激光光强波动无关的248 nm退偏器检测方法
下载: 567次
With the development of the semiconductor industry, the requirement for photolithography resolution is significantly increasing. As the resolution increases, the polarized illumination is required to improve the image contrast. Depolarizers are devices that convert incident-polarized light into unpolarized light, with the degree of polarization (DOP) of the output light as a core performance index. Depolarizer plays an essential role in the deep ultraviolet (DUV) lithography tool illumination system. Despite some reports on design, manufacture, and performance evaluation, most traditional detection methods work in the visible band. There is still no direct method for detecting the performance of the depolarizer for specific DUV wavelength applications. The key problem is that the high-level control requirement of the measurement error contradicts the insufficient performance of the light source and polarizing devices. To solve this problem, this study proposes a method for detecting 248 nm depolarizer independent of laser intensity fluctuation (Fig. 2 Schematic of the method, Fig. 7 Experimental equipment). The 248.372 nm excimer krypton fluoride (KrF) laser is used as the light source. The adverse effects caused by the laser intensity fluctuation are overcome through pre-polarizing and polarization beam splitting design. The measurement error is analyzed and verified experimentally. The DOP measurement value is highly consistent with the theoretical value, verifying the validity of the proposed method. The DOP results in the repeatability and reproducibility tests show very low standard deviations and variation coefficients, which are consistent with the analysis level. The proposed method provides a stable and effective tool for offline performance detection of the depolarizer used in the 248 nm lithography tool illumination system.
To detect the depolarizer used in 248 nm lithography, the light source needs to be monochromatic and high powered to eliminate dispersion and be effective under the conditions of a large beam area and a low transmittance of each device. Only the excimer KrF laser is suitable for the requirement. However, measured standard deviation of the emitted light intensity is 2.886% (Fig. 3); thus, the 3σ of 8.658%, even larger than the theoretical difference between the maximum and minimum light intensity in DOP measurement. For example, if DOP is 4%, the theoretical difference is only 8.333%. The valid information will be covered by random noise, making it difficult to obtain effective measurement results. We use pre-polarizing and polarization beam splitting designs to overcome this difficulty. The reference light and detection light synchronously reflect the light intensity fluctuation. Therefore, the fluctuation can be eliminated by the division operation. The pre-polarizing design can eliminate the interference of the polarization fluctuation of the light source on the polarization splitting ratio. Additionally, we quantitatively analyze six types of errors (Figs. 46) and distinguish the target and effect of each error, including their summary. We also conduct verification experiments and stability tests. The quarter-wave plate (QWP) can convert the incident linearly-polarized light into a circularly-polarized light, which has the same effect as a depolarized light in the detection. The actual retardation of QWP is not strictly a quarter wavelength but can be measured using an ellipsometer. The theoretical value of DOP is determined by retardation. Therefore, QWP can be used as a standard sample to verify the validity of the proposed method. Furthermore, repeatability and reproducibility measurements of the depolarizer sample can verify the stability of the proposed method.
The error analysis shows that the proposed method is highly suitable for performance detection of samples with small DOP, such as depolarizers. The absolute system, random, and total absolute errors are less than 0.1226%, ~0.2000%, and ~0.3226%. In the verification experiments, the mean retardation of the QWP measured using a VASE ellipsometer is 89.285° and the theoretical value of DOP is 1.2479%. The measured DOP using this device is 1.2902%, the standard deviation is 0.0458%, and the coefficient of variation is 3.5477% (Table 1). Compared with the theoretical values, the measurement system error is only 0.0423%, confirming the validity of the proposed method. In the repeatability test, the DOP of the depolarizer is measured with a mean, standard deviation, and coefficient of variation of 2.0701%, 0.0772%, and 3.7287%, respectively (Table 2). The measurement results show that the repeatability level of the device (3σ=0.2316%) is consistent with the analysis expectation (~0.2000%). In the reproducibility test, the DOP of the depolarizer is measured with a mean, standard deviation, and coefficient of variation of 2.1137%, 0.1030%, and 4.8730%, respectively (Table 3). The reproducibility level (3σ=0.3090%) is only slightly worse than the repeatability level, indicating the stability of the device.
This study proposes a detection method for the 248 nm depolarizer independent of laser intensity fluctuation. We selecte an excimer KrF laser with a stable output wavelength of 248.372 nm as the light source. The adverse effects caused by the light intensity fluctuation are overcome through pre-polarizing and polarization splitting design. The error analysis shows that the proposed method is suitable for performance detection of the depolarizer. The absolute system error is less than 0.1226%, and the random error is ~0.2000%. The verification experiments show that the measured mean DOP is highly consistent with the theoretical value. It also shows that the measurement system error is only 0.0423%, confirming the validity of the proposed method. The stable test shows the repeatability level (3σ=0.2316%) is consistent with the analysis expectation (~0.2000%), and the reproducibility level (3σ=0.3090%) is only slightly worse than the repeatability level, indicating the stability of the device. Therefore, the proposed method provides a stable and effective tool for offline performance detection of the depolarizer used in the 248 nm lithography tool illumination system. Furthermore, the method is essential and should be further developed to meet the requirements of shorter wavelength lithography systems.
1 引言
随着半导体产业的发展,人们对光刻分辨率的要求越来越高。为了提高分辨率,高端光刻工艺所采用的曝光波长已发展到深紫外(DUV)乃至极紫外(EUV)波段,深紫外光刻的数值孔径已经发展到0.5~1.35水平[1-3]。与此同时,离轴照明技术、相移掩模技术、光学邻近效应修正技术和光瞳滤波技术等分辨率增强技术也逐步得到了研究和广泛应用[4-9]。然而,随着分辨率的提高,照明光束的偏振态对最终的成像对比度起到了关键作用[10-14],最主要的原因是成像时存在多级次衍射光的干涉。不同级次S偏振光的振动方向一致,而P偏振光则不一致,从而使得这两个偏振分量在成像对比度上有很大差距。所以,各类偏振控制器件在高端光刻照明系统中必不可少。
退偏器是一类将入射偏振光转化为非偏振光的器件,其核心性能指标是出射光的偏振度(DOP)。其中,空间退偏器(更严格的表述是“伪”退偏器[15],通常称为“退偏器”)通过产生空间上渐变的偏振序列来达到平均退偏的效果,这与光刻照明系统的大光束尺寸适配。关于空间退偏器设计、制造、性能评估的研究已经有很多报道[16-23],但是,常见的偏振测量系统,如Hinds Instruments公司采用光弹调制法进行扫描偏振测量的Exicor系统[24]、Ilis公司采用改进塞纳蒙特法进行成像偏振测量的StrainMatic系统[25]以及相关研究中涉及的偏振测量系统[26-27],大多工作在可见光波段,目前尚缺乏直接对深紫外特定波长下应用的退偏器进行性能检测的方法,关键问题在于光源、偏振器件的性能不能满足高水平控制测量误差的需求。针对这一问题,本文提出并验证了一种248 nm退偏器检测方法,即:使用248.372 nm KrF(氟化氪)准分子激光器作为光源,利用预起偏和偏振分光设计克服激光强度波动造成的不利影响;最后对该方法进行了测量误差水平分析和实验验证。验证实验的DOP测量值与理论值高度一致,证明了该方法的有效性。重复实验结果与分析结果的误差水平相当,取得了非常低的标准差和变异系数,验证了该方法的稳定性。
2 原理
2.1 待测退偏器
如
2.2 检测方法
本文提出的248 nm退偏器检测方法如
该激光器的波长稳定性达到了亚纳米量级,显著减小了色散引起的测量误差,但检测中可能出现的激光光强波动会降低测量的精度和重复性,这对于退偏器这种出射DOP极小的器件的检测而言尤为不利。如
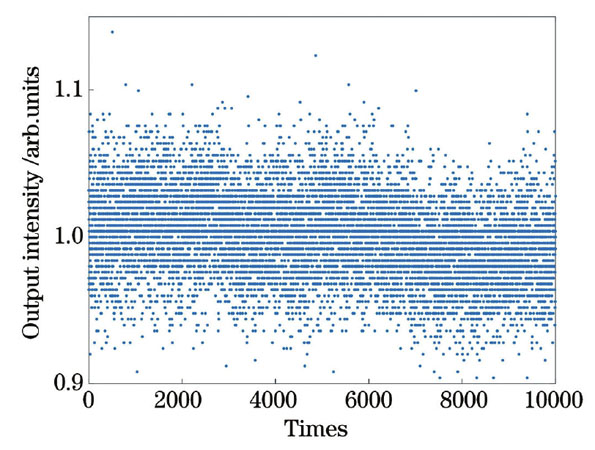
图 3. KrF准分子激光器出射光强的10000次重复测量结果
Fig. 3. 10000-repeated measurement results for output intensity of excimer KrF laser
装置工作时,旋转检偏器一周,同时记录参考光的光强和检偏后的光强,并进行比值处理,得到测量光强。通过拟合得到测量光强曲线,并取对应最大光强值Imax和最小光强值Imin,则DOP的测量值DP可以表示为
3 测量误差分析
在248.372 nm波长下,石英晶体的双折射率差Δn为0.01111,退偏器楔角α为0.834°,楔形波片中心厚度为1.374 mm,通光面直径D为25 mm。以入射光振动方向与退偏器光轴的夹角(=45°)为标准角度,以第二章的原理为依据,分析本装置的测量误差水平。
入射光的振动方向偏差以及光束尺寸的变化都会带来测量误差。由(5)式模拟计算的DOP相对于两者的关系曲线如
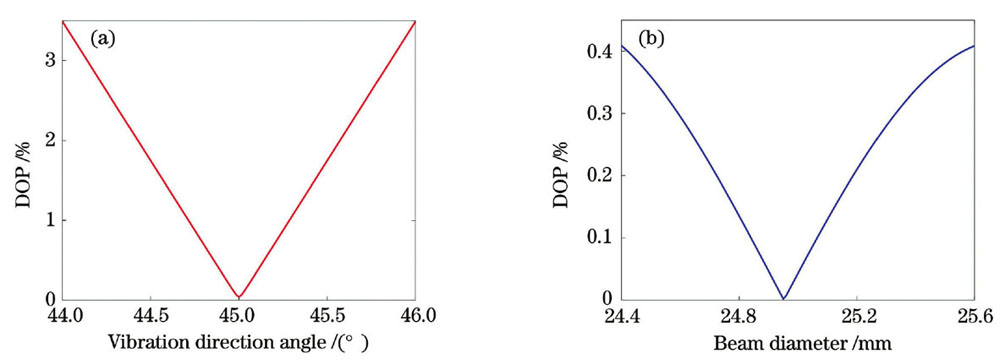
图 4. DOP与入射光振动方向角、光束尺寸的关系曲线。(a)DOP与入射光振动方向角的关系;(b)DOP与光束尺寸的关系
Fig. 4. DOP versus vibration direction angle and beam size of incident light. (a) DOP versus vibration direction angle of incident light; (b) DOP versus beam diameter
起偏器和检偏器的消光比也会带来测量误差。包含有起偏器消光比RPE1的DOP测量值DP1以及包含有检偏器消光比RPE2的DOP测量值DP2的计算公式为

图 5. DOP与起偏器、检偏器消光比的关系曲线。(a)DOP与起偏器消光比的关系;(b)不同DOP真实值下,DOP测量值和真实值的绝对误差与检偏器消光比的关系
Fig. 5. DOP versus extinction ratio of polarizer and analyser. (a) DOP versus polarizer extinction ratio; (b) absolute error between the measured and true DOP values versus analyser extinction ratio under different true DOP values
检偏器旋转精度和光功率计探头精度也是测量误差的来源。检偏器的旋转依赖于旋转步进电机,存在一个最小的步进角度,这会导致采集的光强最值处可能出现偏差。假设检偏器采集数据位置与实际最大、最小角度的偏差均为β,则根据马吕斯定律可得到DOP测量值DP3为
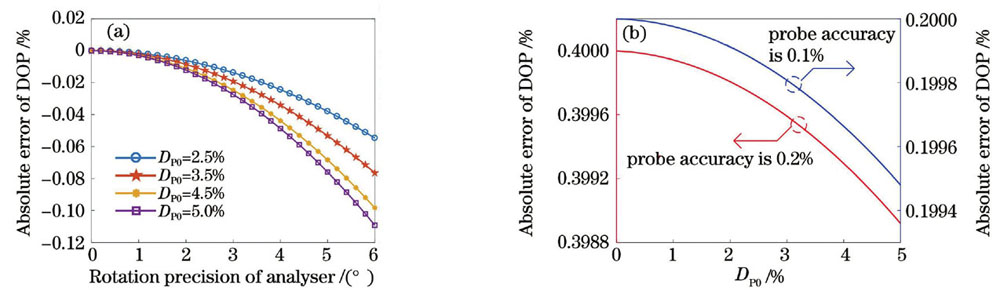
图 6. DOP测量值和真实值的绝对误差曲线。(a)不同真实值下绝对误差与检偏器旋转精度的关系;(b)不同探头精度下绝对误差与真实值的关系
Fig. 6. Absolute error curves of measured and true DOP values. (a) Absolute error versus rotation precision of analyser under different true values; (b) absolute error versus true DOP value under different probe accuracy values
接下来对上述讨论的所有误差进行综合分析。入射光束误差和起偏器误差会导致检测时出射光的DOP真值增加,检偏器的消光比和旋转随机误差会导致DOP测量值相对于真值降低,光功率计探头精度会引起随机误差。特别地,如
4 实验结果分析
248 nm退偏器检测实验装置如
使用四分之一波片作为验证实验的样品。理想情况下,四分之一波片具有将入射线偏振光转换为圆偏振光的能力(快轴和入射光振动方向呈45°时),而圆振偏光在本文检测方法下与退偏光具有相同的效果。实际的波片延迟量并不严格为四分之一波长(但可以由椭偏仪测定),因此DOP的理论值DPS为
表 1. 波片验证测试得到的DOP测量结果
Table 1. DOP measurement results obtained by wave-plate verification test
|
表 2. 退偏器DOP重复性测试结果
Table 2. DOP measurement results of depolarizer in repeatability test
|
表 3. 退偏器DOP再现性测试结果
Table 3. DOP measurement results of depolarizer in reproducibility test
|
使用本装置对一退偏器进行了性能检测,该退偏器的结构如2.1节所述。
5 结论
为了直接对应用于深紫外特定波长下的退偏器的性能进行检测评估,选用输出波长稳定的248.372 nm KrF准分子激光器作为光源来设计退偏检测方法,并利用预起偏和偏振分光设计克服了激光光强波动造成的测量难题。对该方法的测量误差进行量化分析,分析结果表明,该方法适用于退偏器性能检测,绝对系统误差小于0.1226%,随机误差约为0.2000%,总绝对误差约为0.3226%。
为了验证该方法的有效性和稳定性,搭建了一套实验装置,并采用该装置对四分之一波片样品和退偏器样品分别进行了检测。波片验证结果表明,DOP测量值与理论值高度一致,测量系统误差仅为0.0423%,证明了该方法的有效性。退偏器检测结果表明该装置的重复性水平(3σ=0.2316%)与分析预期相当,再现性水平(3σ=0.3090%)仅略差于重复性水平,具有良好的性能稳定性。
本工作为248 nm光刻机照明系统中使用的退偏器提供了一个稳定有效的离线性能检测方法,具有重要的实际意义。未来将对其进行进一步开发研究,以满足更短波长光刻系统的需求。
[1] Bruning J H. Optical lithography: thirty years and three orders of magnitude: the evolution of optical lithography tools[J]. Proceedings of SPIE, 1997, 3049: 14-27.
[2] Fay B. Advanced optical lithography development, from UV to EUV[J]. Microelectronic Engineering, 2002, 61/62: 11-24.
[3] 袁琼雁, 王向朝. 国际主流光刻机研发的最新进展[J]. 激光与光电子学进展, 2007, 44(1): 57-64.
[4] Rizvi S A. National technology roadmap for semiconductors: an analysis and perspective[J]. Proceedings of SPIE, 1998, 3331: 190-196.
[5] Zhang Y Q, Wu R M, Zheng Z R. Freeform surface off-axis illumination design with the Monge-Ampère equation method in optical lithography[J]. Applied Optics, 2014, 53(31): 7296-7303.
[6] Chen M, Zhang F, Zeng A J, et al. Method of pupil shaping for off-axis illumination in optical lithography[J]. Journal of Optical Technology, 2016, 83(3): 154-158.
[7] Oh S, Han D D, Shim H B, et al. Optical proximity correction (OPC) in near-field lithography with pixel-based field sectioning time modulation[J]. Nanotechnology, 2018, 29(4): 045301.
[8] Shen Y J, Peng F, Zhang Z R. Efficient optical proximity correction based on semi-implicit additive operator splitting[J]. Optics Express, 2019, 27(2): 1520-1528.
[9] 朱思羽, 杨宝喜, 马晓喆, 等. 光刻机环状多分区高能量利用率光瞳校正研究[J]. 中国激光, 2021, 48(17): 1704001.
[10] Flagello D G, Mulkens J, Wagner C. Optical lithography into the millennium: sensitivity to aberrations, vibration, and polarization[J]. Proceedings of SPIE, 2000, 4000: 172-183.
[11] Totzeck M, Graupner P, Heil T, et al. How to describe polarization influence on imaging[J]. Proceedings of SPIE, 2005, 5754: 23-37.
[12] 庞武斌, 岑兆丰, 李晓彤, 等. 偏振对光学系统成像质量的影响[J]. 物理学报, 2012, 61(23): 234202.
Pang W B, Cen Z F, Li X T, et al. The effect of polarization light on optical imaging system[J]. Acta Physica Sinica, 2012, 61(23): 234202.
[13] 罗红妹, 岑兆丰, 李晓彤, 等. 实际高数值孔径光刻系统的偏振分析[J]. 光学学报, 2013, 33(11): 1122002.
[14] Dejkameh A, Erdmann A, Evanschitzky P, et al. Fourier ptychography for lithography high NA systems[J]. Proceedings of SPIE, 2018, 10694: 106940B.
[15] KligerD S, LewisJ W, RandallC E. Polarized light in optics and Spectroscopy[M]. San Diego, CA, USA: Academic Press, 1990.
[16] McClain S C, Chipman R A, Hillman L W. Aberrations of a horizontal-vertical depolarizer[J]. Applied Optics, 1992, 31(13): 2326-2331.
[17] Zhang D Y, Luo F, Luo Y Q, et al. Cholesteric liquid crystal depolarizer[J]. Optical Engineering, 2007, 46(7): 070504.
[18] Bagan V A, Davydov B L, Samartsev I E. Characteristics of Cornu depolarisers made from quartz and paratellurite optically active crystals[J]. Quantum Electronics, 2009, 39(1): 73-78.
[19] 宋师霞, 宋连科. 双光楔旋光退偏器的Mueller矩阵分析[J]. 光学学报, 2009, 29(7): 1947-1950.
[20] de Sande J C, Santarsiero M, Piquero G, et al. Longitudinal polarization periodicity of unpolarized light passing through a double wedge depolarizer[J]. Optics Express, 2012, 20(25): 27348-27360.
[21] 刘晓林, 李明, 安宁, 等. 矩形光瞳下双巴比涅型消偏器的设计与分析[J]. 激光与光电子学进展, 2018, 55(8): 082601.
[22] 田杰文, 薛庆生, 鲁凤芹, 等. 星载光栅色散型光谱仪消偏器的设计与分析[J]. 光学学报, 2019, 39(3): 0313001.
[23] Li M X, Li H, Zhang S Q, et al. The manufacture of depolarizer in transform system of immersion photolithography polarization state[J]. Proceedings of SPIE, 2019, 11334: 113340T.
[24] Wang B L, Hellman W. Accuracy assessment of a linear birefringence measurement system using a Soleil-Babinet compensator[J]. Review of Scientific Instruments, 2001, 72(11): 4066-4070.
[25] Katte H. Imaging measurement of stress birefringence in optical materials and components[J]. Photonik International, 2009, 1: 39-41.
[26] 毛靖华, 王咏梅, 石恩涛, 等. 星载成像光谱仪退偏器的设计及测试[J]. 光学学报, 2017, 37(4): 0423001.
[27] Kalbarczyk A, Jaroszewicz L R, Bennis N, et al. The young interferometer as an optical system for a variable depolarizer characterization[J]. Sensors, 2019, 19(14): 3037.
张灵浩, 夏克贵, 马兴华, 朱玲琳, 曾爱军, 黄惠杰, SergeyAvakaw. 激光光强波动无关的248 nm退偏器检测方法[J]. 中国激光, 2022, 49(4): 0404003. Linghao Zhang, Kegui Xia, Xinghua Ma, Linglin Zhu, Aijun Zeng, Huijie Huang, Avakaw Sergey. Detection Method for 248 nm Depolarizer Independent of Laser Intensity Fluctuation[J]. Chinese Journal of Lasers, 2022, 49(4): 0404003.