True random coded photon counting Lidar
1 Introduction
Photon counting Lidar has become more and more popular in the ranging and three-dimensional imaging area due to its capability of extremely weak signal detection1-4. But it's a statistical sampling technique and needs to accumulate enough photons to establish statistical histograms. However, the multi-period cumulative process greatly slows down the measuring speed and it is not appropriate for scenarios that require quick measurements. In order to solve this problem, researchers have proposed the pseudo-random coded photon counting Lidars.
The combination of pseudo-random coded method and photon counting ranging technique is called the pseudo-random coded photon counting ranging Lidar. The pseudo-random coded photon counting ranging Lidar has been favored by more and more researchers due to its advantages of low transmitting power, overcoming range ambiguity and fast detection. In 1983, Takeuchi et al. took a random phase code modulate continuous-wave laser with an external optical modulator, as a laser source in a laser ranging system to improve the signal-to-noise ratio5. Sun6 applied pseudo-random coded method to photon counting laser altimeter for long-range targets ranging. Hiskett7, Krichel8 and Ullrich9 used a finite non-periodic pulse train or pulse-position modulation technique to resolve range ambiguity. Zhang realized the detection of a non-cooperative target in 1.2 km using the pseudo-random coded photon counting Lidar ranging technique10. Yang realized high spatial resolution detection using a high speed pseudo-random modulation photon counting fiber laser ranging system11. In addition, it can effectively improve the measurement speed of the system compared with the pulse accumulation photon counting Lidar12-13.
However, the pseudo-random coded photon counting Lidar also has some shortcomings. Firstly, this method usually uses mature M-sequence or other random sequence. In both cases, the interval between two adjacent '1' bits is less than the single photon detector's dead time. When the single photon detector responds to a photon, it will enter the dead period and cannot respond to any other arriving photons during it. Thus, if the pulse interval of two adjacent '1' bits is less than the single photon detector's dead time, the front '1' bit will make the single photon detector dead, and the latter '1' bit cannot be responded even the signal photons arrived. Therefore, the ranging performance is degraded. To overcome this issue, we propose the true random coded method. It's well known that the noise pulse of a single photon detector is completely random, thus we take advantage of this character, and use the noise pulse as the signal source to drive the laser and generate a true random laser pulse sequence for the Lidar detection. The true random coded method has two advantages over the pseudo-random coded method.
Firstly, a single photon detector will not generate any pulse during its dead time, which means that the output pulse interval between any two adjacent pulses is larger than its dead time. Therefore, we can consider that any two '1' bits in the true random sequence are independent of each other. In other words, they do not have a negative effect on each other. In this case, the dead time effect can be completely avoided.
Secondly, due to its physical randomness, the probability of that two true random sequences are identical is extremely low, thus it has excellent anti-crosstalk performance. Therefore, the true random coded photon counting Lidar has obvious advantages in the scenario where multiple Lidars are working simultaneously, such as vehicle automatic driving14.
In addition, it is worth mentioning that for the single photon detector used to generate random pulses, we don't need it has high performance as the one used for return signal detecting, because it can be much cheaper than a function generator. Furthermore, one can even think about utilizing the noise from the receiving detector itself (Gm-APD 2 in
2 System description
2.1 System structure and ranging principle
As shown in
where N is the number of '1' bit in the true random sequence, R is the target distance, and c is the speed of light. When τ corresponds to the time of flight, the cross-correlation function g(τ) has the maximum value.
2.2 The auto-correlation of the true random coded photon counting Lidar
In order to prove the feasibility of the true random coded photon counting ranging Lidar, we first need to verify the auto-correlation of true random sequences. This is the premise that the true random coded photon counting Lidar system can be used for ranging. The number of '1' bits per unit time is called the mean pulse count density. The normalized auto-correlation coefficient of the random sequence can be written as
where a(t) is the true random sequence and T is the period of the true random sequence (the length of the true random sequence).
We record a 200 μs length true random sequence and complete auto-correlation operation. As shown in
In this section, the feasibility of the true random coded photon counting Lidar was proved. And in the following section, we mainly show the ranging performance improvement of the true random coded photon counting Lidar compared with the traditional pseudo-random coded photon counting Lidar.
3 Experiment
3.1 Ranging performance comparison
We built an experimental platform, with which the ranging performances of the true random coded method and the pseudo-random coded method are compared. In the true random coded method, the density of '1' bit is 1 Mcps, and the length of the true random sequence is 200 μs. In other words, about 200 laser pulses are emitted in a true random sequence. For the pseudo-random coded method, we choose the commonly used M sequence. To generate a common M sequence with the same length (200 μs) and the same pulse width (4 ns), the degree of the M sequence will be 14. The main parameters of the two Lidar systems are given in
Table 1. Main parameters of the Lidar system.
|
When the noise count is 1 Mcps, we compare the auto-correlation function of the true random coded method and the pseudo-random coded method under different echo signal intensities. As shown in

Fig. 4. Normalized auto-correlation range images with two different echo photons number for the true random coded method and the pseudo-random coded method.
For the same echo signal intensity, the side-lobe of the true random coded method is lower than that of the pseudo-random coded method, which means that the ranging performance of the true random coded method is better than that of the pseudo-random coded method. Further, the auto-correlation function's side-lobes of the two methods decrease with the enhancement of echo signal intensity, but the true random coded method has obvious advantages over the pseudo-random coded method in suppressing side-lobes.
The comparisons above are between true random sequences and common M sequences with the same length and the same pulse width. When the M sequence is modified sparsely enough that with the same pulse numbers as the true random sequence by adding '0' codes, it seems the same as the true random sequence if we only look at just one period. However, the sparse pseudo-random sequence is repeated periodically (as shown in
Meanwhile, the probability that the system can correctly identify targets is also an important index to evaluate the ranging performance. When the noise counts are 1 Mcps and the mean echo photons number per '1' bit is 1-5, 300 experiments are completed with different echo signal intensity for the two methods, respectively. Under different echo signal intensities, the probabilities of the two methods correctly measuring the target distance are shown in

Fig. 5. The detection probability statistical results of the true random coded method and the pseudo-random coded method at different mean echo photons number.
When the echo signal intensity is weak, the detection probability of both methods is relatively low. And the difference of detection probabilities between the two methods is not obvious. This is because when the number of echo photons is small, the detection probability of the '1' bit in the two methods is low. There are many '1' bits in the true random sequence and the pseudo-random sequence that cannot be detected, which makes the correlation of both methods poor. Meanwhile, when the number of echo photons is small, the influence of dead time on the pseudo-random coded method is not obvious. Thus, the detection probability of both methods is relatively low, but there is no obvious difference.
With the increase of echo signal intensity, the detection probability of '1' bit increases. The true random coded method can effectively avoid the influence of detector dead time. Thus, the detection probability increases rapidly, and finally approaches 100%. However, with the increase of echo signal intensity, the influence of dead time on the pseudo-random coded method is more obvious. That is to say, there are more '1' bits in the pseudo-random coded method which cannot be detected because the detector enters the dead period. Therefore, the detection probability of the pseudo-random coded increases slowly, and is significantly lower than that of the true random coded method.
3.2 Anti-crosstalk performance comparison
To evaluate the anti-crosstalk performance of the coding, we use the cross-correlation function. The normalized cross-correlation can be written as:
where a1 and a2 are two different sequences and T is the period of them (the length of the sequences). We use the same true random sequence as above. For the M sequence, it is modified by adding '0' codes to make it sparse enough as same as the true random one (200 pulses in 200 μs).
The normalized cross-correlation for the true random sequence (a) and the sparse pseudo-random sequence (b) are shown in
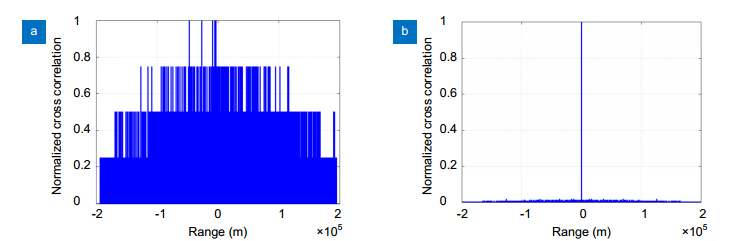
Fig. 6. Normalized cross-correlation range images for the true random sequence (a) and the sparse pseudo-random sequence (b).
3.3 Scanning imaging of the true random coded method
From
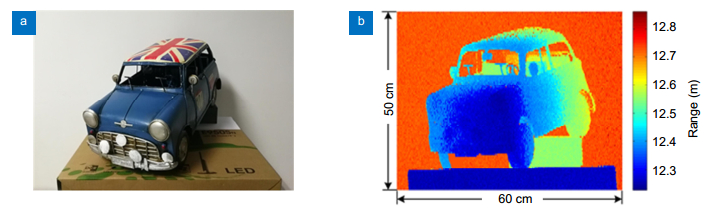
Fig. 7. (a) The picture of the model car and (b) three-dimensional scanning imaging of the model car.
Table 2. Key parameters for the depth profile measurement.
|
4 Conclusion
The true random coded photon counting Lidar system retains the advantages of traditional pseudo random coded photon counting Lidar system, such as low transmitting power, fast detection and overcoming range ambiguity. It uses a single photon detector as a random sequence generator. Single photon detector generates random sequence, which belongs to true random sequence and has very high anti-crosstalk ability. More importantly, it can effectively avoid the influence of detector's dead time and improve the detection performance of the system. A simple experimental platform is built to complete the principle verification. Experiments verify that the ranging performance of the true random coded method is obviously better than that of the traditional pseudo-random coded method. Finally, we use the true random coded photon counting Lidar to complete a three-dimensional scanning imaging of a model car.
5 Acknowledgements
This work was supported by the National Natural Science Foundation of China (NSFC) (61805249), and the Youth Innovation Promotion Association CAS (2019369).
6 Competing interests
The authors declare no competing financial interests.
Article Outline
Bo Liu, Yang Yu, Zhen Chen, Weiqiang Han. True random coded photon counting Lidar[J]. Opto-Electronic Advances, 2020, 3(2): 02190044.