内容,通过观测方向上的平均风速,将空间统计数据转换为时间统计数据,根据Ishimaru[16]和Tatarski[17]的理论,功率谱密度SI(ω)可以由时间协方差函数的傅里叶变换定义
在此,将Kolmogorov的结构函数理论推广到统计均匀的、各向同性的温度波动,得到了与纵向速度波动相同的幂律关系[14],即
Kolmogorov通过量次分析表明,折射率的结构函数在惯性副区遵从5/3次幂律,惯性副区内的折射率波动的三维功率谱Φn(κ)可以由5/3次幂律表示
大气湍流引起的波动会直接影响探测器的信噪比参数,从而影响误码率(BER, RBE)[17]。定义OOK激光通信系统的误码率关系为
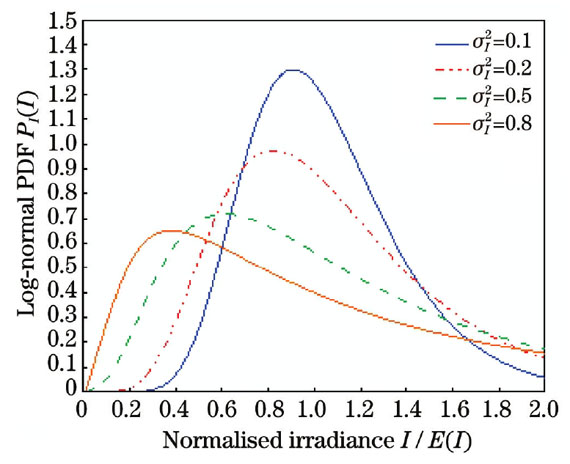
图 1. 不同闪烁方差下的光强概率密度分布曲线
Fig. 1. Light intensity probability density distribution under different optical intensity flicker
在此仅考虑大气湍流影响下的信噪比方程,以建立闪烁方差及误码率的关系式,信噪比的表达式如下
3 自适应乘法APD接收机方案
在明确大气湍流对通信质量的影响之后,考虑APD增益控制补偿的原理。APD乘法闭环调节算法符合雪崩光电二极管的乘法特性。根据APD检测理论[19],APD的响应方程为
M可表述为
在此基础上,实验设计了APD增益控制接收机,接收机的硬件如
控制算法流程图如
4 实验与结果
为了验证上述方法,设计如下实验,自由空间光通信系统普遍采用强度调制(IM)/直接探测(DD)的方式,假定通信系统仅受到大气信道湍流的影响,实验总体框图如下:
IM/DD通信模型从结构上可以分为发射端和接收端两部分。发射端待传数据经调制后加载在激光器上,通过光学天线整形后以平行光发射,经过大气信道到达接收端,由光学天线将信号光汇聚在APD靶面上,完成光信号到电信号的转换,而后通过信号处理得到数据的恢复。
在上述理论和实践的基础上,进行了500 m弱湍流情况下等效激光通信验证实验,旨在验证在弱湍流情况下,通过APD增益闭环控制抑制湍流引起的光强抖动。
在广东省东莞市华为松山湖基地进行通信距离为500 m的激光通信试验,实验当日情况为平均气温25 ℃,天气状况为多云微风。实验系统由APD接收端和LD发射端组成,测试现场如
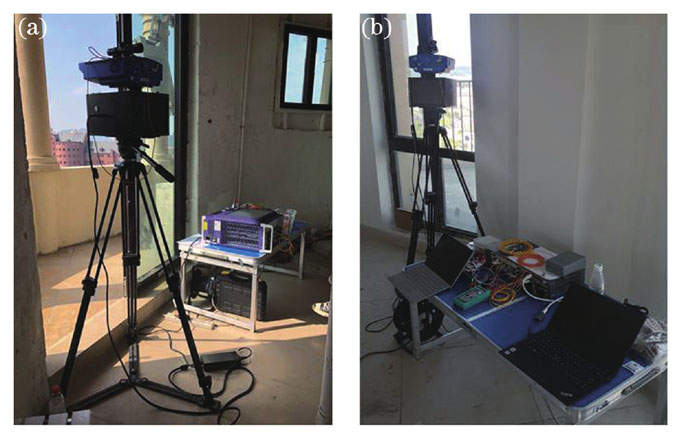
图 5. 实验现场图。(a)A端实验现场图;(b)B端实验现场图
Fig. 5. Experimental site map. (a) A side of experimental site map; (b) B side of experimental site map

图 6. 弱湍流条件下接收光信号强度图。(a)无增益控制;(b)有增益控制
Fig. 6. Received light signal intensity map for medium turbulence conditions. (a)Without control; (b)with control
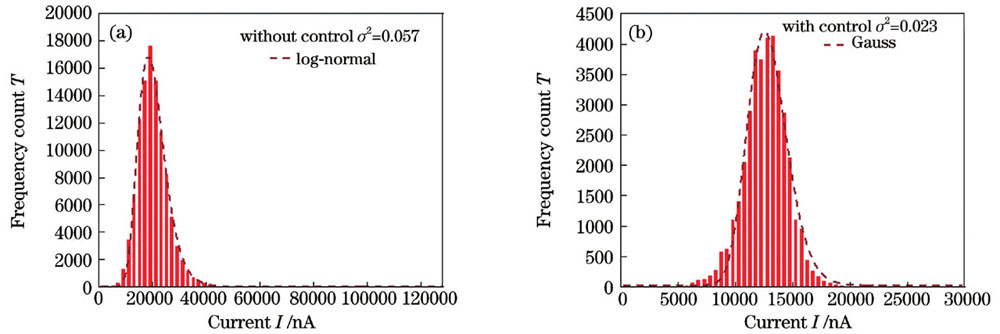
图 7. 500 m无、有增益控制的概率分布图。(a)无增益控制;(b)有增益控制
Fig. 7. Probability distribution for 500 m without and with gain control. (a)Without control; (b)with control
从

图 9. 500 m通信实验误码率测试(BERT)图。(a)无增益控制;(b)有增益控制
Fig. 9. BERT charts for 500 m communication experiments. (a) Without control; (b) with control
为进一步验证APD自适应增益控制对探测系统的通信性能改善效果,对一天中不同时间段即11时至17时的闪烁方差及误码率进行测试。采用相同的测试模式,分别在开启自适应增益控制及未开启自适应增益控制的情况下采集APD探测到的电流值,而后统计不同时刻的闪烁方差,结果如
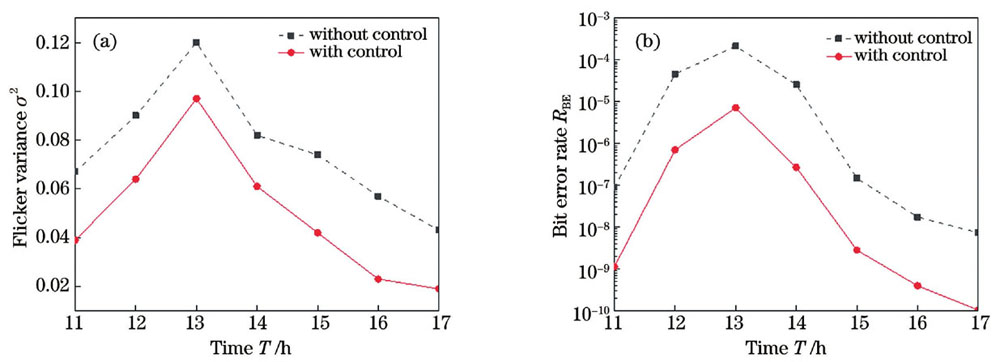
图 10. 不同时段测试图。(a)闪烁方差测试图;(b)误码率测试图
Fig. 10. Test plots for different time periods. (a) Flicker variance test plots; (b) BER test plots
从
5 结论
本文研究了大气湍流对激光通信系统的影响,提出了基于APD增益因子闭环调控的通信探测接收方法。通过搭建无线激光通信实验,验证了APD增益闭环调控方法在湍流的衰落信道下,对系统性能有明显的改善作用。以上实验表明,APD的增益控制可以有效抑制大气湍流抖动产生的光功率波动,从而显著提高通信性能。在弱湍流条件下,开启自动增益控制的功率谱曲线更符合5/3次幂律谱,光强闪烁方差由0.057降低至0.023,系统误码率由1.75×10-8降为3.99×10-10,降低了两个数量级,并统计不同时间段下的湍流强度及对应的系统平均误码率变化曲线。结果表明了自适应增益控制算法有效地抑制了湍流引起的信号功率波动,实现了高抗干扰能力的无线激光通信。
[1] 韩立强, 游雅晖. 大气衰减和大气湍流效应下多输入多输出自由空间光通信的性能[J]. 中国激光, 2016, 43(7): 0706004.
[2] 姜会林,佟首峰,张立中. 空间激光通信技术与系统[M]. 北京:国防工业出版社,2010: 50-113.
JiangH L, TongS F, ZhangL Z. The technologies and systems of space laser communication technologies and systems of space laser communication[M]. Beijing: National Defense Industry Press, 2010: 50-113.
[3] 曹明华, 武鑫, 王惠琴, 等. Gamma-Gamma大气湍流下超奈奎斯特光通信系统性能[J]. 中国激光, 2020, 47(9): 0906003.
[4] 李晓燕, 张鹏, 佟首峰. 大气湍流影响下基于自适应判决门限的逆向调制自由空间光通信系统误码率性能分析[J]. 中国激光, 2018, 45(6): 0606001.
[5] 曹阳, 李岳, 李小红. 无线光通信中极化码构造方法研究[J]. 光学学报, 2020, 40(21): 2106003.
[6] Yuksel H, Davis C C. Aperture averaging for studies of atmospheric turbulence and optimization of free space optical communication links[J]. Proceedings of SPIE, 2005, 5892: 58920P.
[7] Yuksel H, Milner S, Davis C C. Aperture averaging for optimizing receiver design and system performance on free-space optical communication links[J]. Journal of Optical Networking, 2005, 4(8): 462-475.
[8] Lee E J, Chan V W S. Diversity coherent and incoherent receivers for free-space optical communication in the presence and absence of interference[J]. Journal of Optical Communications and Networking, 2009, 1(5): 463-483.
[9] Li M, Cvijetic M. Coherent free space optics communications over the maritime atmosphere with use of adaptive optics for beam wavefront correction[J]. Applied Optics, 2015, 54(6): 1453-1462.
[10] Zhang Y, Wang P, Liu T, et al. Performance analysis of a LDPC coded OAM-based UCA FSO system exploring linear equalization with channel estimation over atmospheric turbulence[J]. Optics Express, 2018, 26(17): 22182-22196.
[11] Yao H F, Chen C Y, Ni X L, et al. Analysis and evaluation of the performance between reciprocity and time delay in the atmospheric turbulence channel[J]. Optics Express, 2019, 27(18): 25000-25011.
[12] Yao H F, Ni X L, Chen C Y, et al. Performance of M-PAM FSO communication systems in atmospheric turbulence based on APD detector[J]. Optics Express, 2018, 26(18): 23819-23830.
[13] Yaglom A M, Newell G. An introduction to the theory of stationary random functions[J]. Journal of Applied Mechanics, 1963, 30(3): 479.
[14] 易湘. 大气激光通信中光强闪烁及其抑制技术的研究[D]. 西安:西安电子科技大学,2013: 23-27.
YiX. Research on irradiance scintillation and mitigation technology in atmospheric laser communications[D]. Xi’an: Xidian University, 2013: 23-27.
[15] Dang N T, Pham A T. Performance improvement of FSO/CDMA systems over dispersive turbulence channel using multi-wavelength PPM signaling[J]. Optics Express, 2012, 20(24): 26786-26797.
[16] Ishimaru A. Wave propagation and scattering in random media[J]. Wave Propagation & Scattering in Random Media, 1978, 1(6): 407-460.
[17] TatarskiV I. Wave propagation in a turbulent medium[M]. New York: Academic Press, 1961: 338-366.
[18] Li Y, Li M, Poo Y, et al. Performance analysis of OOK, BPSK, QPSK modulation schemes in uplink of ground-to-satellite laser communication system under atmospheric fluctuation[J]. Optics Communications, 2014, 317: 57-61.
[19] YarivA, YehP. Photonics: optical electronics in modern communications[M]. New York: Oxford University Press, 2006: 529-530.
Article Outline
周畅, 于笑楠, 姜会林, 王潼, 安宁. 基于APD自适应增益控制的近地无线激光通信信道大气湍流抑制方法研究[J]. 中国激光, 2022, 49(4): 0406002. Chang Zhou, Xiaonan Yu, Huilin Jiang, Tong Wang, Ning An. Atmospheric Turbulence Suppression Methods for Near the Earth Wireless Laser Communication Channels Based on Avalanche Photodiode Adaptive Gain Control[J]. Chinese Journal of Lasers, 2022, 49(4): 0406002.