Photonics Research, 2019, 7 (9): 09001042, Published Online: Aug. 13, 2019
Simultaneous dual-contrast three-dimensional imaging in live cells via optical diffraction tomography and fluorescence
Download: 644次
Figures & Tables
Fig. 1. Comparison of the 2D and 3D diffracted field using Fourier relationship. (a) 3D light field of a cell, shown as different planes of wavefront information; (b) 2D light field of a cell extracted from (a); (c) 3D and (d) 2D Fourier spectrum of the light fields. Under Fourier operation, a “slice” of the diffracted field results in a 2D spectrum as shown in (d), whereas the entire volumetric diffracted field results in a 3D “spherical cap,” as shown in (c).
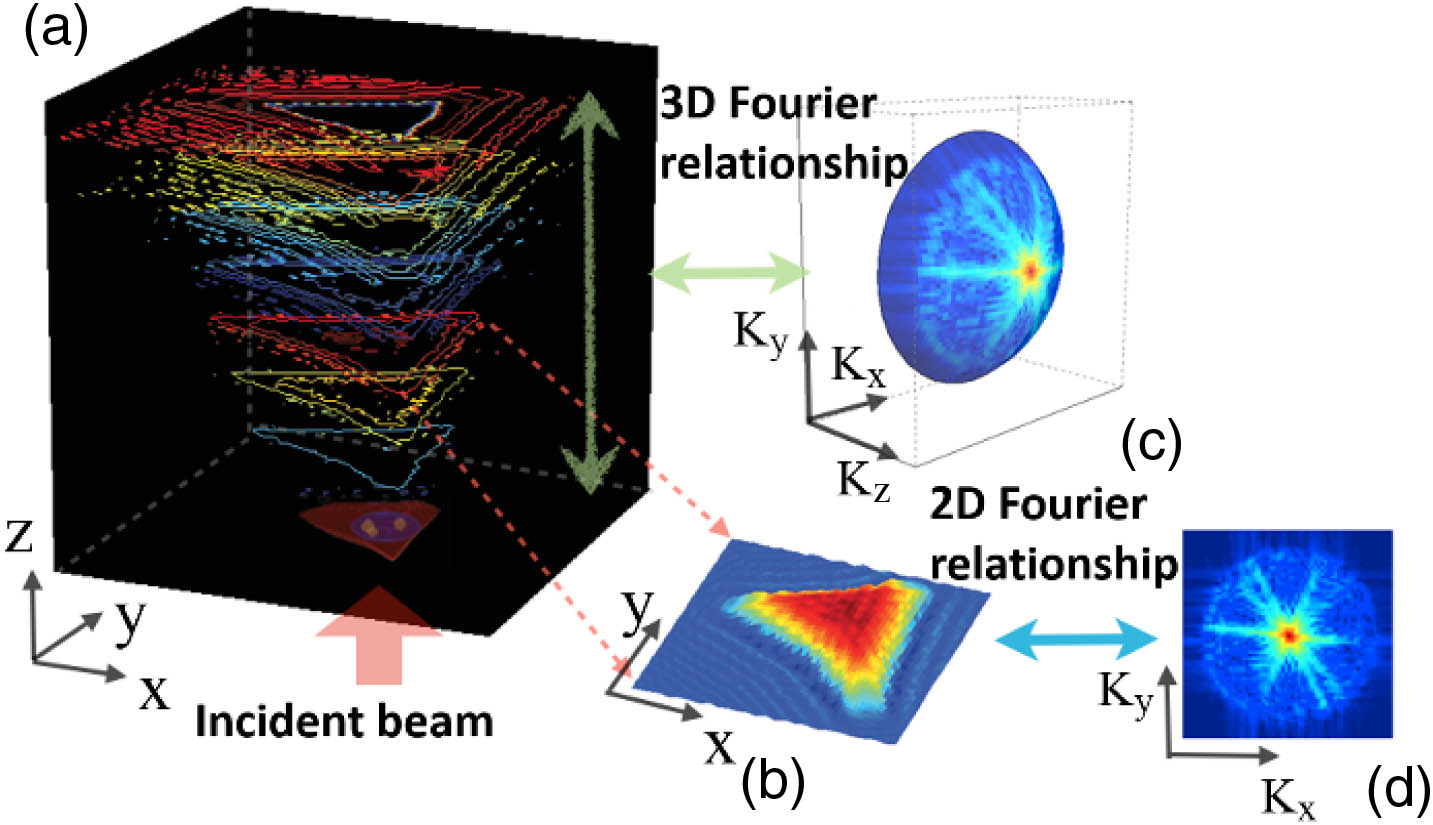
Fig. 2. Complex spectra synthesis of the scattering potential using three example angles. (a) A cell is subject to a multiangle plane-wave illumination with a maximum subtended half-angle θ ; hence, N.A. = sin θ . The 3D angular spectra under different incident angles can be acquired (only three angles shown). (b) 2D side view of the angular compensation method introduced during the synthesis of the 3D angular spectrum (only three angles shown). The green arc represents the center reference cap, the red and blue arcs represent the “compensated” caps from incident angles θ and − θ , respectively. Δ F z is the amount of shift in axial direction for the incident angle θ . (c) 3D view of a “synthesized” angular spectrum under three example angles. In (a), the center illumination and two maximum subtended angles illuminations formed three individual spherical caps in the Fourier domain as the 3D angular spectra. The respective 3D angular spectra are then translated and shifted accordingly, as shown in (b), so that angular information introduced in the incident beams is compensated. A synthesized angular spectrum for the three example angles is shown in (c), where all the 0th-order components coincide at a point.
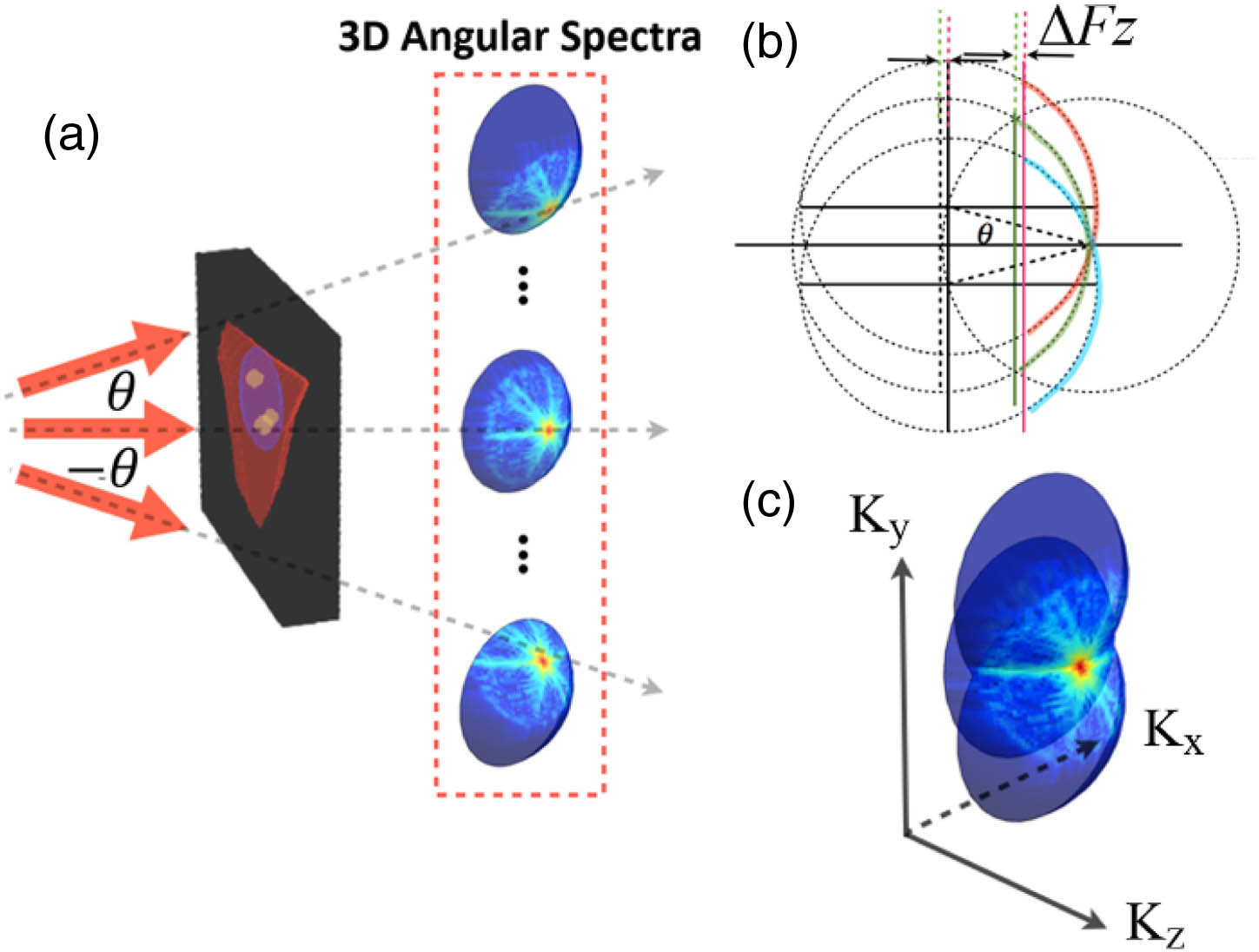
Fig. 3. Schematic diagram of the multimodal setup combining tomography with fluorescence. The tomography module is based on an off-axis digital holographic microscope setup in transmission. Different illumination angles are introduced by galvo mirror. The inset illustrates the tilted angle illumination situation. The fluorescence module is based on the epifluorescence microscopy. BS, beam splitter; GM, galvo mirror; SL, scanning lens; C, condenser; O, objective lens; TL, tube lens; Ex, excitation filter; Em, emission filter; DM, dichroic mirror.
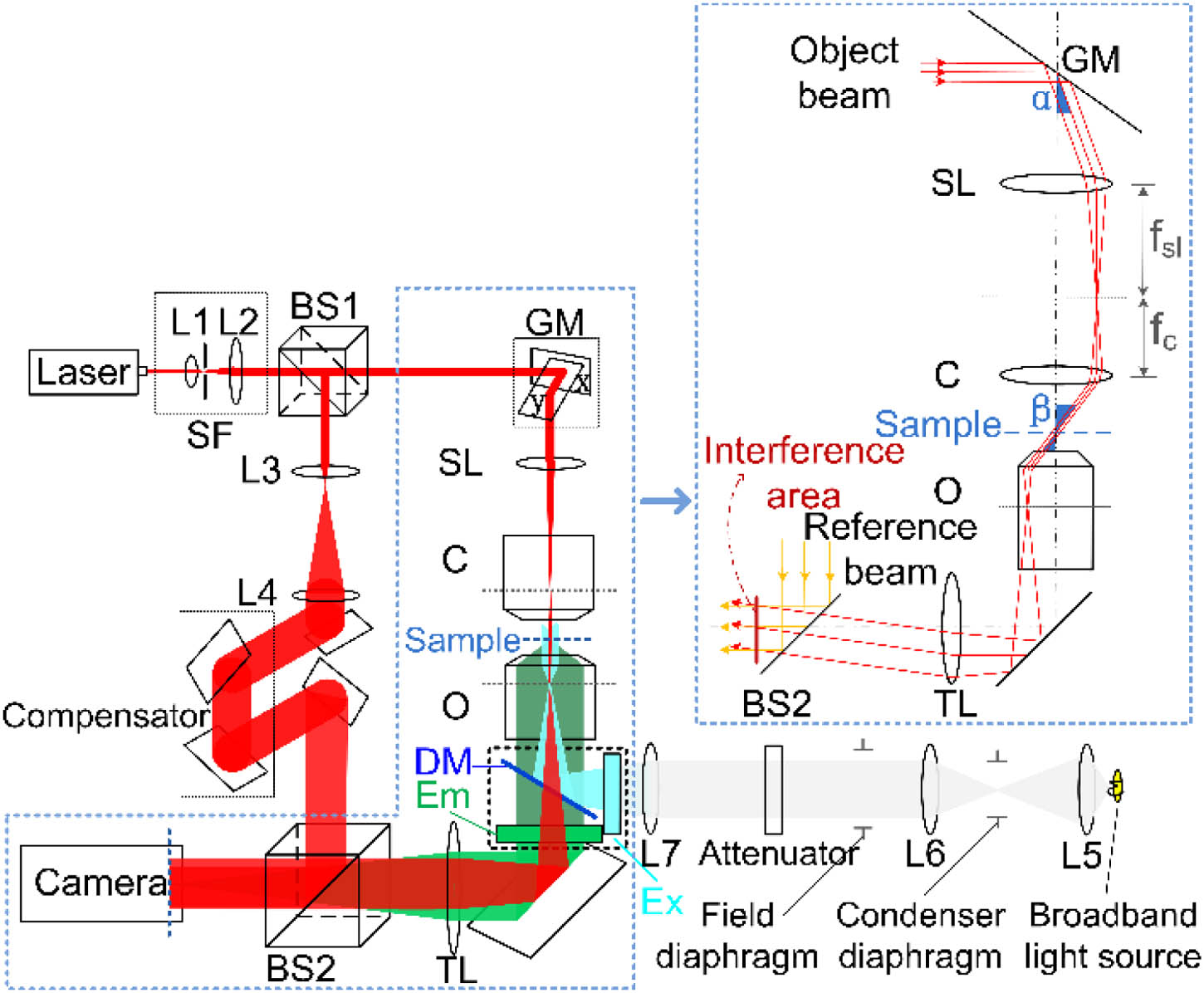
Fig. 4. Results for 3D reconstructions. (a) Buccal cell and (c) neuron, with internal structures delineated by groupings of refractive indices. The rotating views of the buccal cells and neuron can be found in Visualization 1 and Visualization 2 , respectively. Optical phase distribution of the same (b) buccal cell (in dashed box) and (d) neuron calculated from the interferometric measurements. The units for the color bars in (b) and (d) are radians. Part of the buccal cell in (a) is removed for clear visualization of the subcellular structures. The magnification is 20 × with an N.A. of 0.45.

Fig. 5. Structures reconstructed using RI spatial mappings in apoptotic T cells are shown to be compatible with wide-field fluorescence counterparts. (a) Cells with internal structures delineated from groupings of RI values (top view). Inset gives an opened-up view of the cell through the central z plane of the nucleus. The rotating view of the cells can be found in Visualization 3 . (b) 2D slice of RI distributions along x y plane; (c) wide-field fluorescence image of the same cells. The nuclear contents highlighted with blue contour lines in (b) are based on the intensity distributions of fluorescence in (c). The cell nuclei were stained with Hoechst dye. The value of RI in the range of 1.388–1.419 (pseudo-colored red orange) matches very well with what the fluorescent signal is showing, indicating that our technique is able to differentiate functional substructures within the cell. The magnification is 40 × with an N.A. of 0.6.

Fig. 6. Distributions of RI values (row 1) and fluorescence intensities (row 2) for Jurkat T cells under different conditions (scale bar, 10 μm). (a), (b) Viable cell; (c), (d) UV light-induced apoptotic cells with trovafloxacin treatment; (e), (f) necrotic cell. The positions of stained DNA, where many proteins are localized, are highlighted with blue contour lines in RI mappings based on fluorescence imaging. The magnification is 40 × with an N.A. of 0.6.
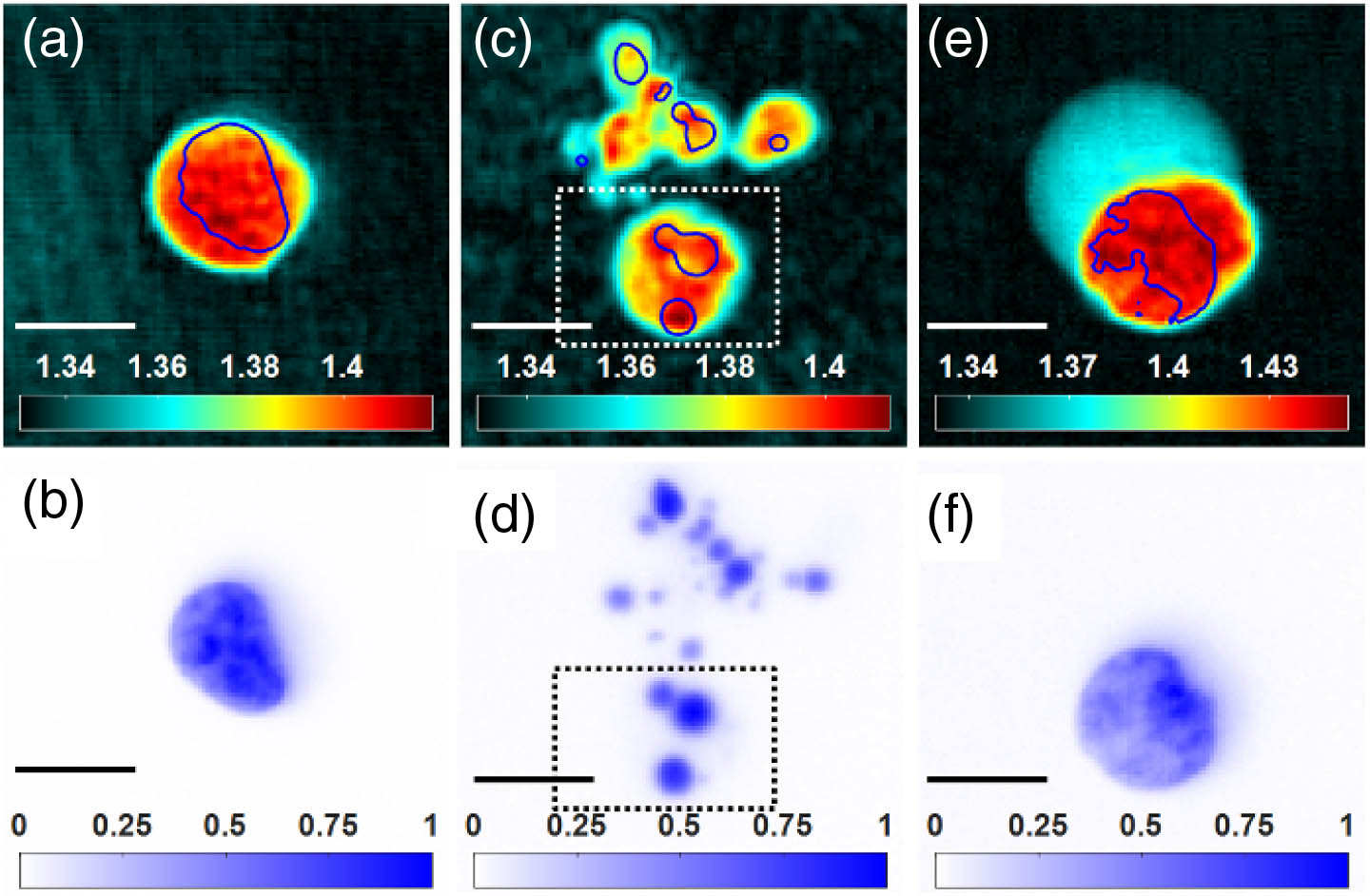
Fig. 7. Monte Carlo simulation of a phantom object volumetric diffraction and its quantitative reconstruction. (a) The configuration of the Monte Carlo simulation; (b) 3D view of the semispherical-shaped phantom object; (c) reconstructed RI distribution of the object in central y z plane; (d) cross section of the CTF (central f x f z plane), which is synthesized by 21 spherical Fourier caps.

Chen Liu, Michael Malek, Ivan Poon, Lanzhou Jiang, Arif M. Siddiquee, Colin J. R. Sheppard, Ann Roberts, Harry Quiney, Douguo Zhang, Xiaocong Yuan, Jiao Lin, Christian Depeursinge, Pierre Marquet, Shan Shan Kou. Simultaneous dual-contrast three-dimensional imaging in live cells via optical diffraction tomography and fluorescence[J]. Photonics Research, 2019, 7(9): 09001042.