Broadband mid-IR antireflective Reuleaux-triangle-shaped hole array on germanium
Download: 989次
Monocrystalline germanium (Ge) is a preferred lens and window material for high-performance infrared (IR) imaging systems because of its high refractive index and broadband transparency (1.8–25 μm) in the IR region. Broadband antireflective (AR) performance is urgently required alongside the development of third-generation IR detectors, which feature the application of common-aperture multiband imaging systems spanning the medium-wavelength and long-wavelength IR (MWIR, 3–5 μm, and LWIR, 8–12 μm, respectively) bands. Fabricating micro-nanostructures on the surface of optical glass has proven effective in reducing Fresnel reflections and improving the problems of adhesion, thermal mismatch, and stability associated with AR coatings[1,2]. This technology was inspired by the protruding micro-nanostructures on the surface of a moth’s cornea that can reduce reflection and protect the moth from predators. To date, various versions of the moth eye-like structures have been fabricated, including the use of shapes such as pyramids, sinusoids, tips, or truncated cones. In the nanoscale regime, the shape of a micro-nanostructure is often intimately linked to its physical and chemical properties. Different shapes, dimensions, curvatures, and packing orders of the micro-nanostructure possess different AR capacities[3
The Reuleaux triangle is a geometric shape of constant width and may be applied as a useful mechanical form. In the last decade, the Reuleaux triangle has attracted attention in the fields of chemistry, biology, and electromagnetism. It has been found that Reuleaux-triangle-shaped nanocrystals or molecular structures exhibit unusual scattering-peak characteristics[10], magnetocaloric[11], electromagnetic[12], antiferromagnetic[13], and good catalytic properties[14]. Exploring the impact of the Reuleaux-triangle shape on the optical properties of micro-nanostructures is an interesting challenge. In this work, we focus on investigating the mid-IR AR performance of Reuleaux-triangle-shaped holes on Ge. Holes, rather than protruding moth eyes, were chosen because they are more resistant to high-velocity abrasion (sand, rain, hail) and more durable in harsh environments. The Reuleaux-triangle-shaped holes were designed with slanted sidewalls to gradually match the refractive index of air to that of the Ge material. Figure
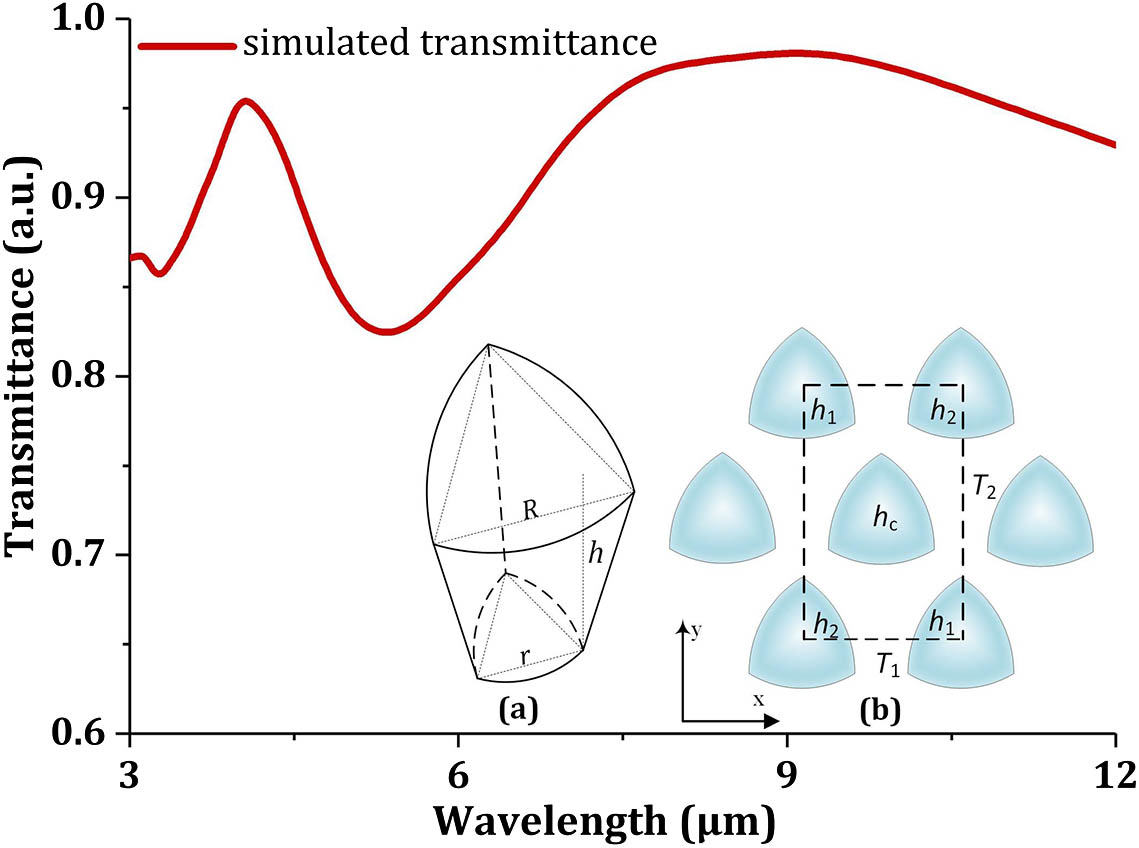
Fig. 1. Optimal simulated transmittance (red line) of the Reuleaux-triangle-shaped hole array on Ge for , , and . Inset (a) is a single Reuleaux-triangle-shaped hole, and (b) shows Reuleaux-triangle-shaped holes forming a closely packed hexagonal array (top view). The polarization direction of the incident light is 0° with the x axis.
The Reuleaux-triangle-shaped holes are closely packed into a hexagonal array, as shown in Fig.
Rigorous vector diffraction theory was used to simulate the transmittance of the Reuleaux-triangle-shaped hole array on one side of the Ge substrate, and the other side is ideal non-reflection. In general, the value of
To explore the optical properties of the Reuleaux-triangle-shaped holes, we simulated the electric field distribution for a single period of the hole array [the rectangular region with the dashed outline in Fig.
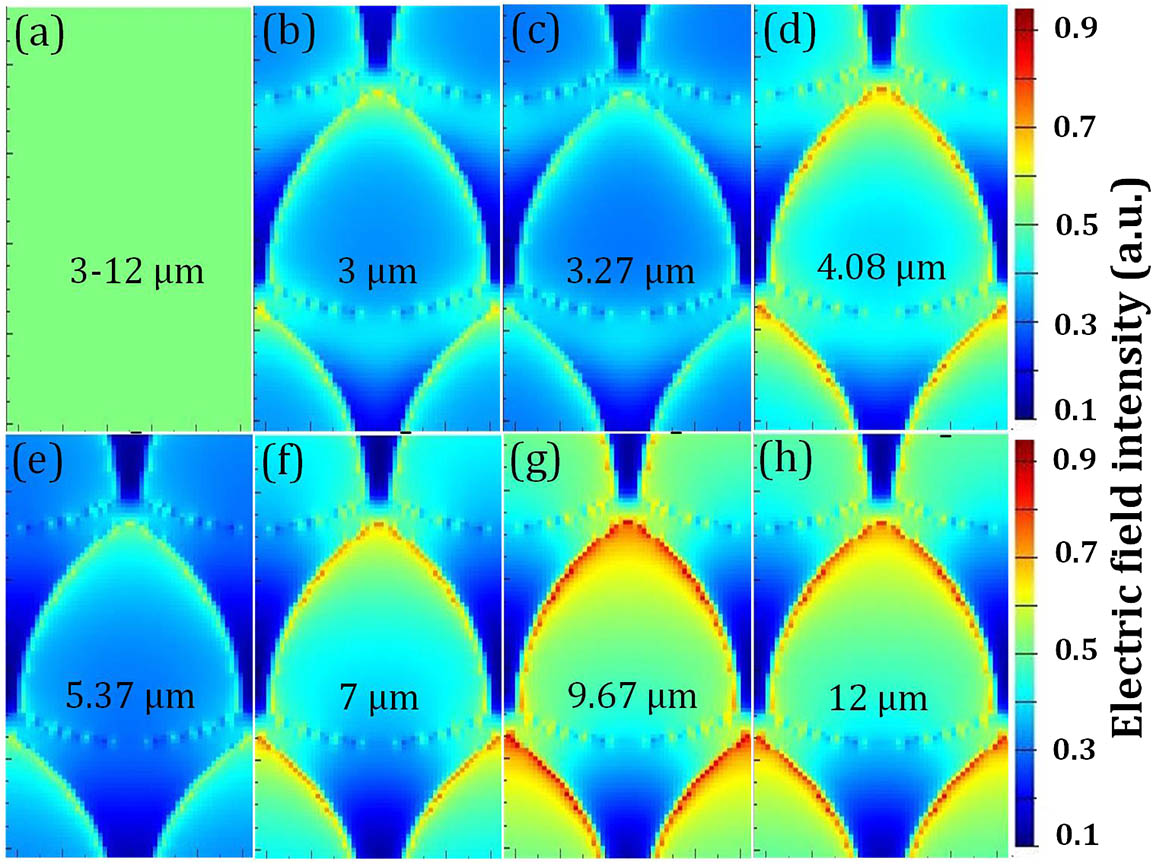
Fig. 2. Electric field distributions for a single period of the Reuleaux-triangle-shaped hole array: (a) incident 3–12 μm electromagnetic wave with uniform distribution of field intensity, and (b)–(h) different mid-IR wavelengths passing through the Reuleaux-triangle-shaped holes. The incident light is polarized.
When fabricating the Reuleaux-triangle-shaped hole array on Ge, large-area replication, sufficient hole depth, and the shape of Reuleaux triangle were the principal considerations. Nanoimprint lithography (NIL) and inductively coupled plasma (ICP) etching were selected for the fabrication in this work. NIL is an effective large-area nano-pattern replication technology that also possesses the advantages of ultra-high resolution, high output, and low cost. ICP technology can achieve sufficient depth and steepness with appropriate proportions of etchant, passivator, and physical bombardment gas. In addition, inspired by the fact that a disorderly distribution of triangles will form on the {111} planes of cubic crystals (Si, Ge) by chemical etching[16] and induction annealing[17], we attempted to fabricate an orderly Reuleaux-triangle-shaped hole array on {111} Ge with a simple circular mold.
A double-side-polished, N-type Ge wafer (
The rate of etching is mainly determined by the chemical reaction between
B–Cl adheres to the Ge surface to prevent further reaction between
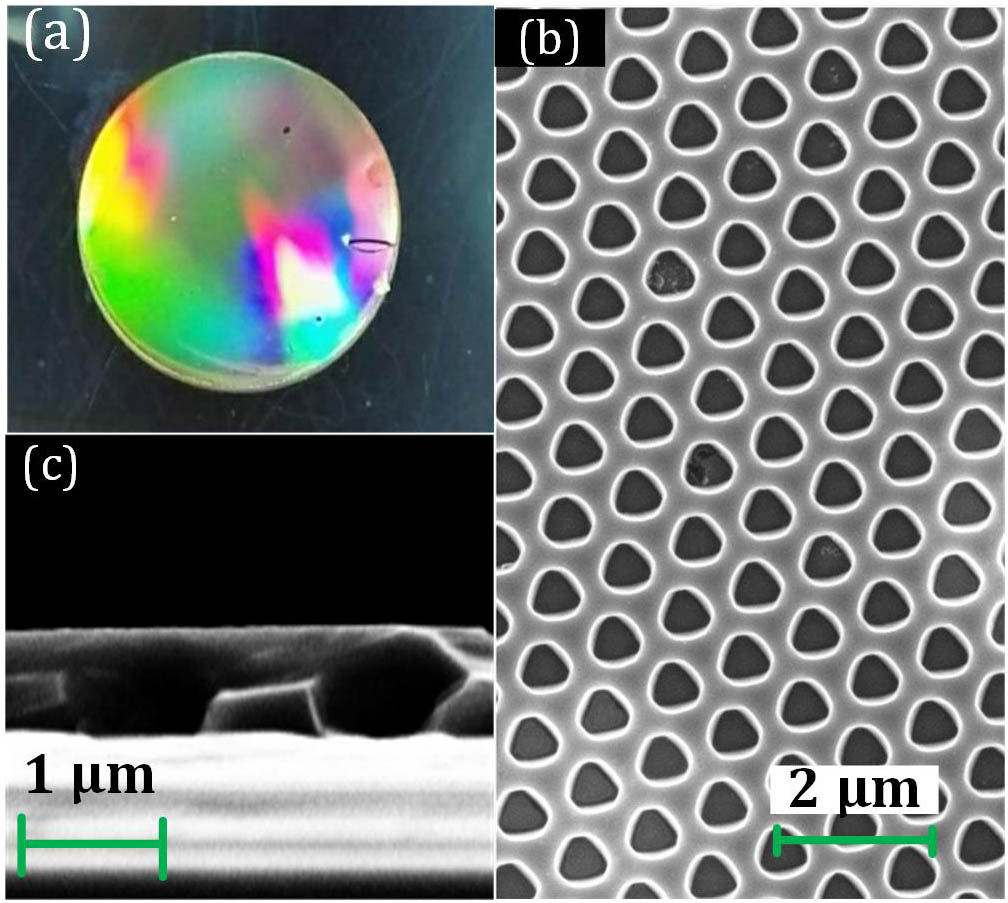
Fig. 3. (a) Photograph and (b) SEM micrograph of the fabricated Ge wafer with a Reuleaux-triangle hole array. (c) SEM side view of two Reuleaux-triangle holes.
Figure
Transmittance of the fabricated element at the normal incidence angle was measured using a double-beam IR spectrometer (WGH-30, Tianjin Gangdong Sci. & Tech. Development Co., Ltd.). Figure
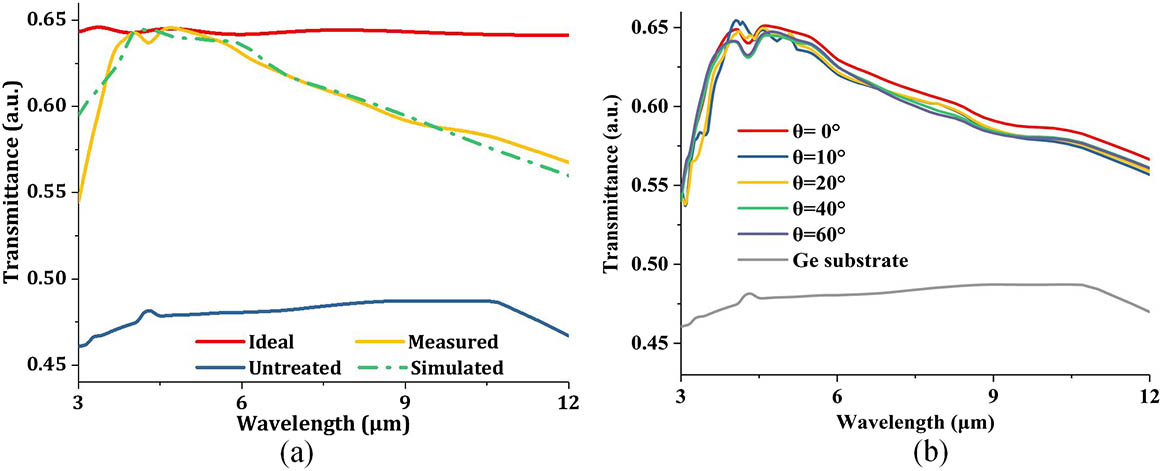
Fig. 4. (a) Transmission spectra of the fabricated element (yellow line), Ge substrate (2 mm thickness, blue line), updated simulated element with nonuniform hole depths (green dot-dashed line), and the maximum theoretical antireflection on one side (red line). (b) The measured transmittance versus incident angle.
In addition, we noticed that the measured transmission curve in Fig.
In summary, we successfully fabricated a large-area gradient-index Reuleaux-triangle-shaped hole array on the Ge {111} plane by NIL and ICP etching to realize a broadband mid-IR AR surface. The average transmittance increases 15.67% over the waveband at 3–12 μm and maintains stability at the incidence angle of up to 60°. At the vertices of the Reuleaux triangle, the electric field intensities are enhanced due to interference of the incident and reflected radiation. This local enhancement characteristic is probably also useful for the development of mid-IR radiation-trapping micro-nanostructures for IR detectors. We were also able to establish that nonuniform hole depths can modulate the shape of the transmittance curve. In the future, we will further investigate the effect of nonuniform hole depths or nonuniform diameters on the modulation of the properties of IR optical materials.
[1]
[2]
[3]
[4]
[5]
[6]
[7]
[8]
[9]
[10]
[11]
[12]
[13]
[14]
[15]
[16]
[17]
[18]
[19]
Haijuan Cheng, Miao Dong, Qinwen Tan, Linghai Meng, Yi Cai, Jie Jiang, Weisheng Yang, Haizheng Zhong, Lingxue Wang. Broadband mid-IR antireflective Reuleaux-triangle-shaped hole array on germanium[J]. Chinese Optics Letters, 2019, 17(12): 122401.