2014, 2(1) Column
High Power Laser Science and Engineering 第2卷 第1期
Large aperture N31 neodymium phosphate laser glass for use in a high power laser facility
Download:1316次

Large aperture Nd:phosphate laser glass is a key optical element for an inertial confinement fusion (ICF) facility. N31, one type of neodymium doped phosphate glasses, was developed for high peak power laser facility applications in China. The composition and main properties of N31 glass are given, together with those of LHG-8, LG-770, and KGSS- 0180 Nd:phosphate laser glasses, from Hoya and Schott, and from Russia. The technologies of pot melting, continuous melting, and edge cladding of large size N31 phosphate laser glass are briefly described. The small signal gain profiles of N31 glass slabs from both pot melting and continuous melting at various values of the pumping energy of the xenon lamp are presented. N31 glass is characterized by a stimulated emission cross section of 3:8 10??20 cm2 at 1053 nm, an absorption coefficient of 0.10–0.15% cm??1 at laser wavelength, small residual stress around the interface between the cladding glass and the laser glass, optical homogeneity of 2 10??6 in a 400 mm aperture, and laser damage threshold larger than 42 J/cm2 for a 3 ns pulse width at 1064 nm wavelength.
neodymium phosphate laser glass large aperture glass ICF facility Potassium dihydrogen phosphate (KDP) single crystals are the only nonlinear crystals currently used for electro-optic switches and frequency converters in inertial confinement fusion research, due to their large dimension and exclusive physical properties. Based on the traditional solution-growth process, large bulk KDP crystals, usually with sizes up to 600×600 mm2 so as to make a frequency doubler for the facility requirement loading highly flux of power laser, can be grown in standard Holden-type crystallizers, without spontaneous nucleation and visible defects, one to two orders of magnitude faster than by conventional methods. Pure water and KDP raw material with a few ion impurities such as Fe, Cr, and Al (less than 0.1 ppm) were used. The rapid-growth method includes extreme conditions such as temperature range from 60 to 35 ℃, overcooling up to 5C, growth rates exceeding 10 mm/day, and crystal size up to 600 mm. The optical parameters of KDP crystals were determined. The optical properties of crystals determined indicate that they are of favorable quality for application in the facility.
large-scale KDP crystals rapid growth optical quality A high power narrow-linewidth nanosecond all-fiber laser based on the master oscillator power amplifier (MOPA) configuration is demonstrated. A pulsed seed with high repetition rate of 10 MHz was generated by modulating a continuous-wave (CW) single-frequency fiber laser at 1064 nm by using an electro-optic intensity modulator (EOIM). After multi-stage cascaded power amplification, the average power was boosted to be kilowatt level. The pulses from the main amplifier had a pulse width of 3 ns and an average/peak power of 913 W/28.6 kW. Further power scaling of the pulses was limited by stimulated Raman scattering (SRS) for the moment, method for SRS suppression and further power scaling was briefly discussed.
fiber laser nanosecond pulsed laser narrow linewidth stimulated Raman scattering An ion beam has the unique feature of being able to deposit its main energy inside a human body to kill cancer cells or inside material. However, conventional ion accelerators tend to be huge in size and cost. In this paper, a future intenselaser ion accelerator is discussed to make the laser-based ion accelerator compact and controllable. The issues in the laser ion accelerator include the energy efficiency from the laser to the ions, the ion beam collimation, the ion energy spectrum control, the ion beam bunching, and the ion particle energy control. In the study, each component is designed to control the ion beam quality by particle simulations. The energy efficiency from the laser to ions is improved by using a solid target with a fine sub-wavelength structure or a near-critical-density gas plasma. The ion beam collimation is performed by holes behind the solid target or a multi-layered solid target. The control of the ion energy spectrum and the ion particle energy, and the ion beam bunching are successfully realized by a multi-stage laser–target interaction.
Intense short-pulse laser laser ion acceleration laser ion cancer therapy laser–plasma interaction Effects of target pre-heating and expansion on terahertz radiation production from intense laser-solid interactions
Download:663次

The first experimental measurements of intense (-7×1019 W cm-2) laser-driven terahertz (THz) radiation from a solid target which is preheated by an intense pulse of laser-accelerated protons is reported. The total energy of the THz radiation is found to decrease by approximately a factor of 2 compared to a cold target reference. This is attributed to an increase in the scale length of the preformed plasma, driven by proton heating, at the front surface of the target, where the THz radiation is generated. The results show the importance of controlling the preplasma scale length for THz production.
target heating terahertz radiation We review the present status and future prospects of fast ignition (FI) research of the theoretical group at the IAPCM (Institute of Applied Physics and Computational Mathematics, Beijing) as a part of the inertial confinement fusion project. Since the approval of the FI project at the IAPCM, we have devoted our efforts to improving the integrated codes for FI and designing advanced targets together with the experimental group. Recent FI experiments [K. U. Akli et al., Phys. Rev. E 86, 065402 (2012)] showed that the petawatt laser beam energy was not efficiently converted into the compressed core because of the beam divergence of relativistic electron beams. The coupling efficiency can be improved in three ways: (1) using a cone–wire-in-shell advanced target to enhance the transport efficiency, (2) using external magnetic fields to collimate fast electrons, and (3) reducing the prepulse level of the petawatt laser beam. The integrated codes for FI, named ICFI, including a radiation hydrodynamic code, a particle-in-cell (PIC) simulation code, and a hybrid fluid–PIC code, have been developed to design this advanced target at the IAPCM. The Shenguang-II upgraded laser facility has been constructed for FI research; it consists of eight beams (in total 24 kJ=3!, 3 ns) for implosion compression, and a heating laser beam (0.5–1 kJ, 3–5 ps) for generating the relativistic electron beam. A fully integrated FI experiment is scheduled for the 2014 project.
fast ignition integrated simulation codes It is demonstrated by simulations and analysis that a wakefield driven by an ultrashort intense laser pulse in underdense plasma can emit tunable electromagnetic radiation along the laser propagation direction. The profile of such a kind of radiation is closely associated with the structure of the laser wakefield. In general, electromagnetic radiation in the terahertz range with its frequency a few times the electron plasma frequency can be generated in the moderate intensity regime. In the highly nonlinear case, a chain of radiation pulses is formed corresponding to the nonlinear structure of the wake. Study shows that the radiation is associated with the self-modulation process of the laser pulse in the wakefield and resulting transverse electron momenta from modulated asymmetric laser fields.
laser wakefield PIC simulation tunable radiation transverse momentum 动态信息
动态信息 丨 2023-06-21
HPL Highlight (Vol. 11, e40): NIF仍然是全球唯一一个能够进行ICF点火实验的装置动态信息 丨 2023-06-21
HPL Highlight (Vol. 11, e9): 变分神经网络预测激光尾波场加速电子能谱动态信息 丨 2023-06-21
HPL Highlight (Vol. 11, e7): 目标探测网络加入,激光等离子体加速器实现实时诊断动态信息 丨 2023-06-21
HPL Highlight (Vol. 11, e5): 910 nm波段超宽带种子源,向100 PW超强超短激光进发动态信息 丨 2023-06-21
HPL Highlight (Vol. 11, e4): 100 PW单束压缩!多步压缩器进阶双光栅激光评论微信公众号
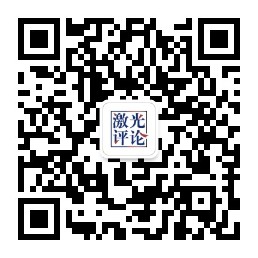
点击菜单“联系编辑”即可添加期刊编辑为好友啦