基于20/400 μm增益光纤的3 kW近单模全光纤放大器及其长时工作特性
下载: 1146次
1 引言
光纤激光器具有转换效率高、输出光束质量好、结构紧凑等优点,在航空航天、工业加工、生物医疗等领域得到了广泛的应用[1-4]。随着应用领域的拓展及深入,人们对高功率光纤激光器提出了更高的要求,比如更高的功率、长时间稳定运行以及近衍射极限的光束质量。近年来,受激拉曼散射(SRS)、抽运源亮度、热透镜效应等光纤中的非线性效应一直是限制高功率光纤激光器发展的重要因素[5-8]。2010年,Eidam等[9]发现了横向模式不稳定效应(TMI),该效应是限制高功率单模近衍射极限光纤激光功率提升的主要原因。当激光器的功率达到TMI阈值后,输出激光的光束质量急剧退化[10-12],同时输出功率也出现明显波动,功率稳定性下降。而由于光纤盘绕等原因,TMI产生的高阶模激光将泄漏到包层中,从而导致输出功率滞涨,甚至出现功率下降的现象[13-16]。因此,TMI不仅限制了激光器功率的提升,还使激光器处于不安全的工作状态。
为了抑制TMI效应的产生,国内外学者在理论和实验方面都进行了广泛研究。德国耶拿大学、美国克莱姆森大学等机构的研究人员认为,增益光纤中的热效应引起光纤纵向周期性折射率调制,导致激光器中基模与高阶模的功率耦合,从而产生TMI效应 [17-21]。根据这一理论,人们发现可以从降低热负荷、抑制高阶模和长周期光栅等方面来提高激光器的模式不稳定阈值。具体来说,可以通过采用低吸收系数泵源[22]、改变抽运方式[23-24]、设计特殊光纤[25-27]、增益饱和效应[28-30]等方法提高TMI阈值功率。
由于SRS效应以及抽运源亮度等因素的限制,目前输出功率大于3 kW的激光器主要是基于纤芯直径为25 μm[31]和30 μm[32-33]的大模场双包层增益光纤。然而,纤芯直径大于25 μm的增益光纤内支持的模式较多,很难实现准单模激光输出。如果不对光纤结构进行精心设计,此类大模场增益光纤的多模输出特性将导致其TMI阈值普遍较低,很难实现高稳定、高可靠及高功率的准单模激光输出。在实际的工业生产应用中,则需要同时保证激光器的功率、光束质量和输出稳定性。目前,鲜有如何抑制TMI以获得长时间稳定可靠的激光输出的报道。由于大模场面积光纤结构设计具有特殊性与复杂性,而纤芯直径为20 μm的商用光纤输出近单模激光,其模式不稳定抑制相对容易,若进行批量生产,可以较好地保证其工艺一致性。因此,基于纤芯直径为20 μm的商用双包层光纤研制3 kW级高稳定、高可靠的激光器是较为明智的选择。但是,由于该光纤的纤芯直径较小,光纤中的高能量密度将导致非线性效应的产生。如何在抑制TMI的同时兼顾SRS的抑制[34],也是需要考虑的问题。
基于此,本文对纤芯/内包层直径为20/400 μm的掺镱光纤放大器进行研究,通过优化激光器的结构和参数,实现准单模3 kW激光输出。采用弯曲盘绕增益光纤的方式[33,35-36]实现TMI阈值的提升;通过优化抽运方式和抽运功率配比[23,37-38],综合抑制TMI和SRS效应,成功实现了可长时间稳定工作的3 kW近单模激光输出,输出激光SRS抑制比约为20 dB。在长时间(10 h)高功率运行过程中,时/频域上并未监测到模式不稳定效应,为实现高功率光纤激光器以及3 kW级光纤激光器的工业设计提供可靠参考。
2 实验结构
本实验系统采用全光纤非保偏主振荡功率放大结构,放大器的结构如

图 1. 双向抽运结构的全光纤放大器示意图
Fig. 1. Schematic of all-fiber amplifier with bi-directional pumping configuration
3 实验结果与分析
3.1 弯曲选模抑制TMI效果研究
基于
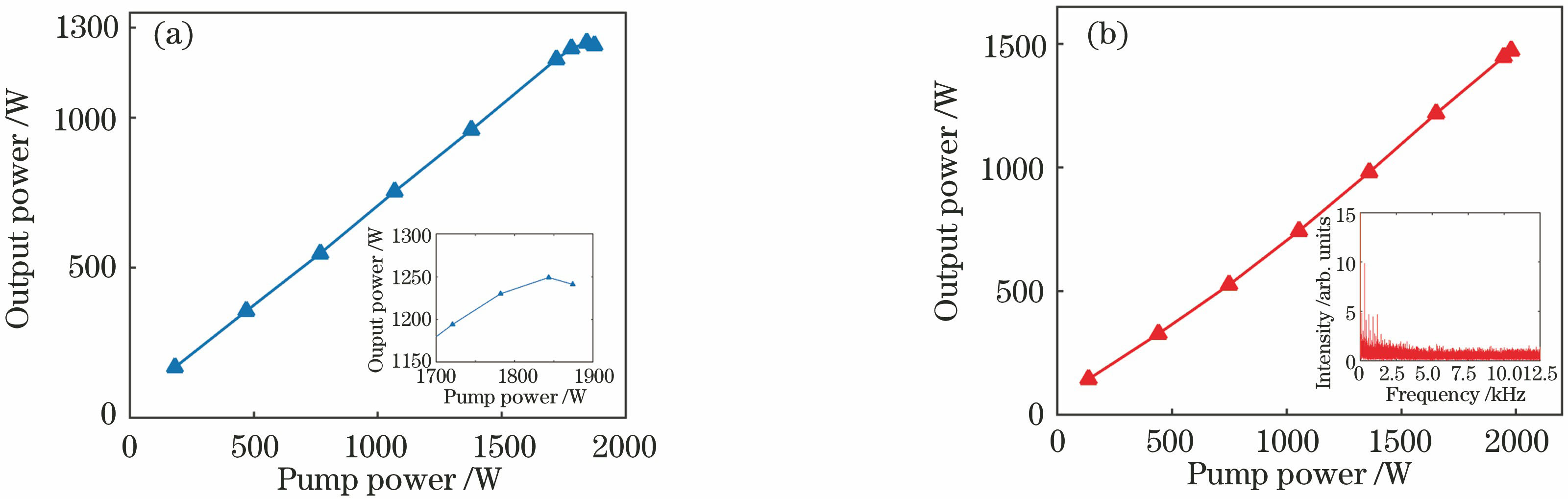
图 2. 未进行TMI抑制时单向抽运结构的全光纤放大器输出功率曲线。(a)前向抽运输出功率及功率下降处细节图;(b)后向抽运输出功率及阈值处的频域信号
Fig. 2. Output power curves of all-fiber amplifier with single-directional pumping configuration before suppression of TMI. (a) Output power for forward pumping and detailed figure when output power drops; (b) output power for backward pumping and frequency domain signal at threshold
为了提高放大器TMI的阈值,在不改变系统结构的情况下,利用光纤盘B进行光纤盘绕,将增益光纤的最小弯曲直径减小为10 cm,并再一次进行前向和后向单独抽运实验,结果如
从

图 3. TMI抑制后单向抽运结构的全光纤放大器的输出功率和输出光谱。(a)前向抽运输出功率及1390 W处光谱;(b)后向抽运输出功率及1810 W处光谱
Fig. 3. Output power and spectra of all-fiber amplifier with single-directional pumping configuration after suppression of TMI. (a) Output power and spectrum at 1390 W for forward pumping; (b) output power and spectrum at 1810 W for backward pumping
3.2 采取双端抽运和弯曲选模,综合抑制TMI和SRS
利用减小弯曲半径的方法能够很好地抑制TMI效应,而且后向抽运方式的热分布较为均匀,能够实现更高的TMI阈值以及SRS阈值。但是由于单向抽运源功率的限制,前/后向单独抽运都无法实现抽运功率为3 kW的激光输出。因此必须采取双端抽运的方式,才有可能实现3 kW激光输出。
2017年,国防科技大学的陶汝茂课题组[23]基于不同尺寸的光纤放大器,对双向抽运下不同功率配比的TMI阈值进行了理论计算,发现对20/400 μm增益光纤来说,当后向抽运光比例达到总抽运功率的75%时,可得到最高的阈值功率输出。2018年,Li等[38]在25/400 μm增益光纤级联放大器的实验中发现,后向抽运光比例为70%时可得到最高TMI阈值输出。考虑到如
对空置抽运臂激光的监测中,由于输出光方向的影响,后向激光增长幅度远大于前向激光,当输出功率达到3 kW时,两个剩余臂处监测到的功率约为70 W的后向激光输出,利用光谱仪可以发现,这主要是泄漏至包层中的高阶模信号光。
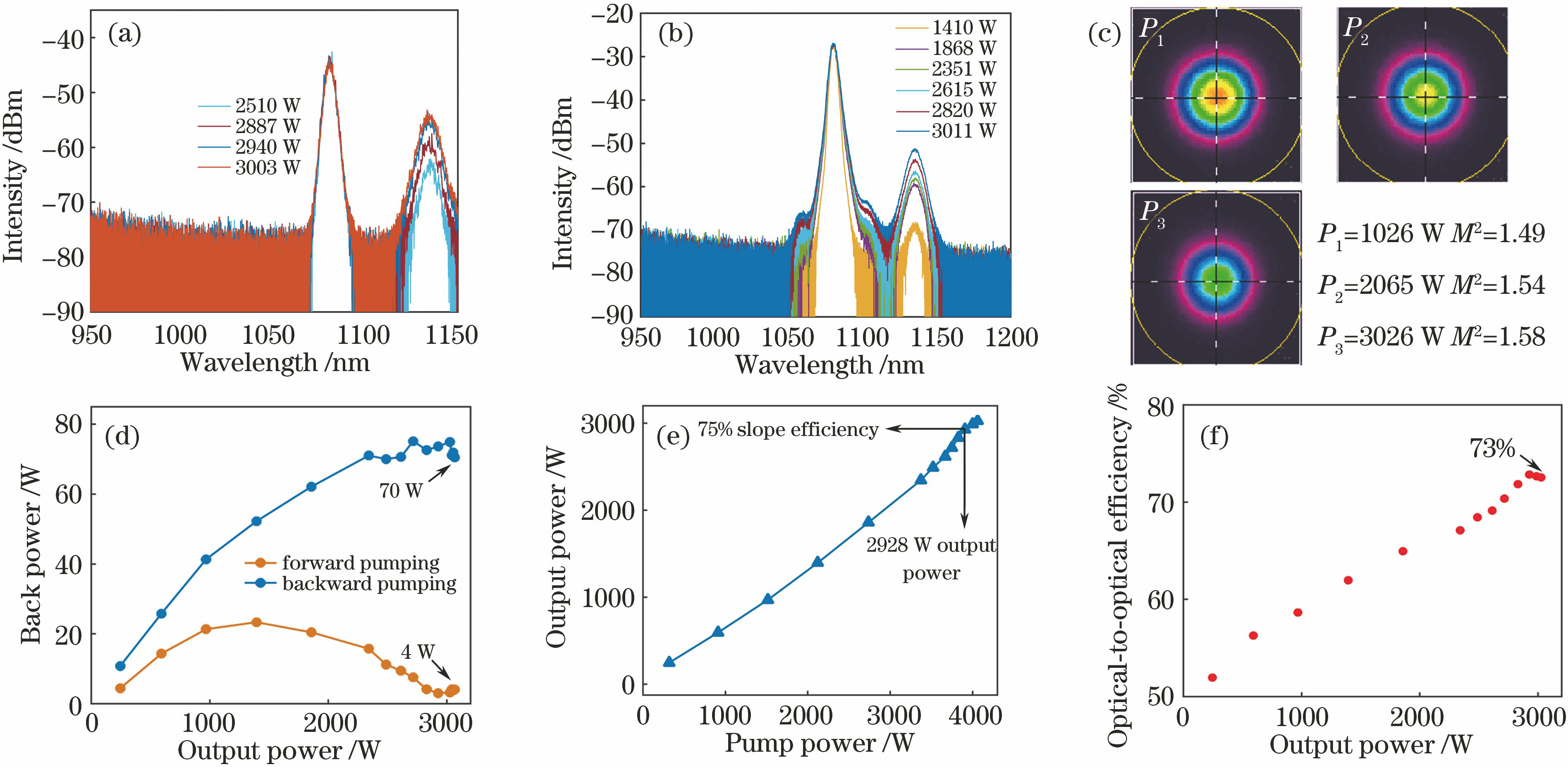
图 4. 3 kW近单模光纤输出过程。(a)优化前不同输出功率下的光谱;(b)优化后不同输出功率下的光谱;(c) 1,2,3 kW下的光斑图及光束质量;(d)空置抽运臂激光;(e)输出功率随抽运功率的变化曲线;(f)光-光转换效率
Fig. 4. Output process of 3 kW near single-mode laser. (a) Spectra before optimization under different output powers; (b) spectra after optimization under different output powers; (c) beam spots and beam qualities at 1, 2, 3 kW; (d) output power of idle pumping port; (e) output power versus pump power; (f) optical-to-optical efficiency
3.3 3 kW放大器长时工作特性研究
考虑到工业加工等应用领域对激光器长时间工作的稳定性和可靠性的要求,对所设计的3 kW放大器进行高功率连续测试实验。实验的时间为10 h,运行功率为3 kW,并对激光器的功率、光谱、光束质量和时域特性进行不间断监测。
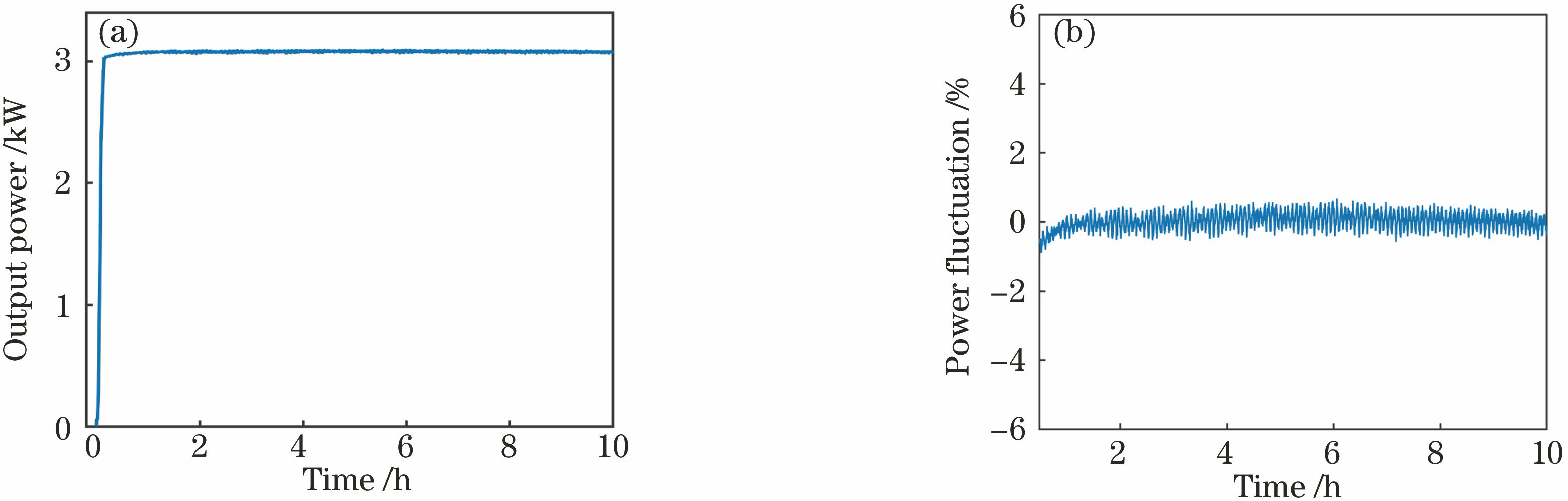
图 5. 放大器连续工作10 h的测试结果。(a)输出功率曲线;(b)功率波动曲线
Fig. 5. Test results of amplifier for 10 h of operation. (a) Output power curve; (b) power fluctuation curve
实验过程中每间隔20 min对光谱、

图 6. 连续测试的10 h内不同时刻的输出结果。(a) 光谱;(b)光束质量;(c) 时域分布;(d) 频域分布
Fig. 6. Output results at different moments in 10 h of continuous test. (a) Spectra; (b) beam qualities;(c) time domain distributions; (d) frequency domain distributions
4 结论
对3 kW近单模全光纤放大器的实现及其稳定长时输出进行实验研究。结果表明,采用减小增益光纤弯曲半径的方式能够滤除光纤中的高阶模式,对提高放大器TMI阈值具有显著作用,在前/后向单端抽运的情况下其阈值功率可分别提升11%和22%。由于减小弯曲半径的方法可滤除进入增益光纤前段的高阶模,后向抽运下放大的输出激光高阶模成分比前向抽运更少,且后向抽运对于热分布更具优势,模式不稳定抑制效果也更明显;优化前/后向抽运功率配比来综合抑制SRS与TMI,采用双端抽运方式实现了功率大于3 kW的激光输出,在SRS抑制比为24 dB时,光束质量低于1.6,近似单模输出,因此抑制SRS对光纤的热管理有一定的促进作用,并能提高TMI阈值,有利于激光器稳定工作。同时,还研究了放大器长时间工作特性,在3 kW水平进行了10 h的连续测试实验。SRS抑制比稳定在20 dB左右,光束质量可稳定保持1.7以下,输出光斑形态良好,时/频域上也未观察到模式不稳定效应特征。所设计的激光器性能稳定,有望用于工业加工等应用并实现长时间工作。
[1] 张军, 潘玉寨, 胡贵军, 等. 高功率光纤激光器的应用与展望[J]. 半导体光电, 2003, 24(4): 222-226.
张军, 潘玉寨, 胡贵军, 等. 高功率光纤激光器的应用与展望[J]. 半导体光电, 2003, 24(4): 222-226.
[2] 王天及, 李耀棠, 杨世宁, 等. 高功率光纤激光器及其应用[ C]. 全国光电技术与系统学术会议, 2003: 1198- 1202.
王天及, 李耀棠, 杨世宁, 等. 高功率光纤激光器及其应用[ C]. 全国光电技术与系统学术会议, 2003: 1198- 1202.
Wang TJ, Li YT, Yang SL, et al. High power fiber laser & its application[ C]. National Conference on Photoelectric Technology and Systems, 2003: 1198- 1202.
Wang TJ, Li YT, Yang SL, et al. High power fiber laser & its application[ C]. National Conference on Photoelectric Technology and Systems, 2003: 1198- 1202.
[4] 宋志强. 大功率光纤激光器技术及其应用[J]. 山东科学, 2008, 21(6): 72-77.
宋志强. 大功率光纤激光器技术及其应用[J]. 山东科学, 2008, 21(6): 72-77.
[6] Jauregui C, Limpert J, Tünnermann A. High-power fibre lasers[J]. Nature Photonics, 2013, 7(11): 861-867.
Jauregui C, Limpert J, Tünnermann A. High-power fibre lasers[J]. Nature Photonics, 2013, 7(11): 861-867.
[8] 史尘, 陶汝茂, 王小林, 等. 光纤激光模式不稳定的新现象与新进展[J]. 中国激光, 2017, 44(2): 0201004.
史尘, 陶汝茂, 王小林, 等. 光纤激光模式不稳定的新现象与新进展[J]. 中国激光, 2017, 44(2): 0201004.
[13] Wang XL, Tao RM, XiaoH, et al. Experimental studies of mode instability and thermal effects in all-fiber amplifier[C]. Advance Solid-State Laser Congress, 2013: JTh2A. 44.
Wang XL, Tao RM, XiaoH, et al. Experimental studies of mode instability and thermal effects in all-fiber amplifier[C]. Advance Solid-State Laser Congress, 2013: JTh2A. 44.
[15] LaurilaM, Jørgensen MM, LægsgaardJ, et al. Highly efficient 90 μm core rod fiber amplifier delivering >300 W without beam instabilities[C]. Conference on Lasers and Electro-Optics-International Quantum Electronics Conference, 2013: 1.
LaurilaM, Jørgensen MM, LægsgaardJ, et al. Highly efficient 90 μm core rod fiber amplifier delivering >300 W without beam instabilities[C]. Conference on Lasers and Electro-Optics-International Quantum Electronics Conference, 2013: 1.
[16] Puju PV, Zelenova MV, Tyrtyshnyy VA. Mode instability observation in fiber amplifier of single-frequency radiation at 1560 nm wavelength[C]. International Conference Laser Optics, 2016: S1- S15.
Puju PV, Zelenova MV, Tyrtyshnyy VA. Mode instability observation in fiber amplifier of single-frequency radiation at 1560 nm wavelength[C]. International Conference Laser Optics, 2016: S1- S15.
[22] Tao R M, Ma P F, Wang X L, et al. Mitigating of modal instabilities in linearly-polarized fiber amplifiers by shifting pump wavelength[J]. Journal of Optics, 2015, 17(4): 045504.
Tao R M, Ma P F, Wang X L, et al. Mitigating of modal instabilities in linearly-polarized fiber amplifiers by shifting pump wavelength[J]. Journal of Optics, 2015, 17(4): 045504.
[34] 王小林, 陶汝茂, 杨保来, 等. 掺镱全光纤激光振荡器横向模式不稳定与受激拉曼散射的关系[J]. 中国激光, 2018, 45(8): 0801008.
王小林, 陶汝茂, 杨保来, 等. 掺镱全光纤激光振荡器横向模式不稳定与受激拉曼散射的关系[J]. 中国激光, 2018, 45(8): 0801008.
罗雪雪, 奚小明, 史尘, 王小林, 张汉伟, 陶汝茂, 韩凯, 周朴, 许晓军. 基于20/400 μm增益光纤的3 kW近单模全光纤放大器及其长时工作特性[J]. 中国激光, 2019, 46(2): 0201004. Xuexue Luo, Xiaoming Xi, Chen Shi, Xiaolin Wang, Hanwei Zhang, Rumao Tao, Kai Han, Pu Zhou, Xiaojun Xu. 3 kW Near-Single-Mode All-Fiber Amplifier Based on 20/400 μm Gain Fiber and Its Long Time Operation Characteristics[J]. Chinese Journal of Lasers, 2019, 46(2): 0201004.