Highly transparent ceramics for the spectral range from 1.0 to 60.0 µm based on solid solutions of the system AgBr–AgI–TlI–TlBr
Download: 640次
1. Introduction
Currently, the mid-infrared (MIR) range is in demand for different fields of science and technology. However, it is poorly understood and developed, compared to the ultraviolet (UV), visible, and near-IR (to 1.0 µm) ranges[1
For two decades Ural Federal University has carried out research in the field of exploring and creating a new photonics’ element base for optical and laser instrumentation, crystals[10,11,25
The process of manufacturing ceramics is fast, because it takes place in two stages: the production of a high-purity raw materials and ceramics synthesis by the vertical method of directed crystallization. The technology is environmentally friendly and energy efficient; moreover, it is more cost effective in comparison with growing crystals, since it requires less material and time costs. Ceramics connect different crystal phases of two or more solid solutions of the AgBr–AgI–TlI–TlBr system. The boundaries of homogeneous and heterogeneous regions of solid solutions existence at low (298 K) temperatures were determined based on thermodynamic studies of AgBr–TlI and systems’ new phase diagrams in the temperature range from 298 K to 773 K and at a pressure of 101.325 kPa[25,29,30,41]. The new systems are quasi-binary cross sections of the AgBr–AgI–TlI–TlBr section of the Ag–Tl–Br–I concentration tetrahedron [Figs. 1(a) and 1(b)]. Figure 1(b) shows the composition areas where it is possible to synthesize ceramics, which is described below.
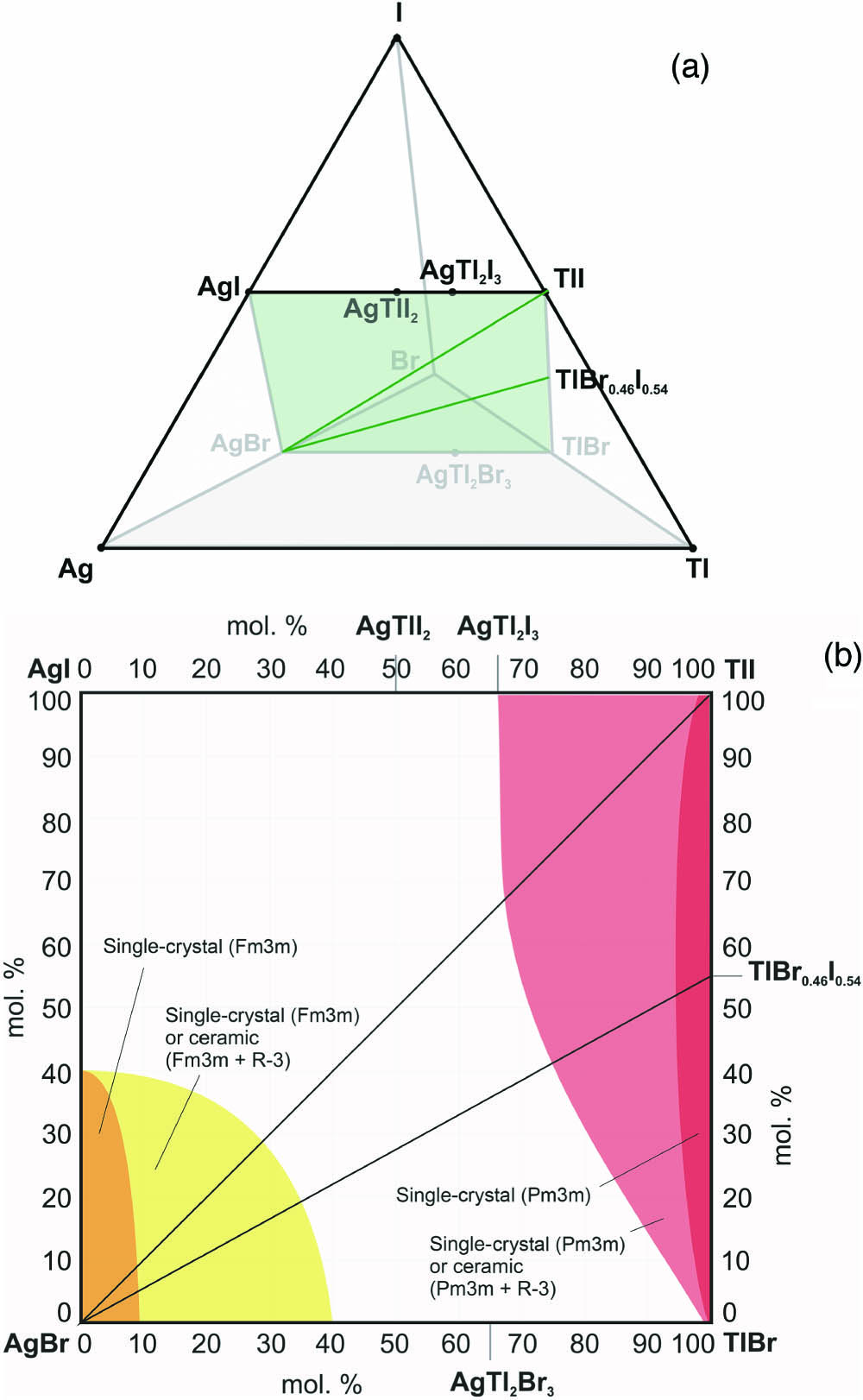
Fig. 1. (a) Concentration tetrahedron of Ag–Tl–Br–I and (b) isothermal cross section of AgBr–AgI–TlI–TlBr.
It should also be noted that the AgBr–AgI–TlI–TlBr materials system has a number of significant advantages over the AgCl-based system. It has increased material’s photo and radiation stability[26,30,31,36]. Thus, as a result of replacing lighter elements with heavier ones, the material’s stability to external negative environmental factors increases, which makes it possible to extend the life of optical products.
The technology of optical ceramics synthesis of AgBr–TlI and systems is eco-friendly, waste-free, energy- and resource-saving, and includes two stages: the production of a high-purity charge and ceramics synthesis by the vertical method of directed crystallization.
2. Materials and Methods
Ceramics synthesis at the first stage involves the production of high-purity raw materials based on solid solutions by a hydrochemical method, thermozone crystallization synthesis (TZCS)[11]. The TZCS method is the basis for the quality ceramics production and allows us to stably obtain high-purity raw materials (99.9999 wt.% and higher) with cationic impurities in the form of polycrystalline solid solutions with the specified composition[42]. The solubility and crystallization of monovalent thallium and silver halides in hydrogenated acid aqueous solutions in a wide temperature range have been studied for theoretical and practical justification of the TZCS process[43]. Supersaturation of the liquid phase is created and maintained by continuous circulation of this phase in a closed hardware chain, where it passes through zones with different temperatures: the saturation zone with a higher temperature and the crystallization zone with a lower temperature (Fig. 2). It is important that TZCS has been successfully implemented at the industrial level, where it has proven itself in all technological indicators as a simple and effective method. The TZCS method has a high yield of pure raw materials up to 97%–98%, is environmentally safe, and is energy-saving, since it does not require expensive equipment and provides the process organization with minimal waste in a closed cycle.
Using the TZCS method, the raw materials of the AgBr–TlI and systems were synthesized. The chemical and impurity composition of the raw materials and ceramics was determined by X-ray analysis and atomic emission spectroscopy with inductively coupled plasma. The total content of impurities, such as Mg, Al, Si, Cr, Mn, Fe, and others, was no more than 0.000035 wt.%. It is worth noting that the removal of water and oxygen-containing impurities from the raw material is carried out by combined cleaning, which includes the process of “leaking through” the molten charge in combination with directed crystallization from the melt.
The second stage of obtaining AgBr–TlI and systems heterophase ceramics is its synthesis by the vertical directed crystallization method from the melt. In order to do this, a raw material was loaded into an ampoule made of Pyrex glass, which had a hole in the lower part, and it was placed above the second ampoule. At a temperature of 470°C–480°C, the raw material melts, and then the process of leaking through the melt into the second ampoule occurs. After the leaking through process ends, the second ampoule is moved at a speed of 3–4 mm/h to the installation’s lower zone, which has a temperature of 250°C–260°C. In the conical part of the second ampoule, composite structures are crystallized based on cubic (Fm3m and Pm3m) and rhombic (R-3) phases of silver halides and monovalent thallium solid solutions. When the growing process ends, crystalline ceramics is obtained as a single-crystal sample with a cubic lattice and interspersed in the form of a rhombic phase. For all subsequent studies, including X-ray and spectral studies, we used plates with an optical surface. Small pieces were cut off from the grown sample, which were pressed into thin plane-parallel plates by hot embossing. Thus, property studies were already carried out on a polycrystalline material. Figure 3 shows a photo of the thin plane-parallel plates’ surface obtained with a ZEISS Crossbeam 550 electron-ion microscope. The figure shows two phases of different colors: the main dark gray phase is a solid solution with an Fm3m structure, and light gray inclusions are a solid solution with Pm3m structure.
X-ray diffraction (XRD) analysis was made by setup Rigaku MiniFlex 600, while the anode material was copper, the radiation type was , , the scanning range was from 3° to 90° with increments of 0.02°, and the scanning rate was 10° per minute. Samples are placed on a quartz glass substrate to achieve the required position. Obtained XRD patterns were processed using the Rigaku PDXL XRD analysis software.
The main optical materials’ characteristic is their transparency range. The spectral range study of the new ceramics was carried out on flat-parallel plates with a thickness of 300–1000 nm and made on a manual hydraulic press Specac 15T by the hot embossing technique[44]. The plates corresponded to different solid solutions’ compositions of the AgBr–TlI and systems. Determination of the ceramics spectral transmittance was performed using IR Fourier spectrometers IRPrestige-21 (Shimadzu), operating in the spectral range of (1.28–41.7 µm) with various combinations of detectors and dividers, and VERTEX 80 (Bruker) with an extended IR range of (14.7–60.6 µm). The card (ICSD ID 38723 or COD ID 1509393, trigonal) was taken as a basis for calculating the lattice parameters of the material with the R-3 structure.
3. Results
Figures 4 and 5 show XRD patterns for samples of AgBr–TlI and systems.

Fig. 4. XRD patterns for ceramic samples of (KRS-5) system: solid square, Fm3m; open diamond, R-3; open square, Fm3m.

Fig. 5. XRD patterns for ceramic samples of AgBr–TlI system: solid square, Fm3m; open diamond, R-3; open square, Fm3m.
For the AgBr–TlI system, two solid solutions represent the cubic phase of the ( and ) composition and the rhombic phase of the () composition. Similarly, in the system, solid solutions with cubic and rhombic phases have the following compositions: ( and ) and (). Thus, two-phase crystalline ceramics can be obtained in composition ranges, where both the Fm3m (or Pm3m) and R-3 phases exist. The presence of cubic and rhombic phases in both systems was confirmed by the X-ray analysis (Figs. 4 and 5). In order to confirm that all of the crystal phases are solid solutions, the dependence of the crystal lattice parameters on the composition for each of the phases was obtained. An example of the obtained dependences for the AgBr–TlI system is presented in Fig. 6. For the system, a similar picture was observed. The lattice parameters for the Fm3m phase linearly increase to in AgBr, after which it passes to other phases. At the same time, the lattice parameters of R-3 increase to . Then, the lattice is distorted with a decrease in the parameter after and the transition to other phases. There is a complex mixture of several rapidly changing phases in the concentration range of , which are not suitable for obtaining any kind of optics. Further, the R-3 phase appears again, and its lattice parameters increase to approximately 77 mol.%, after which a rearrangement occurs with a decrease in the lattice parameters, and the Pm3m phase appears in the system. Lattice parameters of the Pm3m phase increase linearly.

Fig. 6. Lattice parameters modification of Fm3m, Pm3m, R-3 phases depending on the composition.
The ceramics quality and the solid crystal phase ratio depend primarily on the homogenization degree of the initial components and to a lesser degree on the growth rate. A low homogenization degree (for example, with a simple mechanical mixing of finely divided AgBr and TlI raw materials), even at a low growth rate (), leads to the formation of the rhombic phase in a large proportion. It leads to the degradation in the material’s flexibility and complicates the manufacturing of optical products. With a high homogenization degree (TZCS method), even at a high growth rate (up to 10 mm/h), the rhombic phase proportion is less.
Figures 7 and 8 show the transmission spectra of composite ceramics based on two solid solutions of the AgBr–TlI and systems. Also, for comparison, the transmission spectrum of AgBr is shown in these figures. The transmission spectra demonstrate the displacement and expansion typical of most optical materials in the direction of the long-wavelength transmission limit. Such a shift occurs with an increase in the ions’ atomic mass, which makes up the optical materials’ structure. Substitution both in the cationic ( to ) and in the anionic sublattice ( to ) can significantly expand the spectral range. It was established that, depending on the composition, ceramics based on AgBr–TlI systems are transparent in the range from 0.497–0.568 µm to 45.0–67.0 µm, and ceramics based on system are transparent from 0.486–0.522 µm to 45.0–55.0 µm.
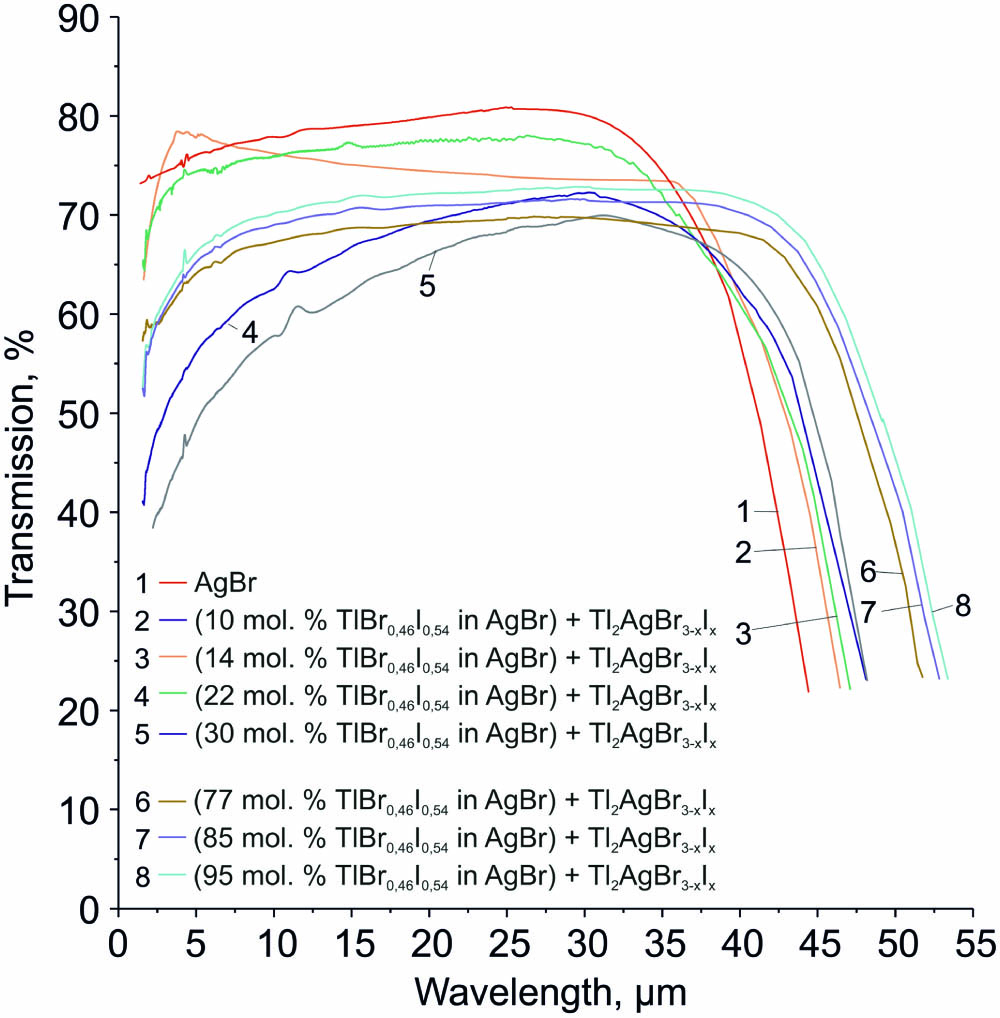
Fig. 8. Transmission spectra of multi-component heterophase ceramics based on two solid solutions of the system.
4. Conclusions
Based on the research findings, we can conclude that multi-phase crystalline ceramics based on several silver and thallium (I) halide solid solutions are promising for the manufacture of various optics and are not inferior to single crystals in their optical properties.
[1]
[2]
[4]
[6]
[13]
[14]
[17] A. S. Bubnova, V. I. Solomonov. Luminescence analysis of ceramic magnesium aluminum spinel Fe2+:MgAl2O4 synthesized from nanosized powders via syntering in air and vacuum. AIP Conf. Proc., 2019, 2174: 020087.
[18] V. V. Osipov, V. A. Shitov, R. N. Maksimov, K. E. Lukyashin, V. I. Solomonov, A. V. Ishchenko. Fabrication and characterization of highly transparent Fe2+:MgAl2O4 ceramics. Proc. SPIE, 2019, 11322: 113220M.
[20] E. V. Kolobkova, V. G. Melekhin, A. N. Penigin. Optical glass-ceramic based on fluorine-containing silicate glasses activated by rare-earth ions. Phys. Chem. Glasses., 2007, 33: 12.
[24]
[25] A. S. Korsakov, L. V. Zhukova, V. Korsakov, D. S. Vrublevskiy, D. D. Salimgareev. Research of phase equilibriums and modelling of structure of AgBr–TlBr0.46I0.54 system. Tsvetnye Metally, 2014, 8: 50.
[37] E. A. Korsakova, A. L’vov, I. Kashuba, V. Korsakov, D. Salimgareev, A. Korsakov, L. Zhukova. Fiber-optic assemblies based on polycrystalline lightguides for the mid-IR. J. Opt., 2019, 86: 439.
L. V. Zhukova, D. D. Salimgareev, A. E. Lvov, A. A. Yuzhakova, A. S. Korsakov, D. A. Belousov, K. V. Lipustin, V. M. Kondrashin. Highly transparent ceramics for the spectral range from 1.0 to 60.0 µm based on solid solutions of the system AgBr–AgI–TlI–TlBr[J]. Chinese Optics Letters, 2021, 19(2): 021602.