单粒子拉曼光谱分析聚苯乙烯微球的老化机理
下载: 1223次
Microplastics are new environmental pollutants of great concern. Due to ultraviolet (UV) radiation, biodegradation or mechanical abrasion in the natural environment, the properties such as size, surface morphology, and composition structure change, and microplastics decompose into smaller micro-plastics. However, up to date, the knowledge of the interaction between micron-sized microplastics and UV is still lacking, and the further decomposition of microplastics into smaller debris increases the surface area and makes it easier to adsorb toxic substances. Smaller microplastics are also more easily absorbed by fish and further into the top of the food chain, with a greater potential impact on humans. Polystyrene (PS) is a polymer synthesized from styrene monomers and used in a wide range of applications in industrial production and daily life. Abandoned PS waste and debris in the environment are common. In this paper, the aging process of micron-sized PS plastics is monitored by simulating the UV irradiation of PS microspheres. Single-particle Raman spectroscopy is used to detect the molecular changes after UV treatments, and scanning electron microscopy (SEM) is used to observe the changes in the surfaces and sizes of the particles in order to understand the effect of natural factors on small PS particles and gain the knowledge of the interaction between tiny microplastics and UV irradiation.
The aging process of micron-sized PS is simulated by exposing the particles to UV irradiation. 1, 2, 3 and 5 μm PS beads are evenly spread on sterile slides and air-dried naturally. The slides are then placed under a UV lamp at a distance of 20 cm from the UV lamp (the intensity is measured to be ~ 2.5 μW/cm2). After 1, 2, 3, 4 or 5 d of irradiation, the slides are removed and PS particles are re-suspended in deionized water. The slides without radiation (i.e. 0 d) are used as controls.
Single-particle Raman spectroscopy is used to monitor the changes of individual PS particles before and after the aging treatment. A 780 nm laser beam with a power of about 30 mW is introduced into an inverted microscope and focused with a 100× oil-immersed objective (numerical aperture of 1.30) to form an optical trap for particle capture. The trapping laser also serves as the Raman excitation source, and the Raman scattering from the trapped particle is collected and focused onto a spectrometer, in which the Raman spectrum is recorded via a charge coupled device (CCD). Raman spectra are saved in an ASCII file. The spectra of individual particles are corrected using the calibration function. The background is first subtracted followed by 3-point adjacent smoothing, and then the spectra are imported into Origin and Matlab for the spectral component analysis and the principal component analysis, respectively. Scanning electron microscope is used to observe the surface textures and morphologies of the PS particles. The size is measured using the ImagJ software, and the 40 PS particles per treatment are measured and statistically analyzed.
The Raman signal intensity of PS decreases significantly with the increase of radiation time, especially for small size PS beads (Fig. 1). New bands form at 497 cm-1 and 1286 cm-1 for 1 μm PS and 392, 512, 526, 1367, 1511 cm-1 for 2 μm PS. With the extension of UV irradiation, the peaks at 620, 794, 1602 cm-1 are broadened (e.g. 1602 cm-1 peak became 15501700 cm-1 peak). The main peaks such as the benzene ring breathing peak at 1001 cm-1 are shifted or split into two adjacent peaks. Broad peaks between 460 cm-1 and 560 cm-1 or between 1220 cm-1 and 1320 cm-1 appear (Fig. 3). These changes indicate that the molecular structure of PS is significantly altered by UV irradiation.
SEM shows that the sizes and morphologies of PS particles also change after UV radiation (Figs. 58). Surface breakage and fragmentation are observed, and the particles change from round sphere shapes to less regular shapes. After a longer UV aging treatment, PS particles are deformed and the sizes of PS particles gradually decrease with increasing aging time. Especially, the smaller the particle size, the more serious the damage.
To further understand the aging behavior of microplastics in nature influenced by environmental factors, we investigate the aging mechanism of micron-sized PS particles at a single-particle level by simulating UV radiation. The spectral characteristics of PS particles under particle size and aging time are characterized using the single-particle Raman spectroscopy, and the principal component analysis further reveals the changes in the chemical structures of PS particles under UV exposure. UV radiation is found to damage the structures of PS polymers and PS molecules, and the small particle size, which is more significantly damaged, is degraded to a higher extent. These results suggest that the microplastics with different sizes exhibit different behavioral processes when facing with the same environmental factors, and that small size microplastics are more likely to be decomposed into small particles or small fragments that are potentially more threatening after UV radiation. This study provides new insights into the aging dynamics of microplastics in the environment from the single particle level.
1 引言
塑料因可塑性强、成本低且易于加工等特点,被广泛应用于工业、建筑、电子、医疗等领域 [1-3]。据统计,2018年全球就生产了3.2亿吨塑料,然而仅6%~26%的塑料可以被回收利用,其余的可能被焚烧、填埋或释放到环境中[2-3],在短期内无法分解,不仅对环境造成严重污染,而且对人类健康和生物群体都具有潜在危害[3-4]。同时,由于化学风化、机械侵蚀和生物降解的作用,大塑料碎片降解形成塑料碎片甚至微塑料,已经在环境中积累了几十年[5],在世界各地的空气、水体和沉积物中都可检测到,对环境构成了严重威胁[6]。
微塑料通常是指粒径小于5 mm的塑料碎片和颗粒。主要来源是人为制造的微小颗粒,如护理产品的生产、纺织品的制造等,以及工业泄漏或不正确处理造成的微塑料释放[3]。次要来源是塑料由于水解、光氧化、生物等作用而发生降解,进而形成较小的碎片[7],以及轮胎、合成草坪、油漆、农业塑料等。微塑料一旦释放到自然界中,可能会受到紫外线(UV)辐射、生物降解和机械磨损等作用,表面出现微裂纹,甚至化学结构发生改变,进而微塑料的尺寸、表面形貌、组成结构等特性发生改变[8-11]。
越来越多的研究关注微塑料的老化与分解问题[12-14],UV辐射是促成微塑料老化的一种基本方法,可引发碳碳主链聚合物降解,从而导致链断裂,形成的较小的聚合物片段[13]。当微塑料表面暴露在紫外线下时,会发生脆化,表面产生裂纹、破损[12-14];随着表面反应的进行,微塑料表面的裂缝和孔隙也会促进聚合物的进一步老化。研究表明,聚乙烯(PE)和聚丙烯(PP)薄膜暴露在太阳紫外线下,容易失去延伸性、机械完整性和强度,并且平均分子量降低[15-16]。
微塑料经过多种自然因素的作用,其尺寸、形貌和组成可能已经发生了很大的改变,甚至混杂或吸附有其他杂质,难以准确辨识。目前,已有多种不同的分析技术被用来表征微塑料,非破坏性的有焦平面阵列傅里叶变换红外光谱法(FPA-FT-IR)[17-18],拉曼显微光谱法(RM)[19-20]和扫描电镜(SEM)[5-6]等;破坏性的有热萃取解吸气相色谱质谱法(TED-GC-MS)[21-22]、裂解气相色谱法(Pyr-GC-MS)[23]等。其中,光镊拉曼光谱技术具有无损、灵敏度高、识别粒径小于1 μm的优点[24-26],有利于粒径在10 μm以下的微塑料的快速识别和表征[27-30]。
然而,关于粒径在10 μm以下的微塑料与紫外线之间相互作用的认识仍然缺乏,而且小尺寸的微塑料进一步分解为更小的微塑料,增加了比表面积,更易吸附大量有毒物质,也更易于被鱼类吸收,进而进入食物链的顶端,可能带来更大的潜在不利影响。聚苯乙烯(PS)是由苯乙烯单体经自由基加聚反应而合成的聚合物,在工业生产和日常生活中的应用非常广泛,遗弃在自然环境中的PS垃圾和碎片也很常见。因此,本文通过将PS微球暴露在紫外线下,分析了不同微米级粒径PS塑料的老化过程。采用光镊拉曼光谱技术检测了单个PS微粒老化处理前后的变化,并结合扫描电镜观察了相应颗粒的表面纹理和破损形态,分析了紫外辐射对PS微塑料的作用及PS塑料老化的行为。
2 材料与方法
2.1 材料
质量分数为2.5%的单分散聚苯乙烯微球来源于无锡瑞格生物科技有限责任公司,存放于4 ℃备用。紫外灯功率为11 W,波长为254 nm,来源于中山市古镇新宇宙电器照明有限公司。
2.2 紫外照射处理
取粒径为1,2,3,5 μm的PS微球各100 μL,分别加入到含有900 μL纯水的4个1.5 mL离心管中。振荡3 min使PS微珠悬浮均匀。然后,取出20 μL PS悬浮液并滴在无菌载玻片上,待涂层均匀,水分自然挥发完。每个样品准备6片,其中1片作为空白对照组,其余5片放置于超净台中,进行紫外线辐射处理。紫外灯距离样品的高度为20 cm并且光线均匀地照射到样品表面(经测算,紫外辐射强度约为2.5 μW/cm2,波长为254 nm)。紫外照射时间分别为1,2,3,4,5 d。取出并用铝箔包裹,以防止空气中粉尘污染和光的影响。
2.3 实验装置
单粒子拉曼光谱实验系统如文献[31]所述。780 nm二极管激光器发出的单束激光经滤波后被导入到倒置的显微镜上,经100× 物镜(数值孔径为1.30)聚焦形成单光束势阱,功率约为30 mW,具有足够强的作用力捕获PS颗粒。被捕获的粒子所激发的拉曼散射聚焦在光谱仪的入口狭缝处,并被电荷耦合器件图像传感器(CCD)探测。该系统在测样前先用粒径为2 μm的聚苯乙烯微球进行校正。
2.4 拉曼光谱的采集
取200 μL的纯水清洗处理过的PS微粒,将其转移至石英样品槽中并放置到倒置显微镜上。在100× 油浸物镜下随机捕获单个PS粒子,采集其拉曼信号,积分时间为5 s。每个处理采集40个微粒的拉曼光谱和同等条件下的5个背景。利用MATLAB软件对样品光谱进行背景扣除和三点平滑处理,处理后导入RamanBaseline软件中进行基线校正,然后导入Origin软件和MATLAB软件中分别进行光谱成分分析和主成分分析。
2.5 塑料表面特征的扫描电镜观察
利用扫描电子显微镜(SEM)进行形貌观察。将经过不同处理后的PS小球表面镀金,置于SEM下。由于UV照射后的塑料微球的表面纹理可能存在差异,对不同处理天数和不同粒径颗粒进行观察,并比较了未处理和老化处理后的PS微球的表面结构。使用ImagJ软件测量SEM图像中颗粒的尺寸,每个处理测量40个PS微粒并进行统计分析。
3 结果
3.1 PS颗粒的拉曼光谱分析

图 1. 不同粒径的PS微球经不同天数的紫外辐射处理后的平均拉曼光谱。(a)1 μm,插图为PS的分子结构;(b)2 μm;(c)3 μm;(d)5 μm
Fig. 1. Average Raman spectra of PS microspheres with different particle sizes treated by UV radiation after different days. (a) 1 μm, molecular structure of PS shown in inset; (b) 2 μm; (c) 3 μm; (d) 5 μm
与对照组相比,1,2,3 μm粒径微球在620,794,1602 cm-1波段处的光谱带都随老化处理时间的增加而逐渐变宽(
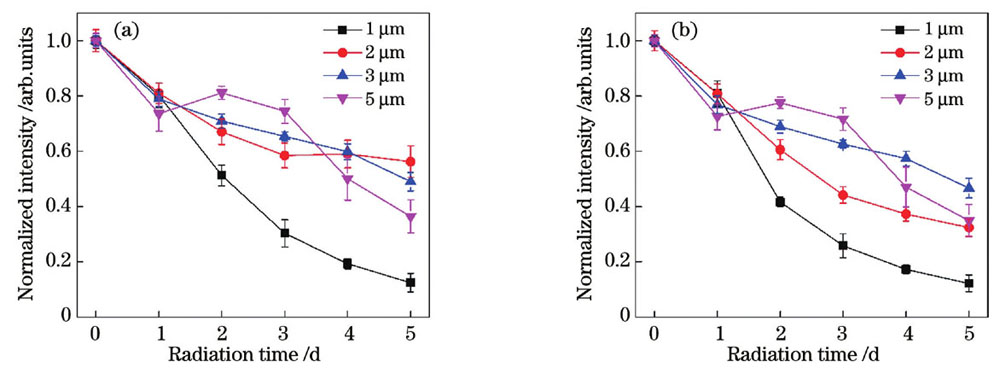
图 2. 不同粒径PS小球的峰信号强度随UV辐射时间的变化。(a)620 cm-1峰;(b)1001 cm-1 峰
Fig. 2. Peak signal intensities of PS microspheres with different particle sizes versus UV radiation time. (a) 620 cm-1 peak; (b) 1001 cm-1 peak
为了进一步了解UV老化处理前后PS颗粒组成的变化细节,将4种不同粒径的PS颗粒的拉曼光谱进行主成分分析(PCA),结果如

图 3. 不同粒径PS颗粒经0~5 d老化处理后的单粒子拉曼光谱的主成分分析。(a)(b)1 μm;(c)(d)2 μm;(e)(f)3 μm;(g)(h)5 μm
Fig. 3. Main component analysis of single particle Raman spectra for PS microspheres with different particle sizes after 05 d aging. (a)(b) 1 μm ; (c)(d) 2 μm; (e)(f) 3 μm; (g)(h) 5 μm
载荷分析显示,对于不同粒径PS微球,影响PC1分值的主要因素几乎相同,分别是620,794,1001,1030,1155,1183,1198,1602 cm-1等处的特征峰,它们反映了PS微球的拉曼光谱信号强度;随着UV处理时间的增加,拉曼信号强度减弱,PC1分值逐渐降低。因此,后续重点关注PC2和PC3的载荷(

图 4. 不同粒径PS微球经0~5 d老化处理后的PC2和PC3载荷分析。(a) 1 μm;(b) 2 μm;(c) 3 μm;(d) 5 μm
Fig. 4. PC2 and PC3 load analysis for PS microspheres with different particle sizes after 05 d aging. (a) 1 μm;(b) 2 μm; (c) 3 μm; (d) 5 μm
在1 μm粒径微球中[
在2 μm粒径PS颗粒中[
而在3 μm和5 μm粒径的微球中[
由此可见,影响PC1分值的主要因子是PS的主要特征峰及其强度的变化;影响PC2分值的主要因子是1001 cm-1峰在紫外线照射过程中分裂出来的995 cm-1和1007 cm-1峰以及620 cm-1峰分裂出来的614 cm-1和627 cm-1峰。但在1~2 μm粒径的微球中,出现一个460~560 cm-1宽峰。影响PC3分值的主要因子是794 cm-1峰向左偏移到798 cm-1及1220~1320 cm-1间的宽峰;更明显的是,2~5 μm粒径的微球中,1602 cm-1峰变宽大(1550~1700 cm-1)。
上述结果表明,PS微粒的拉曼光谱受老化时间的影响显著。峰值强度随老化时间的增加而减弱,特别是620,1001,1030,1183,1602 cm-1处的峰值强度在不同条件下都有所降低。主成分分析表明,经老化处理后,这些主峰发生了偏移或者分裂为两个相邻的峰,预示着分子结构发生了变化。
3.2 扫描电镜表征PS颗粒形貌与大小
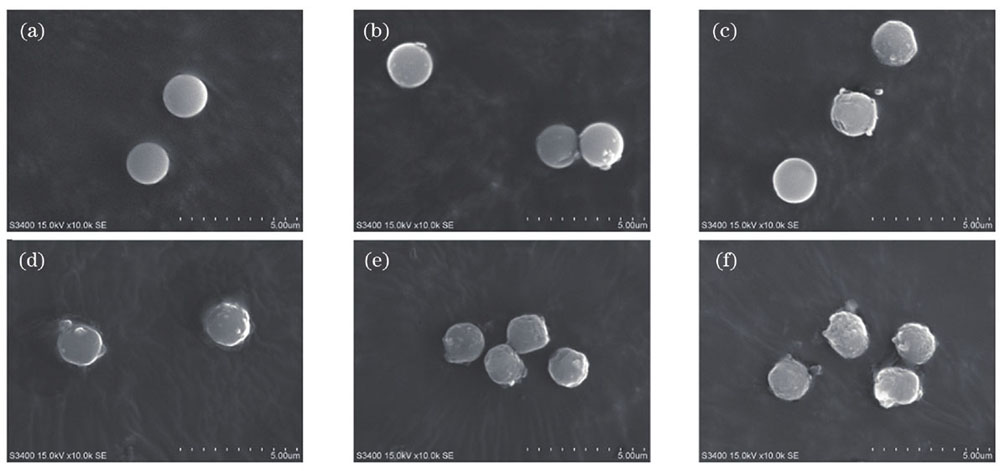
图 5. 2 μm粒径PS颗粒经不同天数的紫外处理后的表面形貌(标尺为5 μm)。(a)0;(b)1;(c)2;(d)3;(e)4;(f)5
Fig. 5. Surface morphologies of 2 μm PS particles after UV treatment for different processing days (scale is 5 μm). (a) 0; (b) 1; (c) 2; (d) 3; (e) 4; (f) 5

图 6. 3 μm粒径PS颗粒经不同天数的紫外处理后的表面形貌(标尺为5 μm)。(a)0;(b)1;(c)2;(d)3;(e)4;(f)5
Fig. 6. Surface morphologies of 3 μm PS particles after UV treatment for different processing days (scale is 5 μm). (a) 0; (b) 1; (c) 2; (d) 3; (e) 4; (f) 5
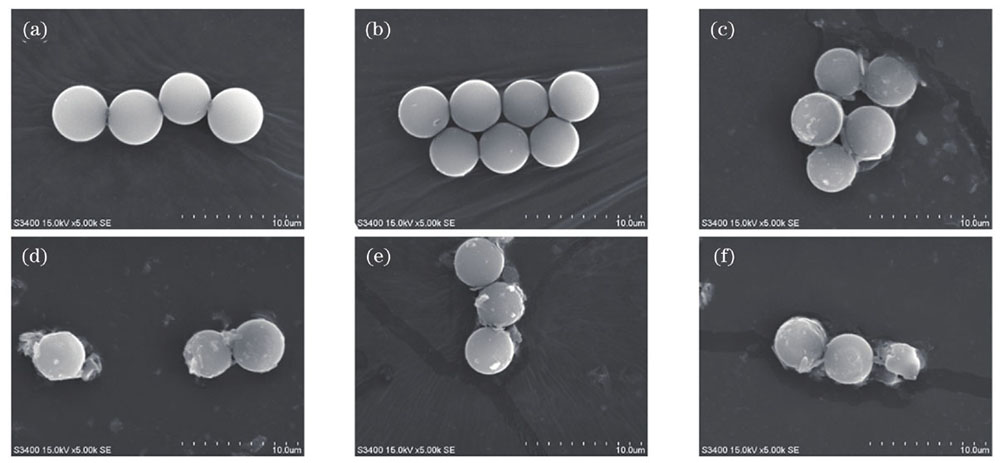
图 7. 5 μm粒径PS颗粒经紫外处理后的表面形貌(标尺为10 μm)。(a)0;(b)1;(c)2;(d)3;(e)4;(f)5
Fig. 7. Surface morphologies of 5 μm PS particles after UV treatment for different processing days (scale is 10 μm). (a) 0; (b) 1; (c) 2; (d) 3; (e) 4; (f) 5
另外,进一步统计了PS颗粒在不同照射时间下的大小(
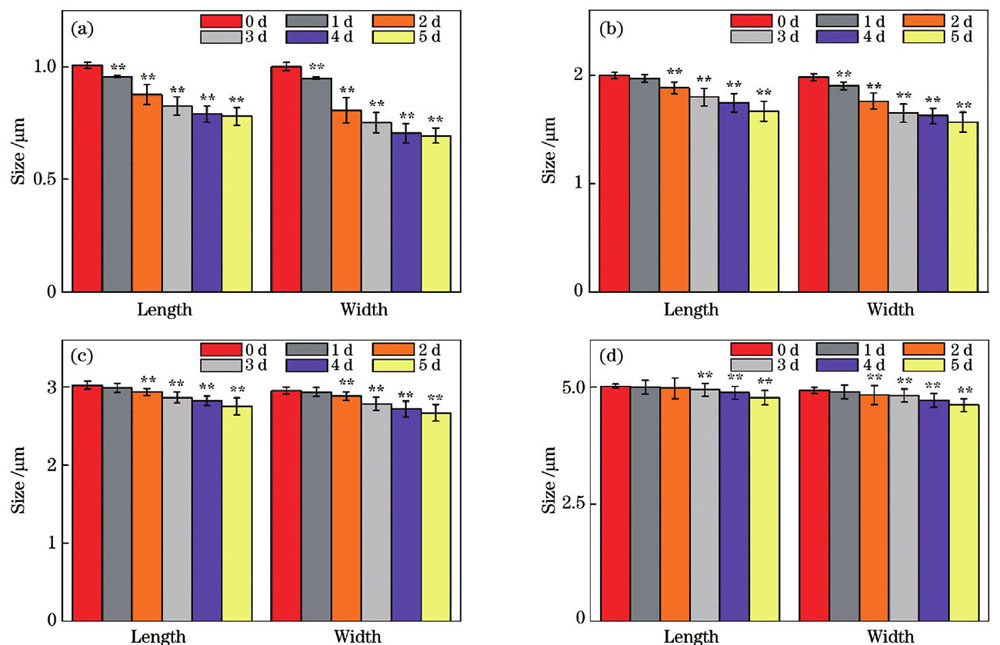
图 8. 经UV处理后不同粒径PS颗粒的粒径变化图(**表示对照组与处理组之间的差异极其显著)。(a)1 μm;(b)2 μm;(c)3 μm;(d)5 μm
Fig. 8. Particle size evolution of PS particles with different particle sizes after UV treatment (** indicates extremely significant difference between contrast and treatment groups. (a) 1 μm; (b) 2 μm; (c) 3 μm; (d) 5 μm
4 分析与讨论
PS是现代塑料工业中使用最广泛的合成聚合物之一,也是实验研究中最常用的聚合物。PS塑料在紫外线照射下,会快速变黄并逐渐脆化。老化作用对PS的化学和机械性能有很强的破坏作用。物理特征如粒径、形状、表面纹理等可以通过扫描电镜从视觉上区分,而化学特征如结构成分等可以通过拉曼光谱进行识别和表征。
PS塑料的芳环对紫外线有很强的吸收能力,并把能量转移到C—H基团,加速了PS的降解,经过照射后会形成更稳定的叔碳自由基[32]。拉曼光谱分析显示,PS微粒的总体信号强度随着辐射时间的增加而显著下降,可能原因是辐射作用破坏了聚合物的结构,由致密有序向松散状态改变,至少在颗粒的表面上是如此的,扫描电镜的结果也证明了这一点(
UV辐射破坏PS微球结构的证据还显示在拉曼光谱的细节中。结合PCA,发现1 μm粒径颗粒在497 cm-1和1286 cm-1处,2 μm粒径粒子在392,512,526,1367,1511 cm-1等处形成了新谱带;在620,794,1602 cm-1这些波段,随着紫外照射时间的延长,峰变宽大(如1602 cm-1峰变为1550~1700 cm-1峰),苯环呼吸峰1001 cm-1等发生了偏移或者分裂为两个相邻的峰,出现460~560 cm-1和1220~1320 cm-1宽峰。这些细节也表明,在紫外光照射下,聚苯乙烯微球的结构发生了明显的变化。
SEM形貌特征也显示,UV辐射后的PS颗粒大小和形貌也发生了改变。首先是辐射令球体表面出现轻微的裂纹,后发展为缺口,裂纹加深,明显破损,出现脆化和碎片化,最后严重破碎,甚至脱落损坏。因为表面破损和碎片脱落,颗粒由圆球体变为不规则的形状,尺寸变小。然而,在这种损伤过程中,粒径较小的PS颗粒的表面结构受到的影响较大。
实验结果表明,暴露在外界环境下的微塑料会在环境因素的影响下发生老化。其老化行为主要发生在微塑料表面,导致微塑料的组成结构和表面形貌发生变化,微塑料颗粒降解产生更微小的碎片,进一步给环境造成更严重的影响。因为更小的微纳米塑料具有更高的比表面积和更强的疏水性,极易吸附海洋中的重金属和持久性有机污染物,如农药、多氯联苯、双对氯苯基三氯乙烷(DDT)等,对生态环境造成严重的化学危害[33]。更小的塑料颗粒或碎片容易被生物吞食,在生物体内蓄积,危害生物安全;或作为载体,携带病毒、细菌以及危害微生物等,一旦进入人类的生物链,将对人类的健康带来潜在的威胁[34-35]。
5 结论
为了进一步理解微塑料在自然环境中受环境因素的影响而发生的老化行为,通过模拟紫外线对微塑料的照射,从单粒子角度对微米级粒径的PS颗粒的老化机理展开了研究。利用拉曼光谱表征了不同粒径和老化时间下的PS微粒的光谱特征,利用主成分分析进一步揭示了紫外线辐射下PS颗粒的化学结构的变化。发现UV辐射对PS多聚物的结构和PS分子的结构都产生了破坏,而且小粒径颗粒受到的破坏更明显,被降解的程度更高。这些结果表明,不同大小的微塑料在同样的环境下表现出不同的老化行为,而小粒径微塑料更容易受UV的影响而分解为潜在威胁更大的小颗粒或小碎片。
[1] Li J, Song Y, Cai Y B. Focus topics on microplastics in soil: analytical methods, occurrence, transport, and ecological risks[J]. Environmental Pollution, 2020, 257: 113570.
[2] Thompson R C, Moore C J, vom Saal F S, et al. Plastics, the environment and human health: current consensus and future trends[J]. Philosophical Transactions of the Royal Society of London. Series B, Biological Sciences, 2009, 364(1526): 2153-2166.
[3] Alimi O S, Farner Budarz J, Hernandez L M, et al. Microplastics and nanoplastics in aquatic environments: aggregation, deposition, and enhanced contaminant transport[J]. Environmental Science & Technology, 2018, 52(4): 1704-1724.
[4] Anderson J C, Park B J, Palace V P. Microplastics in aquatic environments:implications for Canadian ecosystems[J]. Environmental Pollution, 2016, 218: 269-280.
[5] van Cauwenberghe L, Vanreusel A, Mees J, et al. Microplastic pollution in deep-sea sediments[J]. Environmental Pollution, 2013, 182: 495-499.
[6] Zbyszewski M, Corcoran P L. Distribution and degradation of fresh water plastic particles along the beaches of lake Huron, Canada[J]. Water, Air, & Soil Pollution, 2011, 220(1/2/3/4): 365-372.
[7] Galafassi S, Nizzetto L, Volta P. Plastic sources: a survey across scientific and grey literature for their inventory and relative contribution to microplastics pollution in natural environments, with an emphasis on surface water[J]. Science of the Total Environment, 2019, 693: 133499.
[8] ter Halle A, Ladirat L, Martignac M, et al. To what extent are microplastics from the open ocean weathered?[J]. Environmental Pollution, 2017, 227: 167-174.
[9] Zhu K C, Jia H Z, Sun Y J, et al. Long-term phototransformation of microplastics under simulated sunlight irradiation in aquatic environments: roles of reactive oxygen species[J]. Water Research, 2020, 173: 115564.
[10] Corcoran P L, Biesinger M C, Grifi M. Plastics and beaches: a degrading relationship[J]. Marine Pollution Bulletin, 2009, 58(1): 80-84.
[11] Cai L Q, Wang J D, Peng J P, et al. Observation of the degradation of three types of plastic pellets exposed to UV irradiation in three different environments[J]. Science of the Total Environment, 2018, 628/629: 740-747.
[12] Singh B, Sharma N. Mechanistic implications of plastic degradation[J]. Polymer Degradation and Stability, 2008, 93(3): 561-584.
[13] Gewert B, Plassmann M M, MacLeod M. Pathways for degradation of plastic polymers floating in the marine environment[J]. Environmental Science: Processes & Impacts, 2015, 17(9): 1513-1521.
[14] Andrady A L. Microplastics in the marine environment[J]. Marine Pollution Bulletin, 2011, 62(8): 1596-1605.
[15] Obadal M, Čermák R, Raab M, et al. Study on photodegradation of injection-moulded β-polypropylenes[J]. Polymer Degradation and Stability, 2006, 91(3): 459-463.
[16] Hamid S H, Prichard W H. Mathematical modeling of weather-induced degradation of polymer properties[J]. Journal of Applied Polymer Science, 1991, 43(4): 651-678.
[17] Löder M G J, Kuczera M, Mintenig S, et al. Focal plane array detector-based micro-Fourier-transform infrared imaging for the analysis of microplastics in environmental samples[J]. Environmental Chemistry, 2015, 12(5): 563-581.
[18] Ivleva N P, Wiesheu A C, Niessner R. Microplastic in aquatic ecosystems[J]. Angewandte Chemie International Edition, 2017, 56(7): 1720-1739.
[19] Liu R, Taylor D S, Matthews D L, et al. Parallel analysis of individual biological cells using multifocal laser tweezers Raman spectroscopy[J]. Applied Spectroscopy, 2010, 64(11): 1308-1310.
[20] Redding B, Schwab M, Pan Y L. Raman spectroscopy of optically trapped single biological micro-particles[J]. Sensors, 2015, 15(8): 19021-19046.
[21] Eisentraut P, Dümichen E, Ruhl A S, et al. Two birds with one stone: fast and simultaneous analysis of microplastics: microparticles derived from thermoplastics and tire wear[J]. Environmental Science & Technology Letters, 2018, 5(10): 608-613.
[22] Duemichen E, Braun U, Senz R, et al. Assessment of a new method for the analysis of decomposition gases of polymers by a combining thermogravimetric solid-phase extraction and thermal desorption gas chromatography mass spectrometry[J]. Journal of Chromatography A, 2014, 1354: 117-128.
[23] Fischer M, Scholz-Böttcher B M. Simultaneous trace identification and quantification of common types of microplastics in environmental samples by pyrolysis-gas chromatography-mass spectrometry[J]. Environmental Science & Technology, 2017, 51(9): 5052-5060.
[24] 曹馨艺, 金尚忠, 侯彬, 等. 基于拉曼光谱的花粉检测及分类方法[J]. 激光与光电子学进展, 2020, 57(13): 133001.
[25] 何珊珊, 曹盼, 谭红琳, 等. 天然尖晶石和焰熔法合成尖晶石的光谱特征对比研究[J]. 激光与光电子学进展, 2021, 58(5): 0530001.
[26] 靳伟, 鲍海泓, 齐云, 等. 微纳结构光纤光谱学[J]. 光学学报, 2021, 41(1): 0130002.
[27] Anger P M, von der Esch E, Baumann T, et al. Raman microspectroscopy as a tool for microplastic particle analysis[J]. TrAC Trends in Analytical Chemistry, 2018, 109: 214-226.
[28] 刘铭晖, 董作人, 辛国锋, 等. 基于集成特征的拉曼光谱谱库匹配方法[J]. 中国激光, 2019, 46(1): 0111002.
[29] 季明强, 朱启兵, 黄敏, 等. 利用已知混合物拉曼光谱改善混合物成分识别精度的方法[J]. 中国激光, 2020, 47(11): 1111001.
[30] 王其, 曾万聃, 夏志平, 等. 基于随机森林算法的食源性致病菌拉曼光谱识别[J]. 中国激光, 2021, 48(3): 0311002.
[31] Zhang P F, Kong L B, Setlow P, et al. Multiple-trap laser tweezers Raman spectroscopy for simultaneous monitoring of the biological dynamics of multiple individual cells[J]. Optics Letters, 2010, 35(20): 3321-3323.
[32] Fernández-González V, Andrade-Garda J M, López-Mahía P, et al. Impact of weathering on the chemical identification of microplastics from usual packaging polymers in the marine environment[J]. Analytica Chimica Acta, 2021, 1142: 179-188.
[33] Mato Y, Isobe T, Takada H, et al. Plastic resin pellets as a transport medium for toxic chemicals in the marine environment[J]. Environmental Science & Technology, 2001, 35(2): 318-324.
[34] Viršek M K, Lovšin M N, Koren Š, et al. Microplastics as a vector for the transport of the bacterial fish pathogen species Aeromonas salmonicida[J]. Marine Pollution Bulletin, 2017, 125(1/2): 301-309.
[35] Revel M, Châtel A, Mouneyrac C. Micro(nano)plastics: a threat to human health?[J]. Current Opinion in Environmental Science & Health, 2018, 1: 17-23.
Article Outline
张秀娟, 李翠梅, 彭立新, 刘军贤, 王桂文. 单粒子拉曼光谱分析聚苯乙烯微球的老化机理[J]. 中国激光, 2022, 49(4): 0411002. Xiujuan Zhang, Cuimei Li, Lixin Peng, Junxian Liu, Guiwen Wang. Analysis of Aging Mechanism of Polystyrene Microspheres by Using Single Particle Raman Spectroscopy[J]. Chinese Journal of Lasers, 2022, 49(4): 0411002.